Exploring the Hadron Collider: Insights and Discoveries
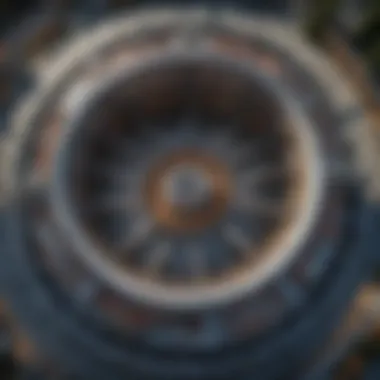
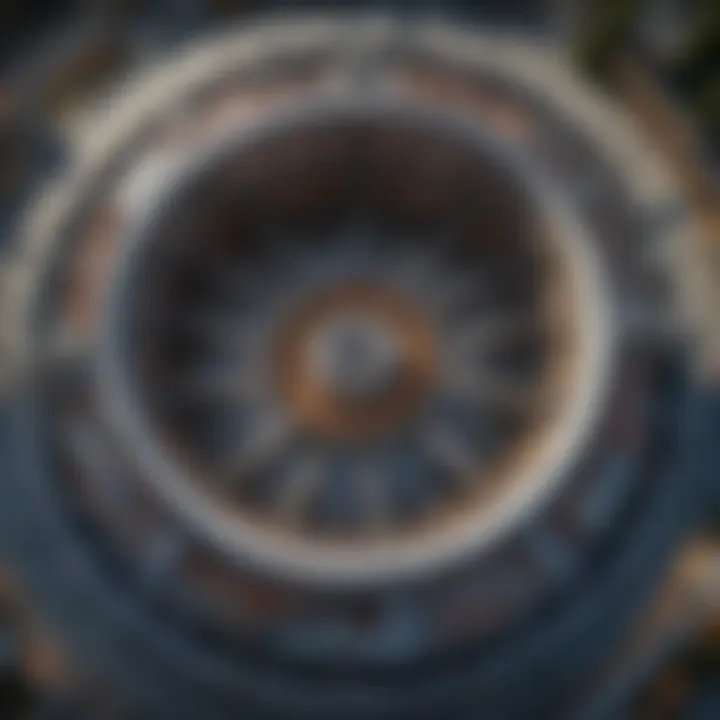
Intro
The Large Hadron Collider (LHC) is a marvel of modern engineering and a cornerstone of particle physics research. It occupies a circular complex that spans the border between Switzerland and France, showcasing the collaboration of many countries and institutions. With a circumference of 27 kilometers, the LHC is designed to collide particles at unprecedented energies, allowing scientists to probe the fundamental building blocks of matter and the forces that govern their interactions.
Such a facility enables the exploration of phenomena that cannot be studied through traditional experimental methods. As researchers look into the subatomic realm, the LHC acts as a critical tool, driving forward questions related to the universe's origins, structure, and ultimate fate. This significant research endeavor is more than just machinery; it represents centuries of scientific thought and collaboration aimed at unraveling the mysteries of our universe.
Prelude to the Hadron Collider
The Large Hadron Collider (LHC) represents a landmark in the field of particle physics. Understanding its significance requires a grasp of its historical evolution, purpose, and impact on modern scientific inquiry. The LHC is not just a machine; it is a culmination of decades of research, international collaboration, and engineering expertise, which together push the boundaries of what we know about the universe at its most fundamental levels.
As we explore the LHC, it becomes evident that this giant accelerator allows scientists to recreate conditions similar to those just after the Big Bang. With such capability, the LHC stands as a vital tool for probing the intricate world of subatomic particles.
This section will unfold the historical context that led to the development of the LHC, as well as its defining purpose in contemporary science. By examining both aspects, we can appreciate the comprehensive framework within which the LHC operates and its ongoing contributions to the understanding of cosmic mysteries.
Historical Context
The roots of the LHC can be traced back to the mid-20th century when physicists began to harness the power of particle accelerators. These devices escalated in complexity, culminating in the creation of colliders that enabled high-energy collisions at unprecedented scales. The successful operation of the Super Proton Synchrotron (SPS) at CERN in the 1980s laid foundational groundwork, acting as a precursor to the LHC’s eventual construction.
The LHC was officially inaugurated in 2008, representing years of effort and collaboration among scientists from over 100 countries. It was built with the ideal of addressing fundamental questions that arise in physics, such as the nature of mass, the existence of dark matter, and the fabric of space-time itself. Key contributors to this project included not just physicists, but also engineers, technicians, and administrators who navigated complex logistical and financial challenges to bring the LHC to life.
