Understanding Biocompatibility in Biomedical Research
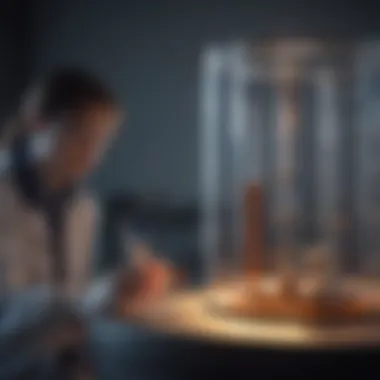
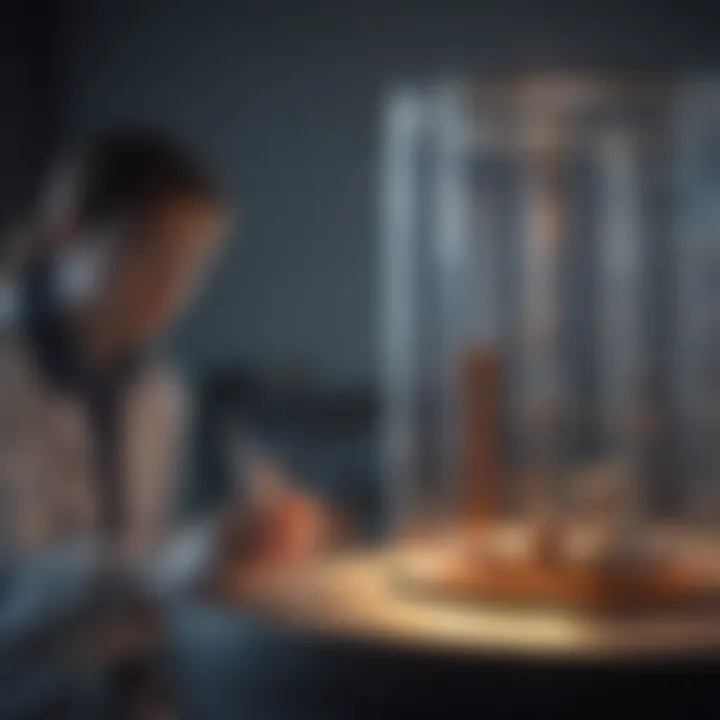
Intro
Biocompatibility is often regarded as the backbone of biomedical materials and devices. This fundamental concept has implications directly impacting patient safety and the effectiveness of medical procedures. After all, when we think about connecting a foreign material to the human body, one cannot help but wonder: will it integrate smoothly, or will it incite a chaotic response? Understanding biocompatibility is not merely an academic exercise; it governs how innovations in medical technology can translate into real-world applications that save lives.
In the complex web of biomedical research, assessing biocompatibility involves a multitude of methodologies and standards. From the intricate designs of in vitro experiments to the more holistic in vivo studies, each method plays a crucial role in determining whether a material can sit comfortably alongside human physiology. This article aims to break down the intricacies involved in evaluating biocompatibility, highlighting everything from traditional practices to the latest innovations reshaping the field.
Overview of Research Topic
Brief Background and Context
The term biocompatibility refers to how well a material interacts with biological systems without causing adverse reactions. In simpler terms, it’s often a question of compatibility between man-made materials and the human body. This evaluation has gained prominence due to the growing reliance on materials for implants, prosthetics, and drug delivery systems.
The history of biocompatibility dates back several decades. Initially, the focus was primarily on identifying toxicity through basic cytotoxicity tests, but as technology advanced and our understanding of biological interactions improved, the field has expanded significantly. We’ve moved from rudimentary assessments to a comprehensive framework involving myriad tests tailored to specific applications. Not only does this improve patient outcomes, but it also fortifies the trust in emerging biomedical technologies.
Importance in Current Scientific Landscape
Today, biocompatibility is central to both innovation and regulation within the biomedical landscape. The ongoing advancements in materials science, biotechnology, and medical engineering necessitate rigorous evaluations to ensure safety and effectiveness. As the demand for patient-specific solutions grows, the onus on accurate biocompatibility assessment intensifies.
Moreover, regulatory authorities such as the FDA and ISO have set stringent guidelines delineating the pathways for biocompatibility testing. Navigating these regulations is critical for researchers and manufacturers alike, as non-compliance could spell disaster for any new product aimed at the medical market.
"Understanding biocompatibility is crucial, not only for the health of individual patients but also for the very foundation of trust in modern healthcare solutions."
Methodology
Research Design and Approach
To dive deep into biocompatibility evaluations, one must employ a systematic approach that balances quantitative and qualitative methods. The research design typically involves a combination of literature review, experimental analysis, and regulatory framework evaluation. A layered methodology ensures that all angles are explored, offering a holistic understanding of what makes materials biocompatible.
