The Essential Contributions of Material Scientists
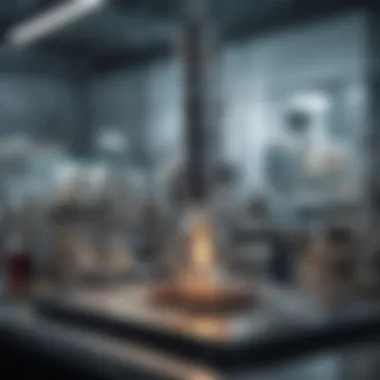
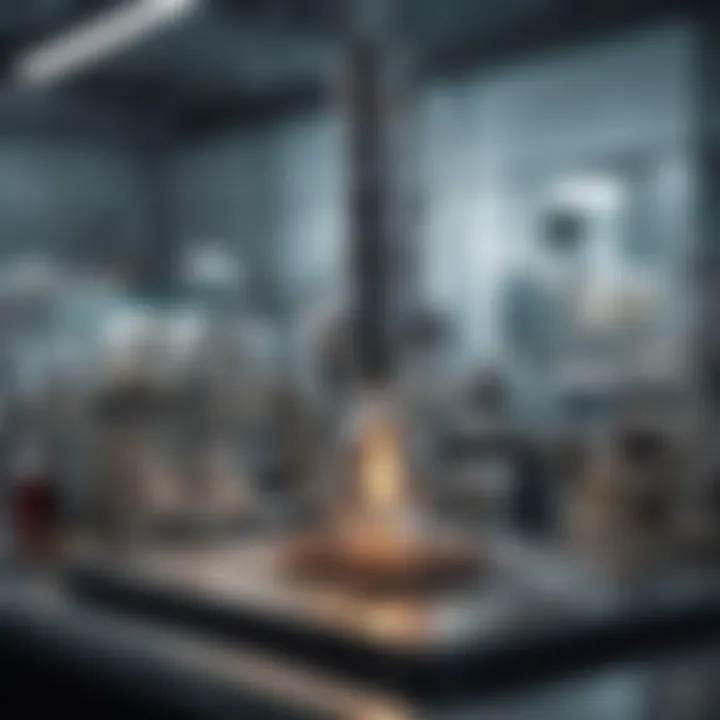
Intro
The field of material science stands at the forefront of technological innovation, transforming the very essence of materials that define our world. A material scientist is someone who delves into the properties, performance, and behaviors of various substances, ultimately shaping advancements across numerous industries—from aerospace to electronics. The intertwined relationship between materials and their applications makes it essential to understand the role of these scientists in both research and development.
Overview of Research Topic
Brief Background and Context
Material science is as old as civilization itself. From early humans experimenting with stone tools to contemporary researchers synthesizing nanomaterials, the quest to manipulate and understand materials has been a constant. The emergence of material scientists emerged during World War II when the demand for innovative materials surged, laying the groundwork for the modern understanding of this crucial discipline.
Today, material scientists seek to explore everything from polymers to metals and ceramics, blending chemistry, physics, and engineering principles. They often play a pivotal role in cross-disciplinary collaborations, where the demands of various sectors necessitate innovative solutions.
Importance in Current Scientific Landscape
In the current age, where technology rapidly evolves, material scientists are unsung heroes who help address pressing global challenges such as sustainability and climate change. They contribute significantly to advancements like renewable energy sources, lightweight materials for transportation, and biocompatible materials for medical applications. The work of material scientists touches on nearly every facet of daily life, and their influence remains profound as they strive to find new and better ways to utilize materials.
Methodology
Research Design and Approach
Material scientists often employ a blend of theoretical and practical research approaches. This combines computational modeling with experimental techniques. The aim is to gain a firm understanding of how different materials behave under various conditions. For instance, using software simulations, researchers can predict how materials will perform before physically creating samples.
Data Collection Techniques
Gathering data in material science happens through a variety of methods. Here are some common techniques:
- Microscopy: Techniques such as electron microscopy provide detailed images of materials at the atomic level, revealing their structure.
- Spectroscopy: This method involves analyzing how materials interact with different wavelengths of light, which helps identify their composition and properties.