Definition and Purpose
The term "Hadron Collider" refers to a particle accelerator designed specifically for colliding hadrons, which are particles like protons and neutrons. The primary purpose of the LHC is to propel protons to near-light speeds and collide them, allowing researchers to observe the resulting interactions and decay products. This process is crucial for understanding the forces at play in the universe and the composition of matter.
Apart from its role in particle collisions, the LHC also serves a broader purpose in the scientific community. It is a platform for international collaboration, facilitating the sharing of data and research outcomes. Furthermore, the knowledge generated from the LHC has implications that extend beyond physics itself, affecting fields such as engineering, computing, and medicine.
In summary, the introduction of the Large Hadron Collider is not merely about a machine. It is about how that machine feeds into our shared quest for knowledge. With the historical context and fundamental purpose of the LHC established, we are better positioned to delve deeper into its inner workings and the ramifications of its discoveries.
CERN: The Driving Force
CERN, the European Organization for Nuclear Research, plays a crucial role in the operation and success of the Large Hadron Collider (LHC). It is more than just a research facility; it is a scientific community that enables researchers from around the globe to explore the fundamental components of matter. The significance of CERN extends beyond its physical structure. It serves as a hub for collaboration, innovation, and groundbreaking discoveries in high-energy physics, shaping our current understanding of the universe.
CERN's influence is notable in multiple layers:
- Innovative Research: CERN is home to numerous experiments that have transformed physics. The LHC, for instance, has provided insights into the Higgs boson, a particle that explains why other particles have mass.
- Global Collaboration: Scientists from varied backgrounds converge at CERN, fostering an international spirit that enhances scientific inquiry.
- Educational Outreach: CERN implements programs that educate and inspire students and educators in the field of particle physics, ensuring future generations can continue the inquiry into fundamental questions.
Understanding CERN's background and its funding mechanisms helps appreciate its substantial impact on science and technology.
Background of CERN
CERN was founded in 1954, emerging from post-war collaborations among European nations. Its establishment marked a foundation for peace through scientific research. The facility began with modest goals but has expanded capabilities over the decades, developing into the world's largest particle physics laboratory.
The organization's location near Geneva, Switzerland, offers a strategic position for collaboration between various nations, nurturing partnerships among member countries. Today, CERN is supported by 23 member states, and its projects engage thousands of scientists, engineers, and technicians.
Overall, CERN has become synonymous with particle physics, paving the way for several significant discoveries that have changed our understanding of the universe.
Funding and Collaboration
CERN operates under a unique financial model that relies heavily on the contributions of its member states. Each member shares the cost proportional to its gross national product. This shared funding model not only enhances cooperation but also ensures an equal stake in the pursuit of knowledge.
- Budget Allocations: The financial commitment from member states is essential. CERN’s annual budget reflects its ambition, with funds allocated to support operational costs, experimental setups, and research initiatives.
- International Partnerships: Collaboration is a cornerstone of CERN’s ethos. Partnerships with universities, research institutions, and private organizations bolster the research capabilities. These collaborations allow for resource sharing, knowledge exchange, and widespread engagement in scientific discoveries.
The collaborative spirit at CERN highlights the importance of international cooperation in advancing scientific frontiers.
Through its strategic funding and collaborative framework, CERN not only facilitates extraordinary research but also reinforces the global scientific community's shared responsibility toward understanding the fundamental laws of nature.
Design and Structure of the LHC
The design and structure of the Large Hadron Collider (LHC) play a crucial role in its overall functionality and effectiveness in particle physics research. Understanding these elements deepens our appreciation of its significant contributions and the challenges it faces. The LHC is not merely a ring; it is a complex assembly of various systems working in concert to facilitate high-energy physics experiments. Its sophisticated design enhances collision rates and enables precise measurements, which are vital for the scientific objectives it aims to achieve.
Engineering Marvels
The LHC’s engineering is a testament to modern science and technology. One of its most remarkable features is its circumference of approximately 27 kilometers, making it the largest particle accelerator in the world. This extensive length allows for multiple acceleration stages. Protons are accelerated to speeds close to the speed of light before colliding. The precision required in engineering components such as magnets and detectors cannot be overstated.
In total, the LHC uses over 1,200 superconducting magnets, which reach temperatures near absolute zero. The magnet systems are integral for steering and focusing the particle beams. Maintaining such low temperatures is a significant engineering challenge. It requires advanced cooling technologies to ensure superfluid helium is continuously circulated.