In some cases, the approach might involve the establishment of a multi-phase study.
- Phase 1: A comprehensive literature review identifying existing biocompatibility standards and testing methodologies.
- Phase 2: Experimental design involving both in vitro and in vivo assessments of selected materials for their biological responses.
- Phase 3: Critical analysis comparing results to existing benchmarks set forth by regulatory bodies.
Data Collection Techniques
Data collection in this realm can take many forms. For in vitro studies, techniques such as:
- Cell Viability Assays
- Cytokine Release Assays
- Histological Evaluations
are commonly used to gauge biological interactions. In contrast, in vivo tests may include implantation studies, where materials are evaluated for tissue response over time. These methods not only yield quantitative data but also provide qualitative insights into material interactions, thus steering the direction of further research.
In summary, encompassing both traditional and emerging strategies fosters an environment where knowledge grows exponentially. This leads to an enriched understanding not just for researchers but also for educators and practitioners who are eager to remain at the cutting edge of healthcare solutions.
Defining Biocompatibility
When it comes to biomedical research, the concept of biocompatibility holds a pivotal position. It encapsulates how materials interact with biological systems, shedding light on their safety and effectiveness for medical use. Understanding biocompatibility is not just a technical requirement—it’s a fundamental necessity in ensuring that new medical devices, implants, and materials do not provoke harmful reactions in the body. The meticulous evaluation of biocompatibility directly impacts patient safety, paving the way for successful medical interventions.
It's crucial to recognize that biocompatibility is multidimensional, ranging from chemical composition to biological response. For instance, a new polymer used in drug delivery must not only effectively release the medication but also be non-toxic, non-carcinogenic, and compatible with the surrounding tissues. This complexity underscores the importance of a structured approach to biocompatibility evaluations; overlooking any aspect can lead to catastrophic outcomes in clinical settings.
The Importance of Biocompatibility in Medicine
The significance of biocompatibility in medicine can't be overstated. Firstly, from the standpoint of regulatory adherence, the evaluation of biocompatibility is often a prerequisite for approval from regulatory bodies like the FDA or the ISO. Their guidelines necessitate rigorous testing to ensure that any new material is safe for patient use. For example, when a new stent is manufactured, its biocompatibility must be thoroughly tested to prevent thrombosis, inflammation, or any adverse reactions that could endanger a patient’s life.
Secondly, biocompatibility plays a crucial role in patient outcomes. When patients receive implants or medical devices that show excellent compatibility with their bodies, there is a marked decrease in post-operative complications. In contrast, poor biocompatibility can result in device failure and may ultimately lead to the need for additional surgeries, sometimes resulting in lasting health issues.
"A good biocompatible material can be the difference between a successful procedure and a complicated recovery."
Moreover, biocompatibility concerns influence the very design processes of biomedical products. If a material is found to elicit a strong immune response, researchers might opt for modifications or to explore alternative materials altogether. This adaptability not only enhances the safety profile of medical devices but also promotes innovation, as researchers are continually pushed to develop better, safer solutions for patient care.
Key Components of Biocompatibility
Understanding biocompatibility involves recognizing various core components that influence how materials behave when introduced into the body. Here are several key elements:
- Chemical Composition: Different materials possess distinct chemical makeups which influence their behavior in biological environments. Metals, polymers, and ceramics each interact uniquely with tissues and fluids.
- Surface Properties: The surface characteristics of a material, including roughness, hydrophilicity, and charge, significantly affect protein adsorption and cell behavior, thus playing a major role in biocompatibility.
- Mechanical Properties: Mechanical integrity and specific attributes such as elasticity or tensile strength are critical for ensuring that a device performs as expected under physiological conditions.
- Biodegradability: For certain applications, materials that can safely break down within the body are vital. Non-biodegradable materials could induce chronic inflammation if they persist long-term.
The assessment of these components forms the backbone of biocompatibility evaluations, driving continuous improvement in material science and clinical applications. Therefore, researchers and developers need to have a holistic understanding of these factors to ensure the safety and effectiveness of biomedical products in real-world scenarios.