- Mechanical Testing: To understand a material’s strength and durability, scientists often conduct stress-strain tests, measuring how materials respond to forces.
"Material science serves not just the ambition of innovation, but the practical requirements of society’s advancement. Without it, many modern achievements wouldn’t likely exist, highlighting its critical importance."
Understanding Material Science
Material science is a vital field that intersects with various aspects of modern life. Understanding this discipline is not just for academic purposes; it's integral for shaping technologies that can improve our daily lives and address towering challenges like sustainability and resource scarcity. The nuances involved in material science touch everything from the gadgets in our pockets to the structures we inhabit. It offers insights into how materials can be designed, modified, and applied to meet rigorous demands.
The importance of delving into material science lies in its multifaceted nature. It encapsulates the study of materials' properties—how they behave under different conditions and how they can be manipulated. This understanding paves the way for innovations that can propel industries forward.
Definition and Scope
Material science focuses on the examination and application of various materials, including metals, polymers, ceramics, and composites. This discipline straddles physics, chemistry, and engineering, creating a rich environment for discovering new materials tailored for specific uses. For instance, a material scientist may work on developing lightweight composites that enhance fuel efficiency in aviation.
- Definition: Material science studies materials and their applications.
- Scope: It involves research, development, and testing of materials in various sectors.
The scope of material science is vast, affecting a range of industries. From designing safer vehicles to creating biocompatible implants, its reach is significant. Knowledge in this field translates into practical applications, impacting health, energy, electronics, and environmental sustainability.
Interdisciplinary Nature
Material science is not confined to a single discipline; it's inherently interdisciplinary. It requires knowledge from physics to understand the atomic structure of materials, chemistry to manipulate chemical properties, and engineering for practical applications. This blend fosters innovation, as ideas and techniques from various fields converge.
- Collaboration is Key: Material scientists often collaborate with chemists, engineers, and physicists to tackle complex problems.
- Emerging Technologies: Awareness of trends in fields like nanotechnology is crucial, as these can influence new material development.
The interdisciplinary nature of material science resonates with several real-world applications. For instance, consider the development of new battery technologies. By understanding not just the chemistry of battery materials but also their mechanical properties and environmental impact, material scientists contribute to creating longer-lasting, more efficient batteries that support the rise of electric vehicles.
In summary, understanding material science provides a foundation for ongoing innovation. As the world navigates challenges like climate change and technological advancement, the role of material scientists becomes increasingly crucial. They are at the forefront, driving progress through advanced materials tailored to our evolving needs.
Key Responsibilities of Material Scientists
Understanding the key responsibilities of material scientists provides insights into their critical role within the field of material science. The work of these professionals not only drives innovation but also holds the key to overcoming numerous challenges faced by modern industries. Material scientists are at the forefront of developing new materials and improving existing ones, which makes their responsibilities paramount in sectors ranging from electronics to construction.
Research and Development
Research and development (R&D) represent the backbone of material science. In this phase, material scientists engage in rigorous experimentation to create or enhance materials to meet specific needs. It’s like throwing spaghetti at the wall to see what sticks, only in this case the goal is a high-performance polymer, a more efficient battery, or a groundbreaking composite material.
R&D is not just about trial and error though; it requires a hefty dose of creativity coupled with a scientific approach. For instance, if a material scientist is tasked with developing a stronger, yet lighter material for aerospace applications, they might delve into advanced composites or explore nano-engineering. The impact of their findings can ripple through the entirety of the manufacturing processes, influencing everything from prototypes to production methodologies.
Material Characterization
Following R&D, material characterization takes center stage. This process involves determining the properties and behaviors of materials, which is crucial to understanding their suitability for particular applications. This responsibility encompasses a range of techniques including spectroscopy, microscopy, and mechanical testing.
From the minutiae of atomic structure to macroscopic mechanical properties, material scientists use characterization to build a comprehensive profile of materials. For example, they might analyze a new alloy's tensile strength and corrosion resistance before it’s used in an automotive application. This step ensures safety and functionality, effectively guarding against potential failures that could arise from inadequate material properties.