Moreover, the detectors are meticulously designed to capture collision data without losing critical information. For instance, the ATLAS and CMS detectors are massive, each weighing thousands of tons and consisting of several layers of sophisticated technology that can analyze the particles produced in collisions with exceptional accuracy.
"The intricacies of the LHC’s design illustrate the depths of human ingenuity and the collaborative spirit of international science."
Technical Specifications
The technical specifications of the LHC reflect its ambitious goals. Powered by a 600 megawatt electrical system, it can achieve particle collision energies exceeding 14 tera-electronvolts (TeV). This energy range is essential for probing fundamental questions about the universe, including the nature of mass and the existence of dark matter.
The LHC operates under a vacuum, allowing particles to travel unimpeded by air resistance. This vacuum is maintained with an impressive technology that ensures the environment is optimal for particle beams. To minimize interference, all components, including the detectors, are designed with redundancy in mind.
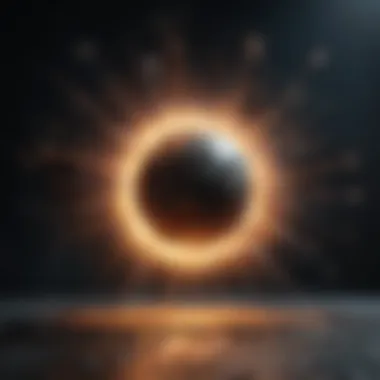
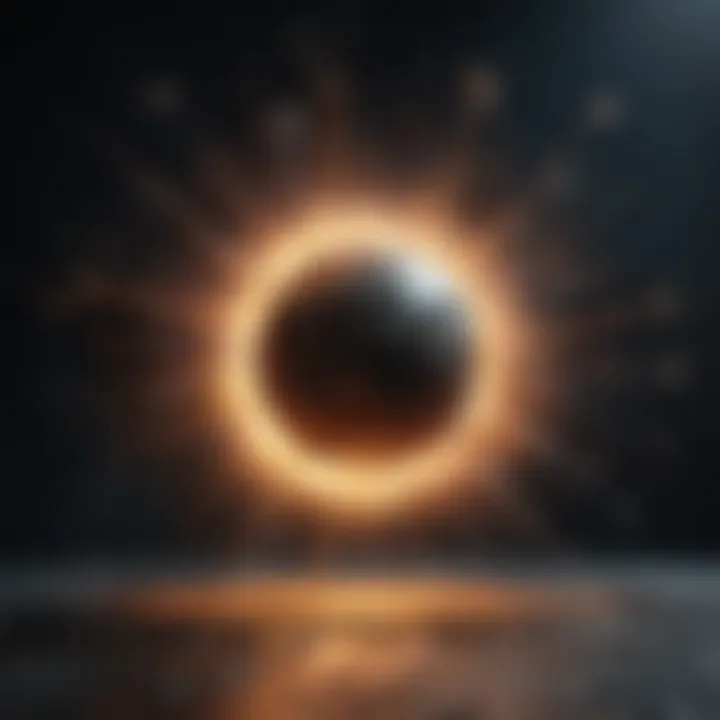
Furthermore, safety mechanisms are in place to manage potential risks associated with high-energy collisions. Users of the LHC can rely on layered safety protocols to ensure that it operates within considered parameters, thus minimizing unforeseen consequences of its operation.
The combination of robust engineering and meticulous specifications enables the LHC to provide invaluable data for research in particle physics, contributing to our understanding of the universe.
How the LHC Works
The operation of the Large Hadron Collider is intricate yet fundamental to its purpose. Understanding how the LHC works requires a breakdown of three core mechanisms: particle acceleration, collision events, and detection techniques. These components are paramount when discussing the collider’s role in advancing particle physics.
Particle Acceleration
Particle acceleration is the first crucial step in the LHC's operational process. The accelerator relies on powerful electromagnets to propel protons and ions to near light speeds. This setup is essential to achieving the energy levels needed for significant collisions, which are crucial for probing the fundamental nature of matter.
The process begins with a source that generates hydrogen ions. These ions are then stripped of their electrons, resulting in pure protons. Once produced, protons enter the linear accelerator, known as LINAC2. Here, they gain initial energy. Following this, the protons move into the Proton Synchrotron and subsequently the Super Proton Synchrotron. Each phase of this acceleration increases their energy and speed.
The LHC itself consists of a 27-kilometer ring located underground, filled with superconducting magnets that guide the protons along their circular path. The magnets need to be kept at extremely low temperatures, approximately -271.3 degrees Celsius, to maintain their superconductive state, which is vital for efficient acceleration.