Historical Background
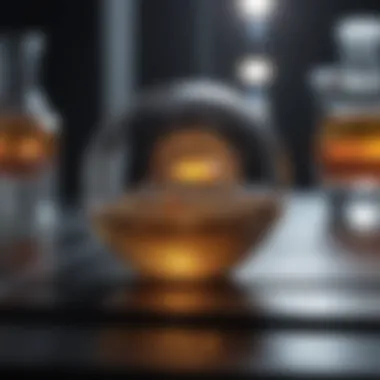
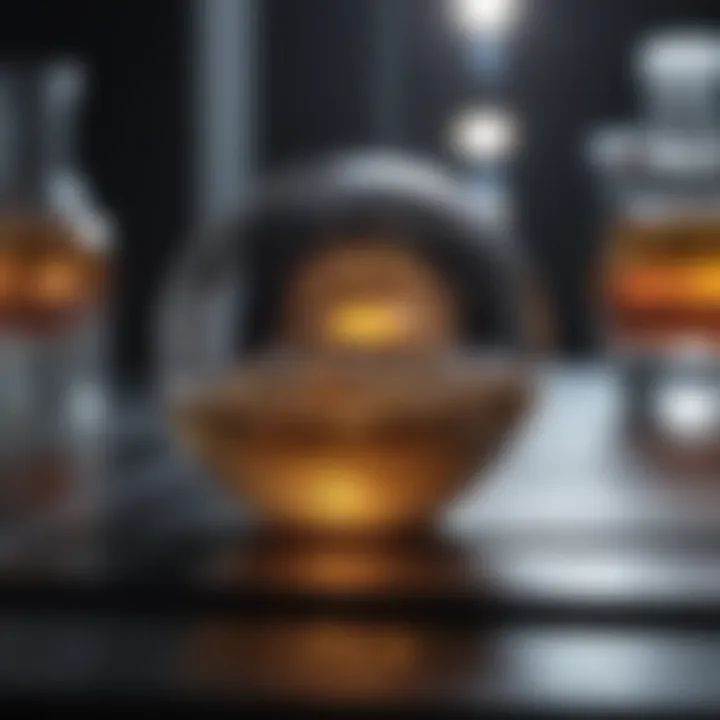
Understanding the historical backdrop of biocompatibility is like piecing together a jigsaw puzzle that reveals the journey of medicine in harmony with materials. Ever since humans have ventured into the realm of medical interventions, the interplay between biological systems and foreign materials has been a vital concern, shaping the evolution of biocompatibility standards.
In the early days, materials used in medicine—like metals, wood, and animal products—were chosen based on availability and practical considerations rather than rigorous testing. This often led to unforeseen complications, as observed with materials like ivory or certain metals that triggered severe immune responses. The shift towards a more systematic approach began in the mid-20th century, spearheaded by pioneers who recognized the need for standardized methods to evaluate how materials could safely interact with biological tissues.
The Evolution of Biocompatibility Standards
As the 1960s rolled in, the demand for safe and effective medical devices surged, parallel to the advances in materials science. The establishment of standards became paramount. Organizations like the International Organization for Standardization (ISO) began to draft guidelines that specified testing criteria to assess biocompatibility. The ISO 10993 series, for instance, emerged as a significant framework, focusing on biological evaluation while considering various factors, including toxicity, systemic effects, and genotoxicity.
These standards have evolved through collaborative efforts among researchers, clinicians, and regulatory bodies. The continuous refinement of these benchmarks reflects a growing understanding of immune responses and tissue interactions, resulting in the implementation of advanced testing techniques. Today, a combination of in vitro, in vivo, and computational methods is utilized to offer a comprehensive assessment.
"Biocompatibility is not just a box to tick; it represents the crucial interface between man-made materials and the complexities of biological systems."
Milestones in Biocompatibility Research
Each stride in biocompatibility research can be marked by milestones that indicate significant progress. One notable milestone occurred in 1986, when the FDA introduced guidelines for testing biocompatibility, aligning closely with international standards. This prompted manufacturers to prioritize safety and efficacy, ensuring their products met stringent requirements before reaching the market.
Furthermore, the introduction of tissue engineering concepts has revolutionized the field. By moving towards regenerative medicine, researchers have made headway in using biocompatible scaffolds that not only support tissue growth but also actively promote healing. The formulation of synthetic polymers, bioactive glass, and metals with tailored surface properties has opened avenues for innovative applications that adhere to the principles of biocompatibility.
The historical perspective on biocompatibility brings forth a critical understanding of not just past mistakes, but also the relentless pursuit of safety and quality in medical interventions. This not only protects patients but also enhances the trust between healthcare providers and medical technology.
Regulatory Standards for Biocompatibility
In the realm of biomedical research, regulatory standards for biocompatibility carry a hefty suitcase of importance. They act as a compass, guiding researchers and developers toward ensuring that medical devices and materials are safe for human use. Compliance with these standards is not merely a bureaucratic box to tick; it's essential for protecting patient safety and enhancing the efficacy of medical innovations.
These standards outline the methodologies for testing biocompatibility and set the bar for acceptable rates of biological safety. Embracing regulatory guidelines can prevent disaster down the road, potentially saving lives and defending organizations from legal ruination. Bottom line? Proper adherence to established standards is both a moral obligation and a sound business strategy.