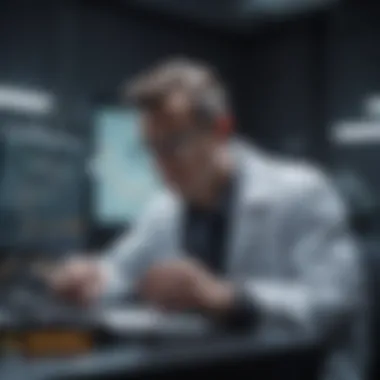
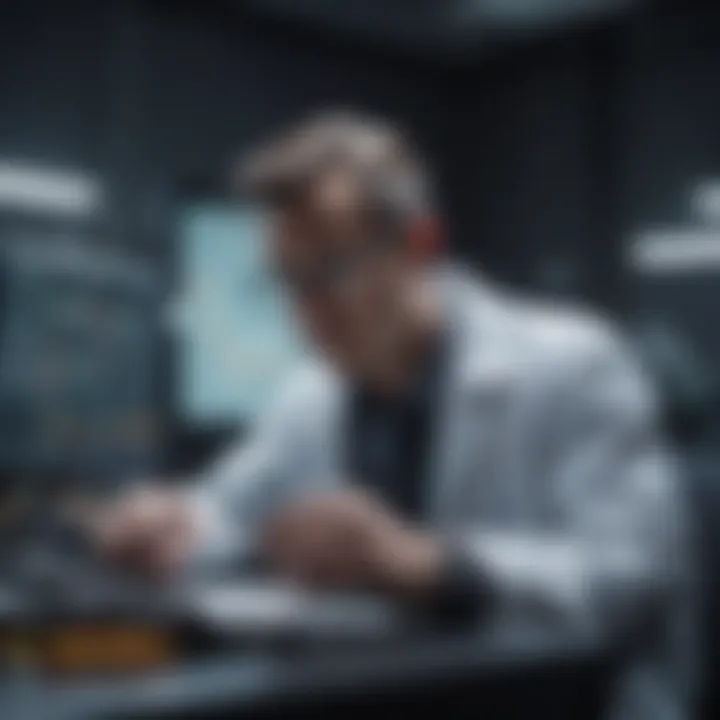
Collaboration with Engineers
Collaboration with engineers is yet another vital responsibility. Material scientists are not lone wolves; they work closely with engineers from various disciplines – civil, mechanical, aerospace, you name it. This teamwork is essential to integrating new materials into products and structures, ensuring that materials meet design requirements without compromise.
Take the example of an automobile manufacturer working on a new electric vehicle. Material scientists would partner with engineers to identify lightweight materials that can extend battery life while maintaining structural integrity. As they exchange knowledge and insights, the end goal is a product that performs excellently—efficiently and safely. Having a collaborative approach ensures that all voices are heard in the material selection process, leading to innovations that could well revolutionize industries.
Quality Control and Testing
Last, but certainly not least, is the aspect of quality control and testing. Material scientists have a responsibility to ensure that every material meets stringent regulations and standards. This may involve rigorous testing protocols to evaluate aspects like durability, safety, and performance.
Think of it as a double-check system; without it, any advancements made could be detrimental. Failure to adhere to quality norms can lead to significant setbacks—not only financially but also in safety and reliability. For instance, materials used in aerospace applications undergo extensive testing under various conditions before they even consider entering production. This aspect ensures that products stay reliable and uphold industry standards, presenting material scientists as both innovators and protectors.
"The role of a material scientist encompasses a mixture of creativity, precision, and collaboration. Their work impacts a multitude of industries and paves the way for future innovations."
Through these responsibilities, material scientists shape the way products are conceived and manufactured. Their contributions are essential not just for technological advancements but also for the society at large.
Methods and Techniques Used
The methods and techniques employed in material science are fundamental to the exploration and advancement of this field. They not only assist material scientists in understanding the properties of various materials but also pave the way for innovative applications across multiple industries. The importance of these methods cannot be overstated, as they are the backbone of research and development efforts in material science.