Collision Events
Collision events are the next step that brings extensive data collection and scientific inquiry. Once the protons are accelerated to the desired energy levels, they travel in opposite directions within the collider, colliding at specific points throughout the ring. These collisions occur around 40 million times per second, generating an extraordinary level of energy that creates conditions similar to those just after the Big Bang.
Each collision produces a variety of particles, many of which are fleeting and exist for only an instant. The study of these collisions helps scientists look deeper into the structure of matter and forces of nature. Through high-energy collisions, researchers can explore phenomena such as the production of the Higgs boson, which is central to our understanding of mass in the universe.
Detection Techniques
After collisions occur, detection techniques become vital to capturing and interpreting the data generated. The LHC is equipped with large detectors, notably ATLAS and CMS, that surround the collision points. These detectors are composed of various sensors and layers that track, measure, and record the multitude of particles produced in each event.
The detection process involves several methods. Use of specialized electronics gathers signals produced by charged particles as they traverse the detector materials. These signals are then processed using sophisticated algorithms to reconstruct the paths and energies of the particles.
Data analysis from the LHC often requires remarkable computational power due to the sheer scale of information collected. Each collision produces an enormity of data points, which must be filtered and understood in context. Thus, advanced statistical methods and powerful computational resources are in demand to derive meaningful interpretations from the results.
Understanding how the LHC works is crucial for comprehending its contributions to the field of particle physics and the nature of the universe.
In summary, the functioning of the LHC encompasses a cycle of acceleration, collision, and detection. Each step is critical in ensuring that the collider can fulfill its ultimate purpose: unraveling the mysteries of matter and the universe.
Scientific Objectives of the LHC
The Large Hadron Collider (LHC) is not merely an impressive feat of engineering; it is fundamentally a tool for addressing some of the deepest questions in modern physics. Understanding the scientific objectives of the LHC allows us to appreciate its significance in the broader context of particle physics.
The objectives primarily revolve around probing the fundamental constituents of matter, exploring the forces that govern how particles interact, and elucidating phenomena that shape the universe. By focusing on these themes, the LHC aims to unravel mysteries such as the existence of the Higgs boson, the nature of dark matter, and the framework of supersymmetry.
Search for the Higgs Boson
The search for the Higgs boson is one of the most celebrated objectives of the LHC. Postulated in the 1960s, the Higgs mechanism is crucial for explaining why particles acquire mass. Before the LHC began operation, the Higgs boson was the last unobserved particle predicted by the Standard Model of particle physics.
The discovery of the Higgs boson in 2012 confirmed decades of theoretical work. It provided evidence for the mechanism that gives mass to elementary particles. This finding not only validated the Standard Model but also opened new avenues for interpreting the universe’s mass structure.
Investigation of Dark Matter
Dark matter poses one of the greatest enigmas in modern physics. Comprising about 27% of the universe's mass-energy content, it does not emit, absorb, or reflect any forms of electromagnetic radiation, which makes it incredibly elusive. The LHC seeks to create conditions that could produce dark matter particles through high-energy collisions.
Identifying dark matter candidates and determining their properties could reshape our understanding of the universe and its evolution. This aspect of LHC research underscores its role in bridging gaps in current knowledge and enhancing our grasp of cosmic phenomena.
Exploring Supersymmetry
Supersymmetry is a theoretical framework that proposes a relationship between two basic classes of particles: bosons and fermions. If confirmed, it would provide answers to several nagging questions in physics, including the hierarchy problem and the nature of dark matter. The LHC conducts experiments specifically aimed at detecting supersymmetric particles.
Since experiments at the LHC can reach unprecedented energy levels, they allow physicists to probe beyond the Standard Model, searching for signs of supersymmetry. This could lead to revolutionary developments in particle physics and a deeper understanding of the universe.
"The scientific objectives of the LHC are not just steps towards discovery; they are milestones in humanity's understanding of the universe."
In sum, the scientific objectives of the LHC are critical for advancing our comprehension of the fundamental aspects of nature. Each aspect, from the search for the Higgs boson to the investigation of dark matter and exploring supersymmetry, contributes not only to particle physics but also to our philosophical and practical understanding of the cosmos.
Major Discoveries
The Large Hadron Collider has truly transformed our understanding of the universe. It has been the site of groundbreaking discoveries, reshaping fundamental physics. These major discoveries not only validate theoretical models but also pave the way for new avenues of research. Analyzing these findings provides insights into the nature of matter, forces, and the cosmos. This section will delve into two significant revelations from the LHC: the Higgs Boson discovery and other noteworthy findings that have emerged over the years.