International Organization for Standardization (ISO)
The International Organization for Standardization, often just called ISO, stands as a pivotal establishment in the field of biocompatibility. Its guidelines help in shaping the criteria that materials must meet before they can be deemed safe for use in medical applications. For example, ISO 10993 is a series of standards that specifically address the evaluation of medical device biocompatibility.
When researchers align their testing protocols with ISO standards, they gain a reputable edge. This can translate into smooth sailing through regulatory approvals, as ISO compliance is widely recognized across different countries. Furthermore, the ongoing revisions of these standards ensure that the protocols stay current, reflecting advances in technology and scientific understanding.
- Key components of ISO standards include:
- Detailed testing methodologies for various biological interactions
- Requirements for risk assessment
- Guidelines on documentation and data interpretation
Adhering to ISO standards is not just good practice; it’s a smart move in the complex landscape of biomedical research, where change is the only constant.
FDA Guidelines on Biocompatibility Testing
The U.S. Food and Drug Administration (FDA) also plays a crucial role in setting the stage for biocompatibility evaluations. Its guidelines serve as the playbook for developers wanting to introduce new devices or materials into the market. The FDA has laid down specific criteria that outline how to conduct biocompatibility testing effectively.
One standout aspect of these FDA guidelines is their emphasis on a systematic approach to safety evaluation. Researchers must provide robust evidence demonstrating that their materials do not elicit adverse biological responses. This essentially means that only those products that can give clear and convincing results stand a chance to make it through the rigorous approval process.
"FDA’s critical goal is ensuring that any medical device is safe and effective; hence, they detail robust review processes that every developer must follow."
Some of the high-priority tests mentioned in FDA guidelines are:
- Cytotoxicity Tests: Assessing the potential damaging effects of materials on cells.
- Sensitization Tests: Evaluating the likelihood of materials causing allergic reactions in users.
- Irritation Tests: Determining whether the device’s surface may irritate biological tissues.
As biocompatibility evaluations evolve, the FDA continues to refine its guidelines, thus ensuring that they provide relevant and actionable insight for researchers and manufacturers alike. By following FDA protocols, developers can efficiently navigate the often-turbulent waters of regulatory submission, maximizing their chances for success.
In summary, when it comes to regulatory standards for biocompatibility, both ISO and FDA guidelines significantly influence the landscape. Their importance cannot be undersold, as adherence not only fosters safe medical innovations but also cultivates trust in the biomedical field. This is crucial for the ongoing development of safe and effective healthcare solutions.
Methods of Biocompatibility Evaluation
Understanding the methods used to evaluate biocompatibility is vital in biomedical research. These evaluations not only ensure the safety and efficacy of medical devices and materials but also set a solid foundation for innovation in healthcare solutions. By employing a range of methodologies, researchers can predict how a material interacts with living organisms, which can prevent adverse outcomes in the clinical setting. Each method comes with its unique advantages and considerations, making it critical to select the appropriate evaluation technique based on specific application needs.
In Vitro Testing Techniques
In vitro testing refers to experiments conducted outside of living organisms, typically in controlled laboratory environments. These techniques offer several perks: they are often less expensive, quicker to perform, and ethically safer than in vivo methods. Common tests include cytotoxicity assays, where researchers assess cell viability in the presence of a material. Two frequently used assays are the MTT assay and the Lactate Dehydrogenase (LDH) release assay. Each provides critical data about how materials may impact cell health.
While in vitro methods have their merits, they do come with inherent limitations—mainly the inability to accurately replicate the complex environment of a living organism. Factors such as biochemical signaling and immune responses can vary significantly in vivo, making it essential to interpret in vitro findings with caution.
In Vivo Testing Procedures
In vivo testing, conducted within a living organism, greater reflects real-life biological interactions. These studies provide comprehensive insights into how materials perform in the physiological environment. Common procedures in biocompatibility testing include implant studies and systemic toxicity assessments.