Synthesis Techniques
Synthesis techniques refer to the methods used to create new materials. These are critical because the properties of a material are often dictated by how it is made. One widely-used approach is the sol-gel process, where a colloidal solution turns into a solid gel, thus creating highly porous materials suitable for coatings or composites. Another notable method is chemical vapor deposition (CVD), which is crucial for producing thin films in electronics. Properly tuning these techniques can result in materials with better strength, conductivity, or light-harvesting capabilities.
- Benefits
- Diverse range of applications
- Ability to engineer materials at the atomic level
- Control over material properties
Considerations about synthesis techniques involve scalability and environmental impact. Not all synthesis methods can transition from the lab to large-scale production without losing their advantageous properties. Plus, eco-friendliness is now a priority, reasoning why innovators pursue greener alternatives.
Analytical Methods
Analytical methods help characterize materials and understand their behaviors under various conditions. Techniques such as X-ray diffraction (XRD) and scanning electron microscopy (SEM) are indispensable tools for material scientists. XRD allows for determining crystalline structures, while SEM provides insights into surface morphology on a microscopic level.
"The continuous evolution of new analytical techniques allows material scientists to dig deeper into the material's properties, leading to discoveries that can reshape technologies."
- Common analytical methods include:
- Thermogravimetric analysis (TGA)
- Fourier-transform infrared spectroscopy (FTIR)
- Raman spectroscopy
Utilizing these methods enables scientists to draw connections between a material's structure and its potential applications, ensuring performance reliability in the field.
Computational Modeling
With growing computational power, the field of material science has seen a significant shift from empirical experimentation to model-driven research. Computational modeling can predict material properties before physical testing, effectively saving time and resources. For example, tools like density functional theory (DFT) can forecast how a new compound behaves at the atomic level. This capability allows for the rapid screening of materials for various applications.
- Advantages of computational modeling:
- Decreases the need for extensive lab testing
- Identifies potential materials for innovative uses
- Facilitates collaboration with software engineers and data scientists
However, reliance on computational methods must be balanced. Modeling may not always accurately predict real-world behaviors due to unforeseen environmental conditions or material defects.
Nanomaterials Exploration
Nanotechnology is the beating heart of modern material science, offering immense potential for innovation across various sectors such as electronics, health, and renewable energy. Nanomaterials showcase unique properties due to their size and surface area-to-volume ratios. For instance, carbon nanotubes possess exceptional tensile strength and electrical conductivity, making them prime candidates for applications in flexible electronics and composite materials.
- Research areas in nanomaterials include:
- Nanoparticles for drug delivery in pharmaceuticals
- Nanoscale coatings for corrosion resistance
- Nanocomposites for enhanced durability in construction
While nanomaterials hold great promise, they also present challenges, including concerns over toxicity and the environmental impact of manufacturing processes. Addressing these challenges is paramount to ensure that advancements in nanotechnology align with sustainability goals.
Applications in Various Industries
The realm of material science weaves itself into numerous sectors, shaping innovations and solving complex challenges. The role of a material scientist extends beyond laboratory walls and academic papers; it translates into real-world applications that directly impact our daily lives. This article delves into how material science contributes to industry advancements, emphasizing the specific elements, benefits, and considerations inherent in each category.
Electronics and Semiconductors
In the fast-paced world of electronics, material scientists are the unsung heroes behind the scenes. They work with materials that conduct electricity efficiently, enabling the creation of smaller, faster, and more reliable devices. Consider silicon, a staple in semiconductors; it is not just any material, but one that has revolutionized computing and communication technologies.
Material scientists play a crucial role in optimizing the purity and properties of silicon wafers. By tweaking the doping process, they enhance conductivity and control electrical characteristics, which is vital for producing integrated circuits.