Higgs Boson Discovery
The discovery of the Higgs Boson in July 2012 was a landmark event in particle physics. This particle was crucial for providing an explanation for how particles gain mass. Prior to its discovery, the existence of the Higgs field was a theoretical concept. Scientists predicted it through the Standard Model of particle physics, but it remained elusive for decades.
When researchers at CERN's ATLAS and CMS experiments confirmed the Higgs Boson, it was as if a puzzle piece in the picture of fundamental physics had finally been placed. The results from the LHC demonstrated the particle’s mass at about 125 giga-electron volts, which was consistent with theoretical predictions. This discovery was vital for several reasons:
- Validation of the Standard Model: It confirmed a central tenet of the Standard Model, thereby consolidating the framework that has dominated particle physics.
- Understanding of Mass: By shedding light on the mechanism that gives mass to elementary particles, it courageously deepens our understanding of the universe's structure.
- Stimulus for New Theories: The existence of the Higgs Boson opens pathways for exploring beyond the Standard Model, which includes potential new physics.
As physicists continue to study the properties of the Higgs Boson, its implications are expected to resonate through the realms of theory and experimentation alike.
Other Significant Findings
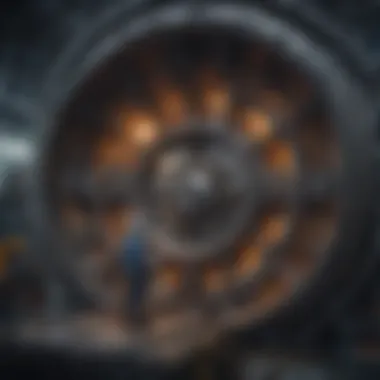
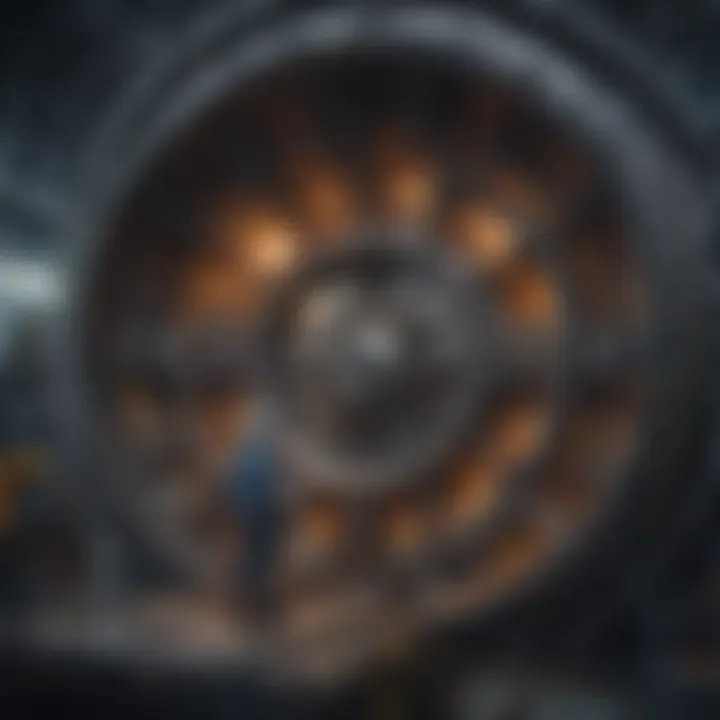
Beyond the Higgs Boson, the LHC has yielded discoveries that contribute to our broader understanding of particle physics and cosmology. Here are some noteworthy findings:
- Top Quark Measurements: Precision measurements of the top quark, the heaviest known elementary particle, have refined theoretical models.
- Search for Supersymmetry: While no direct evidence has been found for supersymmetry, searches for these hypothesized particles continue, suggesting a rich landscape yet to be understood.
- B-meson Decay Observations: Studies involving B-mesons have provided evidence for the violation of charge-parity symmetry, challenging existing theories and presenting a new framework for understanding the universe.
These discoveries, along with dozens of others, showcase the LHC's role as a premier facility for high-energy physics research. Each finding not only reinforces existing knowledge but also serves as a foundation for new explorations into the fabric of reality.
"The discoveries made at the LHC have radically shifted our perceptions of physics. It helps scientists explore the unknown, revealing layers of complexity in our universe."
Future research at the LHC will likely lead to even more significant discoveries, expanding both our knowledge and the frontiers of physics.