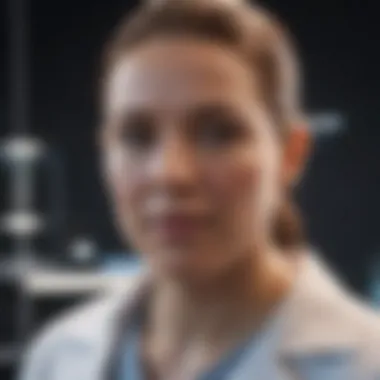
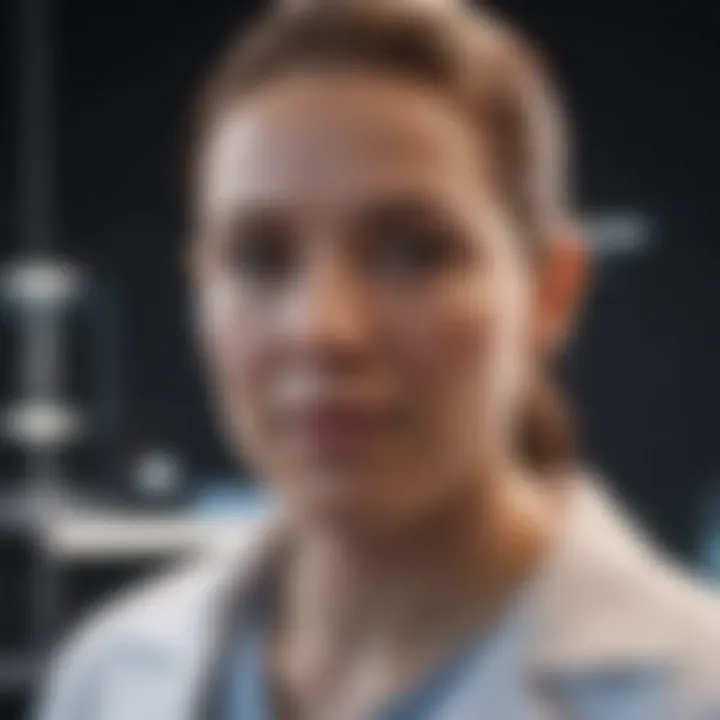
For instance, when evaluating an implantable device, researchers may observe the local tissue response and any inflammatory reactions over time. Rodents or rabbits often serve as model organisms, with specific guidelines requiring ethical approvals, thus raising ethical concerns that must be addressed.
Since in vivo testing can be resource-intensive and time-consuming, it often serves as a confirmatory approach rather than a standalone evaluation method. This reflective approach highlights the importance of combining in vitro and in vivo studies for a balanced assessment of biocompatibility.
Computational Models in Biocompatibility Assessment
The integration of computational models in biocompatibility assessment presents a forward-thinking approach to understanding material interactions. By leveraging simulation techniques and algorithms, researchers can predict biological response based on material characteristics and anticipated interactions at the molecular level.
Machine learning algorithms can analyze massive datasets, generating predictions about materials' safety before physical testing. This not only speeds up the evaluation process but also reduces costs associated with animal testing, making it an attractive option for researchers.
Utilizing these computational models could potentially reveal insights that traditional methods might miss, such as long-term material degradation or unforeseen interactions within cellular environments. The validity of these models heavily relies on the quality of data fed into them, thus requiring continuous refinement and validation against empirical results.
In the evolving field of biomedical research, a blend of innovative testing techniques and established methodologies creates a foundation for improving patient safety and advancing medical science.
By understanding these diverse methods of biocompatibility evaluation, researchers and professionals can make informed decisions in their work, paving the way for safer and more effective medical interventions.
Factors Affecting Biocompatibility
Understanding the factors affecting biocompatibility is crucial, as it plays a significant role in ensuring the safety and effectiveness of medical materials and devices. This topic dives into various components that influence how materials interact with biological systems. Focusing on elements such as material characteristics, surface properties, and mechanical properties provides a comprehensive view of the interplay between materials and biological responses, which is pivotal for advancing biomedical research.
Material Characteristics
The intrinsic properties of materials are fundamental in determining their biocompatibility. Here, we delve into several key aspects:
- Chemical Composition: The elemental makeup of a material can dictate its reactivity with bodily fluids or tissues. For instance, metals like titanium are favored in implants due to their corrosion resistance and biocompatibility, which stems from their oxide layer that minimizes inflammatory responses.
- Structure and Morphology: The physical architecture of a material can significantly impact cell adhesion and growth. For example, porous structures are often engineered for scaffolding in tissue regeneration as they mimic extracellular matrices, facilitating cellular infiltration and integration.
- Biodegradability: The rate at which a material degrades inside the body is critical. Sutures made from polylactic acid are designed to break down over time to minimize long-term foreign body reactions, demonstrating how controlled degradation can enhance biocompatibility.
Highly characterized materials foster better integration with biological systems, thus accentuating the importance of thorough understanding of material traits when developing biomedical solutions.
Surface Properties and Their Influence
Surface properties emerge as a pivotal factor in biocompatibility evaluations, affecting the interaction between biomaterials and biological entities. Several aspects warrant attention:
- Surface Roughness: The texture of a surface can encourage or hinder cell attachment. Generally, moderately rough surfaces enhance osteoblast (bone cell) adhesion, aiding in quicker bone integration for implants.
- Hydrophilicity vs Hydrophobicity: How a material interacts with water also plays a role. Hydrophilic surfaces often promote better protein adsorption, which is essential for cellular responses. For example, poly(ethylene glycol) coatings can be utilized to prevent protein adsorption deliberately, thus minimizing biofouling in medical devices.
- Functionalization: Tailoring surfaces with specific molecules can modify the biological response. Adding peptides that promote cell adhesion can drastically improve the performance of biomaterials in various medical applications.