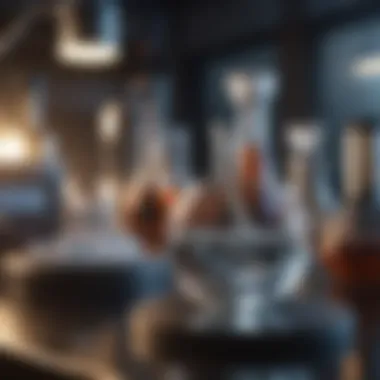
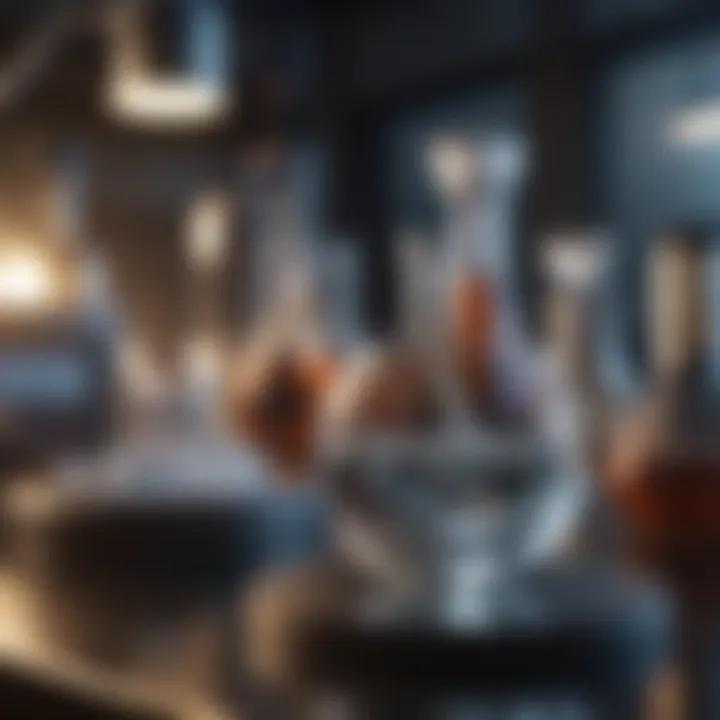
- Key Contributions:
- Development of advanced semiconductor materials such as gallium nitride and graphene.
- Improvement of energy efficiency in electronic devices through better materials.
- Innovations in flexible electronics, enabling wearable technology.
Their work ensures that devices not only function but do so with remarkable efficiency and longevity.
Biomedical Applications
The intersection of material science and medicine is a dynamic field that holds remarkable promise. Here, material scientists innovate solutions that directly affect patient care. From biocompatible materials used in implants to drug delivery systems, their contributions are pivotal.
For instance, titanium alloys are often used in orthopedic implants due to their strength and biocompatibility. Material scientists continue to explore new polymers and composites that can be used in various applications, including sutures and scaffolds that promote tissue regeneration.
- Noteworthy Aspects:
- Development of smart materials that can respond to environmental changes.
- Innovations in 3D printing technologies for bespoke medical devices.
- Research into biodegradable materials to reduce long-term waste in surgeries.
The emphasis on functionality, safety, and sustainability reflects the crucial nature of material science in healthcare.
Energy Sector Innovations
Energy is a cornerstone of modern society, and material scientists are at the forefront of innovations that aim to make energy production and consumption more sustainable. They research and develop materials that enhance the efficiency of energy conversion technologies, such as solar cells and fuel cells.
By designing materials with optimal surface properties, they can significantly improve the light absorption in photovoltaic cells, making solar energy a more viable option.
- Highlights of Their Role:
- Advancements in lithium-ion batteries, providing better energy storage solutions.
- Research into superconductors to revolutionize power transmission systems.
- Development of advanced catalysts for cleaner fuel production.
As the quest for cleaner energy sources escalates, the input from material scientists is more essential than ever.
Construction and Infrastructure
Within the construction industry, material science is a linchpin for architectural innovation and safety. Material scientists delve into the properties of concrete, metals, and composites to ensure structures are not just well-designed, but also enduring and resilient.
Recent developments in nanotechnology and smart materials pave the way for buildings that respond to environmental challenges—think self-healing concrete that can repair its own cracks over time.
- Key Factors Involved:
- Use of high-performance materials that enhance durability and resistance to weathering.
- Research into sustainable materials that reduce environmental impact.
- Innovations in structural health monitoring systems.
Material scientists are integral in pushing the boundaries of what is possible, aligning construction practices with modern needs for sustainability and resilience.