Data Analysis Techniques
The execution of experiments at the Large Hadron Collider is not only about creating high-energy collisions but also effectively analyzing the vast amounts of data generated from these interactions. Understanding Data Analysis Techniques is crucial for extracting meaningful insights and discovering fundamental truths about particle physics. This section discusses two of the key elements of data analysis in the context of the LHC: computational challenges and statistical methods.
Computational Challenges
At a fundamental level, the computational challenges associated with LHC data analysis are immense. The LHC generates up to 40 million proton-proton collisions every second, which results in an overwhelming volume of information. Processing and storing this data requires advanced storage solutions, sophisticated algorithms, and immensely powerful computing resources.
The first aspect of these challenges is the sheer size of the datasets. The LHC has been producing data since it began operation in 2008. Managing petabytes of information requires distributed computing systems. To cope with this data flood, CERN developed the Worldwide LHC Computing Grid (WLCG), a global collaboration that joins hundreds of computing centers around the world. This grid enables researchers to share data and computing resources, maximizing processing efficiency.
Moreover, many events recorded may not be relevant for the analysis. Event selection or triggering is thus a critical part of the process. Using sophisticated algorithms, scientists filter out unimportant events before performing detailed analysis. This step is vital for ensuring that only the most valuable data informs the research conclusions. Ultimately, addressing these computational challenges is foundational for maintaining the integrity and effectiveness of the LHC's discoveries.
Statistical Methods
Statistical methods are equally essential in the realm of data analysis at the LHC. Raw data undergoes rigorous statistical scrutiny to distinguish significant results from background noise. Detecting rare events, such as Higgs boson production, demands robust statistical techniques to ensure that findings are not merely accidental or due to statistical fluctuations.
One of the primary techniques utilized is the hypothesis testing framework. Scientists often establish a null hypothesis, representing the absence of a particular phenomenon. By gathering data and applying statistical tests, researchers assess the likelihood of observing the collected data under the null hypothesis. This method provides a clear framework for discerning significant particles from random occurrences.
Another important statistical method involves various significance measures, particularly the p-value. A low p-value, typically below 0.05, suggests that the observed data would be highly unlikely if the null hypothesis were true. Likewise, confidence intervals help quantify uncertainty and give insight into how reliable the results are.
The integration of statistical techniques in the analysis process allows physicists to validate findings, iterating upon them through multiple methods. In summary, the intricate and advanced data analysis techniques employed at the LHC are indispensable to realizing its scientific objectives.
The ability to analyze data effectively determines the success of discoveries in particle physics, highlighting the complexity and collaborative nature of modern scientific research.
Impact on Physics and Cosmology
The Large Hadron Collider, known as LHC, has had a profound impact on physics and cosmology. This section details the significance of its contributions and the implications for our understanding of the universe. The LHC is not just a research facility; it has become a cornerstone for exploring fundamental questions about matter, energy, and the forces that govern the cosmos.
Advancements in Our Understanding of the Universe
The experiments facilitated by the LHC have advanced our knowledge of the fundamental building blocks of nature. Discoveries such as the Higgs boson have clarified the mechanisms that give rise to mass. This insight has allowed physicists to piece together how particles interact within the framework of the standard model of particle physics.
Moreover, research conducted at the LHC has opened new avenues into the mysteries of dark matter and dark energy. Detection of rare particle interactions provides clues to the unseen part of the universe. It pushes the boundaries of what we understand about the cosmos. This has prompted significant discussions in both theoretical and experimental physics.
Additional findings have also challenged existing theories. For example, the potential discovery of supersymmetric particles could reshape our understanding of particle interactions. Each step forward yields new questions, revealing the intricacies of physical laws and their implications for the universe at large.
Implications for Future Research
The findings from LHC experiments have far-reaching implications for future research. Firstly, the success of high-energy collisions has fueled interest in further upgrades to the collider itself. Proposed enhancements aim to explore energies beyond current levels, which may uncover phenomena not yet observed.
Secondly, the collaboration fostered among international researchers sets a precedent for addressing complex scientific problems. Joint projects enable pooling resources and expertise, enhancing the quality of research output. The shift towards open data collaborations is creating a more inclusive research environment, allowing wider access to results and methodologies.
Lastly, the results from the LHC may lead to the development of new theoretical frameworks. These frameworks can better explain the anomalies and phenomena observed in particles. The role of computational techniques will likewise grow, allowing scientists to model and simulate scenarios that test existing theories and explore new possibilities.
"The LHC is a tool not just for discovery, but for forging new paths in our understanding of the universe."