All these factors underscore the significance of meticulous surface engineering in achieving favorable biological interactions, thereby enhancing the overall biocompatibility.
Mechanical Properties and Biocompatibility
Mechanical properties not only determine how a material performs under stress but also influence its compatibility with surrounding biological tissues. Important considerations include:
- Elastic Modulus: The stiffness of a material impacts how it performs in vivo. A mismatch between the implant's stiffness and that of surrounding tissues can lead to stress shielding, where bone resorption occurs due to inadequate load-bearing on the implant. Optimal matching is crucial in orthopedic implants.
- Tensile Strength: A material's ability to withstand stretching is vital, especially in applications such as cardiovascular devices. A material that fails under stress can provoke adverse reactions and device failure.
- Fatigue Resistance: Repeated stress exposure can lead to material fatigue. For example, coronary stents must be designed to endure the cyclic loading of the heartbeat without failure over time.
Incorporating knowledge of mechanical properties is key to ensuring longevity and effectiveness in medical materials, illustrating a critical dimension of biocompatibility evaluation.
The concept of biocompatibility is multifaceted, encompassing an array of material characteristics, surface interactions, and mechanical behaviors that ultimately dictate the success of biomedical devices and their integration into the body.
Challenges in Evaluating Biocompatibility
Evaluating biocompatibility is not simply a box-ticking exercise; it's a complex field that poses several challenges. Understanding these challenges is crucial because they can significantly impact the safety and effectiveness of biomedical materials and devices. This section will dig into the minutiae of difficulties faced during biocompatibility assessments, revealing why addressing these issues is vital for technological advancement and patient welfare.
Variability in Testing Results
One of the most prominent challenges in evaluating biocompatibility is the variability in testing results. Various factors can cause discrepancies in outcomes, leading to different interpretations of a material's compatibility with biological systems. Consider the in vitro testing, where cell cultures are used to evaluate materials. The cell line chosen, the growth factors administered, or even the environmental conditions—like temperature and pH—can all alter results. It’s akin to trying to hit a moving target without a clear aim.
Variants in in vivo assessments, such as the choice of animal models or the specific anatomical locations for implantation, further contribute to this inconsistency. As a case in point, a study might show that a certain scaffold material is well-tolerated in one species but induces adverse reactions in another. Consequently, the variation leads to a lack of reproducibility and reliability, raising questions about the risk assessments associated with these materials.
In the grand scheme, this variability can lead to costly delays in product development, lost investment, and more critically, potential risks to patient safety if a material deemed "safe" later shows harmful effects in broader applications. Thus, researchers need to adopt standardized protocols and consideration of genetic diversity among test subjects to enhance the reliability of testing outcomes.
Ethical Considerations in Animal Testing
The ethical landscape surrounding in vivo testing presents another layer of complication. Despite being an essential part of the biocompatibility evaluation process, the use of animals raises significant ethical dilemmas. Researchers are often caught between conducting thorough safety assessments and adhering to compassionate treatment of animal subjects. The public scrutiny and demand for humane practices have intensified pressure to rationalize the use of animal testing.
From the perspective of scientific integrity, the ethical concerns necessitate the development of alternative methodologies. Technologies such as organ-on-a-chip and sophisticated computational models aim to reduce reliance on animal testing by simulating human biological functions more accurately. These approaches show promise but still lack wide acceptance in regulatory frameworks, leading to a tug-of-war between innovation and tradition.
"While scientific advancement is pivotal, it should not come on the backs of living creatures. We must balance innovation and animal welfare in biocompatibility testing."
Moreover, ethical considerations are not merely philosophical; they have practical ramifications. Legal actions against firms for unethical testing practices can delay research and tarnish reputations. Thus, embracing alternative testing methods and refining existing protocols is not just a matter of compliance, it's a necessity for ethical integrity and optimal biocompatibility evaluation.