The Impact of Material Science on Society
Material science doesn’t just dwell in labs filled with shiny beakers and advanced machinery; it plays an instrumental role in shaping the society we navigate daily. The science entwine with everyday life, influencing the quality of technologies, environments, and economies alike. By studying and developing new materials, scientists help tackle pressing global issues such as climate change, resource shortages, and health care challenges. Their work can lead to monumental advancements that foster sustainability and improve living standards for many.
Addressing Environmental Challenges
Today, material scientists are at the forefront of combatting environmental issues. By devising greener materials and production methods, they are crucial in diminishing pollution footprints and promoting environmental stability. For instance, consider biodegradable plastics and bio-based composites—these alternatives are not just fleeting trends but provide long-term sustainable solutions to ongoing plastic waste crises.
Moreover, advancements in energy-efficient materials, such as thermoelectric materials, open the doors for reduced energy consumption. With enhanced performance in energy harvesting or insulation, these materials significantly cut down on energy use in buildings, contributing to a lower carbon footprint.
"Material scientists innovate not just for today's needs, but future generations’ realities."
Advancements in Sustainable Materials
Sustainability in material design is hot on the agenda, with scientists finding novel ways to produce and utilize materials that lessen negative environmental impacts. The push for sustainable construction materials is one such example. Engineers working with bamboo or recycled concrete reflect how traditional ideas can be combined with modern technology to create eco-friendly building solutions. Not only does this approach mitigate environmental damage, but it also promotes resource conservation by using what is available more efficiently.
Additionally, researchers are excited about the potential of advanced materials like graphene. This material, touted for its strength and flexibility, has countless applications spanning from electronics to lightweight composite materials for vehicles. The scalability of producing such materials responsibly is key to integrating them into everyday use without aggravating existing ecological issues.
Societal and Economic Benefits
The ripple effect of advancements in material science rounds off nicely into socioeconomic advantages. By driving innovation, material scientists not only enhance product performance but also contribute to job creation within industries such as renewable energy, electronics, and construction. For instance, the development of affordable solar cells can make renewable energy more accessible, positively affecting the economy through job growth in manufacturing and installation sectors.
Furthermore, when companies adopt sustainable materials, they often see an improvement in their brand image. Consumers increasingly prefer brands that showcase commitment to environmental responsibility, resulting in a competitive edge in the market. This intersection of sustainability and economic growth demonstrates how material scientists are not merely working behind the scenes but are pivotal players in the game of societal advancement.
Educational Pathways for Aspiring Material Scientists
In the fast-evolving field of material science, educational pathways are essential to lay the groundwork for a successful career. The complexity of materials and their interactions requires a solid foundation in science and engineering. For individuals considering a career as a material scientist, understanding these pathways is vital, as they address not just the theoretical but also the practical dimensions of this discipline.
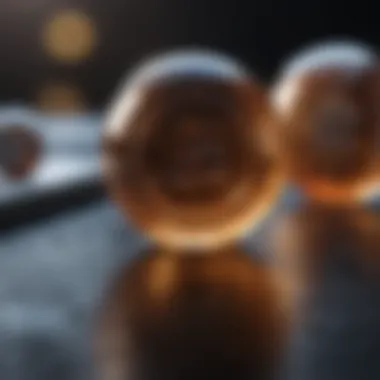
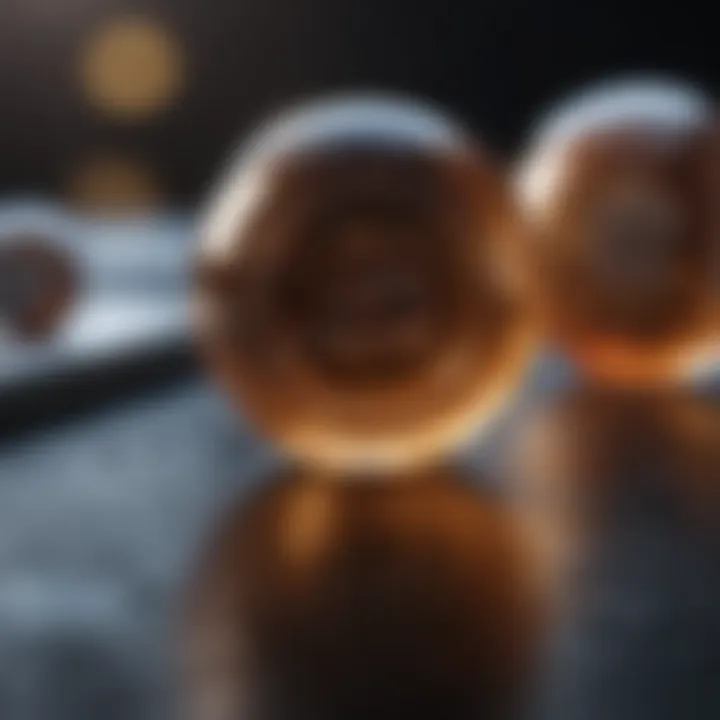
Degree Requirements
The journey typically begins with a bachelor’s degree in material science, materials engineering, chemistry, physics, or a related field. This foundational education introduces students to the principles of bonding, crystallography, and thermodynamics—cornerstones of material properties.