Ultimately, the LHC’s impact reverberates beyond particle physics, influencing cosmology, technology, and even philosophy. The implications of its findings will likely guide scientific inquiry for decades to come. The quest for knowledge will continue, fueled by the boundaries pushed by this remarkable facility.
International Collaboration and Community
The success of the Large Hadron Collider (LHC) at CERN is not solely attributed to its advanced technology or theoretical foundations. A crucial element influencing its progress is the extensive international collaboration that enables it to function effectively. The LHC stands as a testament to what can be achieved when a diverse group of scientists, engineers, and institutions unite towards a common goal. Through collaboration, research can be enriched by varying perspectives and expertise, enhancing the overall scientific output.
Global Partnerships in Research
Collaboration at the LHC stretches across boundaries, with contributions from institutions worldwide. This partnership facilitates a continuous exchange of knowledge and technical skills. Countries such as the United States, Japan, and various European nations have had significant input in the LHC's construction and operation. The combined resources from these diverse collaborations result in not only financial support but also a rich pool of ideas.
The direct benefits of these alliances are manifold:
- Shared Resources: Access to specialized equipment, facilities, and funding.
- Expertise Exchange: Scientists from diverse backgrounds bring unique insights that can lead to innovative approaches in research.
- Broader Impact: Enhanced visibility for findings due to wider dissemination through international channels.
"Collaboration is essential for breaking down the barriers in science," noted a CERN physicist during a recent seminar. This statement underscores the collective effort placed in unraveling the complexities of particle physics.
Role of Scientists and Engineers
At the core of the LHC's endeavors are the scientists and engineers who bring their specialized skills to the project. Their role extends beyond mere participation; they are the driving force behind the innovation and problem-solving that is necessary in such a monumental project.
- Scientists are primarily focused on formulating hypotheses and conducting experimental research. They analyze the data produced by collision events, utilizing advanced computational techniques to extract meaningful results. Their work often leads to new theories or enhancements to existing models in physics.
- Engineers ensure that the LHC's infrastructure operates smoothly. They are involved in the design, maintenance, and optimization of the collider's components. From superconducting magnets to data acquisition systems, their expertise is vital for the LHC's functionality.
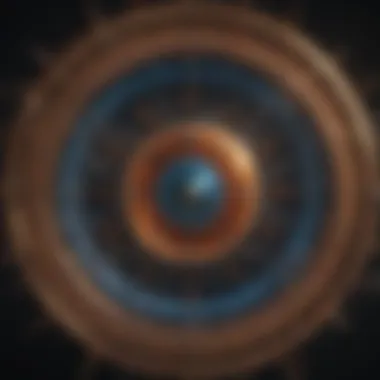
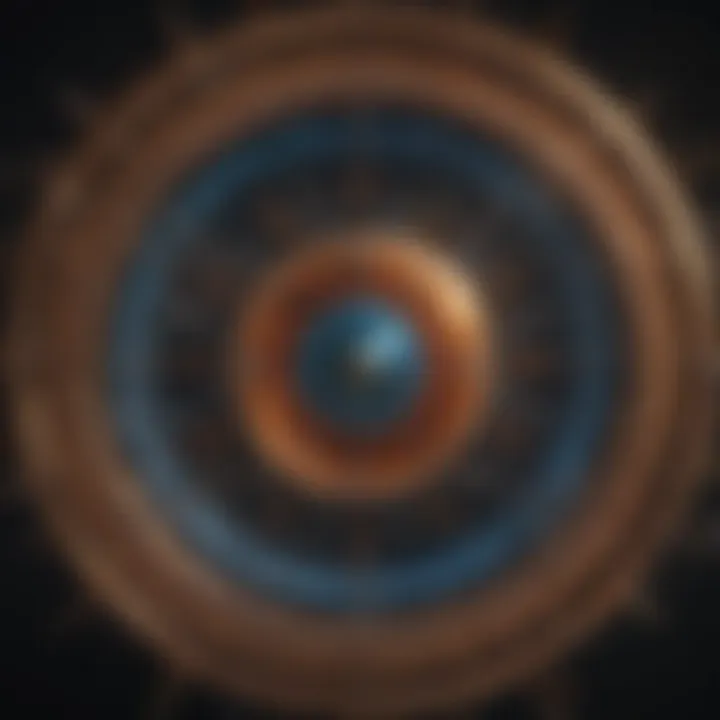
This partnership between scientists and engineers promotes a dynamic environment where challenges can be tackled effectively. As technology evolves, their collaboration must adapt, leading to constant improvements in methodologies and operational techniques.