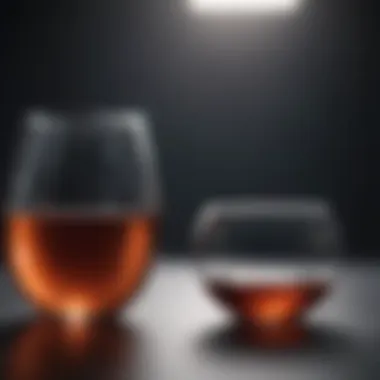
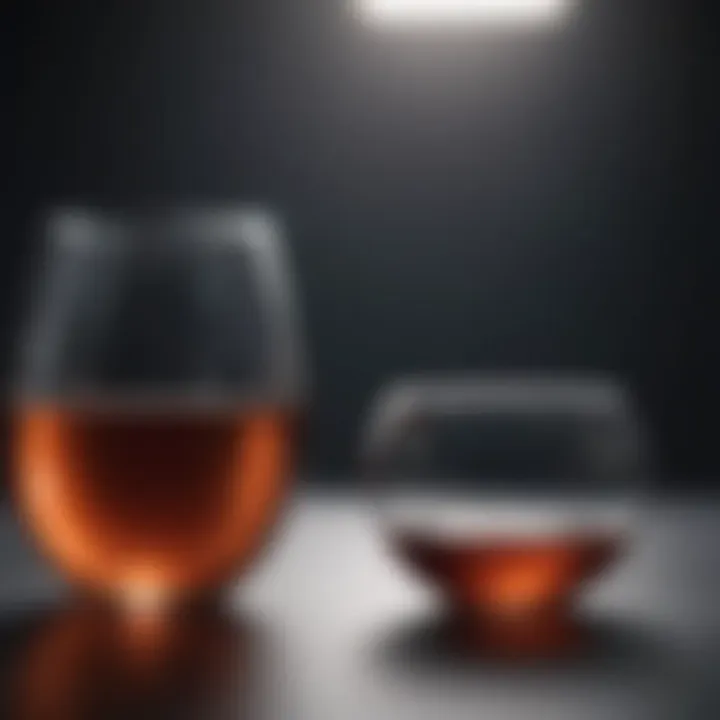
Recent Advances in Biocompatibility Testing
The realm of biocompatibility testing is continually evolving, with significant advancements making waves in recent years. Understanding these developments is crucial for professionals and researchers who are keen on ensuring the safety and efficacy of biomedical materials.
Innovations in biocompatibility testing not only streamline the assessment processes but also enhance the reliability and relevance of test outcomes. The need for more robust testing methods arises from the increasing complexity of biomedical devices and advancements in material science. Indeed, as the field grows, so does the imperative for cutting-edge approaches that can adapt to novel materials and applications.
Innovative Materials and Their Evaluation
One area of considerable progress in recent biocompatibility testing is the development of innovative materials. Traditional materials like stainless steel and titanium have been fairly standard for implants. However, newer biomaterials such as bioresorbable polymers and advanced ceramics are surfacing with properties tailored for specific medical applications. These materials can promote faster healing and reduce the risk of complications, making them appealing alternatives.
Evaluating these new materials involves rigorous testing to ascertain their interactions with biological tissues. For instance, researchers now employ advanced in vitro models that mimic the human environment more accurately than ever before. Such models can include co-culture systems, where multiple cell types are involved, providing a fuller picture of how a material will behave in actual human tissue. The following are some advancements in evaluation techniques:
- Microscopy Techniques: Utilizing techniques like scanning electron microscopy (SEM) provides insights into the surface morphology of new materials at nano-micrometer resolution.
- High-Throughput Screening: This approach allows for rapid testing of numerous material compositions simultaneously, identifying potential candidates that demonstrate desirable biocompatibility characteristics.
- Real-Time Monitoring: Advances in biosensors enable scientists to monitor cellular responses to materials in real time, thus capturing the dynamic nature of cell-material interactions.
These methodologies are setting a new standard in assessing innovative materials, offering deeper insights and ensuring that the products entering the market are both safe and effective.
Biocompatibility in Drug Delivery Systems
Another noteworthy advancement is in the domain of drug delivery systems. As the complexity of therapies increases, so too does the importance of ensuring that the materials used for delivery are biocompatible. Recent studies focus on optimizing drug formulations that utilize nanoparticles or hydrogels. These systems not only need to release the drug effectively but must also do so without eliciting adverse immune responses.
The testing of these systems is multi-faceted. For instance, researchers must evaluate:
- Release Profiles: How quickly and efficiently drugs are released from the carrier materials, which can directly influence therapeutic outcomes.
- Tissue Interaction: Understanding inflammation responses and biocompatibility at the material's surface is vital for ensuring patient safety.
- Long-term Effects: Evaluating the degradation products of drug delivery systems can highlight potential toxicities not apparent in short-term studies.
"The integration of new materials in drug delivery is fundamentally reshaping our approach to treatment; ensuring these materials are biocompatible is non-negotiable."
Case Studies of Biocompatibility Assessments
The exploration of biocompatibility through case studies serves as a pivotal cornerstone for understanding how materials interact within the biological systems of living organisms. These assessments not only highlight the practical applications of theoretical principles but also provide tangible data that researchers and practitioners can rely on. By focusing on specific cases, experts can illustrate the effectiveness of materials used in medical environments while uncovering real-world challenges faced in evaluating biocompatibility.
Studying these examples sheds light on notable practices and the consequent improvements in patient safety and device efficacy. Engaging with concrete instances helps elucidate the sometimes abstract concepts surrounding biocompatibility and makes the evaluations more relatable and easier to grasp.