Many universities nowadays offer specialized programs focusing on advanced materials, nanotechnology, and polymer science. A strong emphasis on laboratory work is crucial, as it equips students with hands-on experience. Furthermore, pursuing a master's or doctorate degree amplifies one's understanding and research abilities, providing a competitive edge in the job market. Graduate programs often delve deeper into specialized topics such as:
- Nanomaterials and their applications
- Biomaterials for medical use
- Composite materials in aerospace
- Smart materials in electronics
A graduate degree not only enhances knowledge but is often a prerequisite for advanced research positions.
Essential Skills Development
While degrees provide the necessary academic background, developing a toolbox of essential skills is equally important. Material scientists need a mix of technical expertise and soft skills to navigate their roles effectively. Some pivotal skills include:
- Analytical Thinking: The capability to scrutinize data and results critically.
- Problem-solving Abilities: Addressing challenges in material design and implementation.
- Technical Proficiency: Familiarity with tools like scanning electron microscopes and finite element modeling software is invaluable.
- Collaboration: Working alongside engineers, chemists, and designers requires strong interpersonal skills.
Additionally, knowledge in computational modeling and data analysis is becoming increasingly necessary due to the rise of big data in materials science.
Internships and Practical Experience
Practical experience serves as a bridge between theory and application. Engaging in internships or co-op programs during or after an academic program grants students insights into the real-world workings of material science. Such experiences offer several advantages:
- Industry Exposure: Familiarity with the latest technologies and industry standards.
- Networking Opportunities: Connections that could lead to job prospects post-graduation.
- Skill Application: Applying classroom knowledge to solve practical problems in a controlled environment.
Students can seek internships in various sectors, including automotive, aerospace, electronics, and biomedical industries. Moreover, participating in research projects under a professor or institution can also enrich practical skills and deepen subject matter understanding.
"Internships are like the dress rehearsals of your career; it’s where you try out your skills before hitting the stage of the professional world."
Future Trends in Material Science
Future trends within material science are a driving force behind innovation across numerous industries. As the world shifts towards more sustainable solutions, the role of material scientists cannot be overstated. These professionals are at the forefront of developing new materials and technologies that not only meet contemporary demands but also anticipate future needs. This section explores emerging materials, the increasing influence of artificial intelligence, and the necessity for global collaborations in research—all positioned to reshape our understanding of what materials can do in a rapidly evolving technological landscape.
Emerging Materials and Technologies
The quest for materials that are lighter, stronger, and more efficient is driving innovations at a dizzying pace. Graphene, a single layer of carbon atoms arranged in a two-dimensional lattice, is one such material that has caught the attention of the scientific community. Its exceptional strength-to-weight ratio and conductive properties could revolutionize everything from electronics to medical devices. Researchers are also delving into bio-based materials that come from renewable sources, offering sustainable alternatives to traditional plastics.
Some key trends in emerging materials include:
- Smart Materials: These materials can respond to environmental changes. For example, shape memory alloys can revert to a predetermined shape upon heating, with applications in medical devices and robotics.
- Self-Healing Polymers: These materials can repair themselves when damaged, reducing waste and prolonging the longevity of products, an exciting field for manufacturers looking to enhance sustainability.
- 3D-Printed Materials: Advances in 3D printing are making it possible to create custom materials on demand, reducing the need for mass production and the associated waste.
Incorporating these emerging technologies into existing infrastructures not only facilitates enhanced performance but also poses significant challenges, requiring material scientists to continuously adapt and innovate.
Role of Artificial Intelligence
Artificial Intelligence is no longer a buzzword but a transformative tool in material science research. By processing vast amounts of data, AI can help identify patterns and predict how materials will behave under various conditions. This capability accelerates the materials discovery process, cutting down the time it takes to bring new products to market. Machine learning algorithms, for instance, can analyze existing datasets to propose new composites or suggest combinations of elements that scientists might not have previously considered.
Benefits of AI in material science include:
- Predictive Modeling: Utilizing AI algorithms helps in predicting the properties of new materials before they are synthesized, ultimately saving time and resources.
- Optimization of Manufacturing Processes: AI can analyze production data in real-time, allowing adjustments that can improve quality and efficiency in manufacturing.
- Enhanced Research Collaboration: AI-driven platforms can facilitate communication and collaboration among researchers globally, fostering a culture of shared discoveries and knowledge.
As AI continues to evolve, there is potential for new methodologies to surface, influencing an entire generation of material scientists and researchers.
Global Collaborations in Research
The challenges facing material science today are complex and multifaceted, necessitating a collaborative approach that crosses borders. Countries and institutions around the globe are pooling resources, talent, and knowledge to tackle shared challenges. Teams involving academic institutions, private industries, and government agencies are forming worldwide partnerships focusing on critical areas such as sustainable energy, health-care innovations, and climate resilience.
Key aspects of these collaborations include:
- Shared Expertise: By combining different schools of thought, researchers can benefit from diverse perspectives that lead to groundbreaking solutions.
- Resource Allocation: Global research initiatives allow for the sharing of expensive resources like labs and equipment, making cutting-edge research more accessible.
- Cross-Disciplinary Innovations: Collaborative projects often involve not just material scientists but also engineers, chemists, and biologists, driving revolutionary advancements that a single field might overlook.
This interconnectedness is crucial as it demonstrates how smooth collaboration can empower material scientists to innovate and solve pressing problems that impact society as a whole.
"Together, we can tackle the challenges of tomorrow by harnessing the power of material science hand in hand with others across disciplines and geographies."
The End
The conclusion of this article serves as a critical juncture, summarizing the key elements that frame the role of material scientists. Their expertise is indispensable in today’s world, where the complexity and range of materials play a vital role across multiple sectors — from electronics to pharmaceutical applications. This section aims to encapsulate the importance of understanding what material science embodies and the profession's significant contributions to advancements in technology and societal progress.
Summarizing the Importance of Material Scientists
Material scientists act as foundational figures in the innovation landscape. Their work not only enhances our understanding of materials but also paves the way for groundbreaking technologies. Here are several striking elements that highlight their importance:
- Innovation at the Core: Material scientists are the driving force behind the development of new materials like graphene and bio-degradable plastics. These innovations directly address environmental issues and lay the groundwork for upcoming tech.
- Interdisciplinary Collaboration: They collaborate closely with engineers, chemists, and physicists to create solutions that are not just theoretical but also applicable in real-world scenarios. This interdisciplinary nature fosters a richer educational environment, engendering a dynamic exchange of ideas that lead to enhanced results.
- Economic Contribution: As the backbone of industries ranging from aerospace to healthcare, material scientists contribute significantly to economic growth. Their ability to create high-performance materials leads to increased efficiency and sustainability, resulting in cost-effective solutions that benefit society.
- Addressing Challenges: In a time where environmental and health challenges loom large, material scientists are problem-solvers. They are tasked with developing sustainable options that lessen environmental impact while improving human health.
"The journey of material science is not just about materials themselves; it's about shaping the future with thoughtful innovations."
Ultimately, through their research, application, and expertise, material scientists emerge as pivotal actors in the narrative of technological progression. Their contributions resonate within the everyday lives of individuals, touching on everything from the devices we use to the materials that ensure safer, more sustainable living. Understanding their role in this complex interplay of science, industry, and society illuminates the path forward, carving a route toward a more innovative future.