Implantable Medical Devices
Implantable medical devices have revolutionized healthcare, providing life-enhancing solutions, but they bring forth unique challenges in their biocompatibility assessments. Cases like cardiac pacemakers and hip implants exemplify the complexity involved in evaluating how these devices behave once placed inside the human body.
- Rigorous Testing: For a cardiac pacemaker, extensive testing is mandatory to ensure it can withstand the body's mechanical and chemical environments without adverse reactions.
- Long-Term Biocompatibility: The longevity of devices like hip implants necessitates an evaluation of their performance over extended periods, since any failure could result in severe complications.
- Integration with Body Tissues: A successful implant integrates seamlessly with surrounding tissues, preserving function while minimizing risks like inflammation or rejection.
Through systematic observations, medical professionals can gather insights about these devices, leading to improvements in design and materials used.
Scaffolding Materials in Tissue Engineering
Tissue engineering represents a promising frontier in regenerative medicine, utilizing scaffolding materials to support cell growth and tissue regeneration.
Assessing the biocompatibility of these scaffolds is crucial as they directly affect the efficacy of therapeutic outcomes.
- Material Composition: Case studies often look at various biodegradable polymers, such as polylactic acid, which have been favored for their compatibility and support of cellular activity.
- Cellular Response: Monitoring cell attachment, proliferation, and differentiation on scaffolds allows researchers to refine their approaches and ensure optimal conditions for tissue regeneration.
- In Vivo Performance: Evaluations under animal models provide essential data on how these scaffolds interact with biological systems, which is vital for understanding potential outcomes when applied in human medicine.
The importance of biocompatibility assessments in these cases cannot be overstated, as they pave the way for innovations in treatment options, ultimately enhancing patient care and contributing to more effective healthcare solutions.
In summary, studying biocompatibility through real-world cases in implantable medical devices and scaffolding materials fosters a deeper understanding of their significance. It illuminates crucial factors affecting patient safety and the efficacy of medical interventions while encouraging continual advancement in evaluation strategies.
Future Trends in Biocompatibility Research
The landscape of biocompatibility research is rapidly evolving, driven by technological advancements and an increasing emphasis on personalized medicine. Understanding these trends is pivotal as they not only shape the future of medical devices and materials but also have significant implications for patient safety and therapeutic efficacy. Being ahead of the curve in biocompatibility can ensure that materials developed meet the stringent safety standards required by health authorities. In this section, we explore two significant aspects that are transforming the field: the role of biomaterials science and the integration of artificial intelligence and machine learning in evaluations.
The Role of Biomaterials Science
Biomaterials science stands at the forefront of biocompatibility developments. This field merges principles from biology, engineering, and materials science to innovate materials that are not just compatible but also proactive in biological environments. Each biomaterial, whether natural or synthetic, possesses unique properties that can influence its interaction with biological systems. Recent driving forces in biomaterials science include:
- Customizability: Developments in polymer chemistry have led to materials that can be tailored to specific applications. Customized surface modifications can promote or inhibit certain cellular responses, enhancing biocompatibility.
- Smart Biomaterials: This category adapts to environmental changes—think hydrogels that respond to pH or temperature variations. Such responsiveness can greatly improve biocompatibility by mimicking natural biological processes.
- Sustainable Materials: There's a growing focus on eco-friendly biomaterials that minimize adverse effects on the environment and human health long after their use. Technologies using biodegradable materials exemplify this trend.
Incorporating these innovative materials into practical applications can significantly reduce the associated risks and side effects seen with traditional biomaterials. Research shows that better material compatibility leads to improved healing times and overall patient satisfaction.
Integrating AI and Machine Learning in Evaluations
The advent of artificial intelligence and machine learning has ushered in a new era of precision and efficiency in biocompatibility evaluations. These technologies offer the potential to analyze complex biological data sets significant for assessing biocompatibility.
Key areas where AI and machine learning are making strides include:
- Predictive Analytics: Algorithms can predict biocompatibility based on historical data sets, reducing the need for extensive in vitro and in vivo testing. This not only saves time but also resources.
- Data Processing: Machine learning tools excel at processing large volumes of information from various evaluation methods, identifying patterns that may go unnoticed by human analysts.
- Real-time Monitoring: AI-enhanced systems enable real-time analysis of cellular interactions with materials in controlled environments. This facilitates immediate adjustments to material formulations based on biological responses.
"The combination of AI and traditional testing methods can lead to a more holistic understanding of biocompatibility, tailoring materials to individual patient needs."
These future trends highlight an exciting path forward for the field of biocompatibility research. By leveraging the capabilities of modern science, researchers can strive towards developing safe, effective biomedical solutions that are harmoniously integrated with the human body.