By fostering international partnerships and recognizing the vital role of its personnel, the LHC continues to thrive as a world-leading facility in the field of particle physics.
Funding and Economic Aspects
Funding and economic aspects are critical to the sustainability and progress of large-scale scientific projects like the Large Hadron Collider (LHC) at CERN. Understanding how financial resources are allocated and how they translate into scientific advancements provides valuable insight into the operations of such projects. The financial framework ensures that necessary resources are available for research and innovation while also balancing public and institutional interests.
Budget Allocations
Effective budget allocation is a cornerstone of the LHC's operation. CERN’s operational budget must be managed efficiently to cover the costs involved in maintenance, upgrades, and ongoing research initiatives. This budget typically includes expenses such as:
- Facility Maintenance: Regular maintenance is crucial to ensure the collider operates at optimum levels.
- Research Funding: Allocations support various research teams working on distinct projects.
- Infrastructure Development: Investment in infrastructure is necessary for future upgrades and expansions.
- Personnel Costs: Funding is required for a large team of scientists, engineers, and support staff.
Decisions on budget allocations are made collectively by the member countries of CERN, which include over 20 nations. Countries contribute based on their Gross National Product (GNP), distributing the financial burden equitably. Adjustments in the budget occur as needed to align with new scientific goals or unforeseen challenges, ensuring that funding remains responsive and effective.
Return on Investment in Scientific Research
The return on investment (ROI) from scientific research in particle physics, particularly from the LHC, is not measured purely in financial terms. While significant projects like these require hefty investments, the yields can be assessed in various forms. Recognized benefits include:
- Scientific Knowledge: Major discoveries, such as the Higgs boson, enhance our understanding of fundamental particles and forces, which can lead to more significant advancements in physics.
- Technological Innovations: The technological developments emerging from the LHC have widespread applications in fields beyond physics, including medicine, computing, and environmental science.
- Educational Impact: Investment in the LHC provides numerous educational opportunities, fostering the next generation of scientists and engineers.
- International Collaboration: The LHC is a hub for global scientific collaboration, demonstrating how diverse entities can work together to address complex scientific challenges.
"The return on investment from scientific research often transcends monetary assessment, reflecting in broader societal advancements."
For more information on the financial structure of CERN and its projects, you can explore resources like CERN's official site or details on funding strategies from Wikipedia.
Future of the LHC
The future of the Large Hadron Collider (LHC) is crucial for understanding the landscape of modern physics. As the world's most powerful particle accelerator, the LHC has already made significant discoveries, including the Higgs boson. However, the journey does not end here. Continuous improvements and future initiatives will allow physicists to probe deeper into the fundamental nature of matter and the universe.
Upgrades and Extensions
Upgrades to the LHC are imperative to enhance its capabilities and expand its scientific reach. CERN is planning several significant upgrades to the accelerator. One of the key upgrades is the High-Luminosity LHC (HL-LHC) project, which aims to increase the luminosity of the collider by a factor of ten. This increase will enable more collision data to be gathered, thereby allowing experiments to probe rarer processes.
Additionally, technological innovations will be introduced, such as advanced superconducting magnets and improved detector systems. These upgrades will optimize performance and enhance data collection efficiency. Enhanced capabilities could lead to discoveries that are currently beyond our reach. The investment in these upgrades demonstrates a commitment to maintaining the LHC's status as a leading facility for particle physics research.
Long-Term Scientific Goals
The long-term scientific goals of the LHC extend beyond immediate discoveries. Researchers aim to explore fundamental questions about the universe. Some of these goals include:
- Investigating New Physics: The LHC still holds potential for discovering new particles, possibly shedding light on dark matter or extra dimensions.
- Studying the Higgs Field: Further exploration of the Higgs boson could reveal new insights into the mechanism of mass generation and the stability of the universe.
- Testing Supersymmetry: The LHC will persist in testing theories such as supersymmetry, providing potential candidates for new particles that may explain current anomalies in particle physics.
- Understanding Matter-Antimatter Asymmetry: Investigations into why the universe is predominantly made of matter rather than antimatter remain a critical goal.
Ethical and Philosophical Considerations
The exploration of ethical and philosophical considerations in the context of the Large Hadron Collider (LHC) is of paramount importance. As with any major scientific endeavor, ethical questions arise regarding safety, societal implications, and the philosophical underpinnings of scientific inquiry. These topics not only inform public discourse but also shape the future of research in high-energy physics, ensuring that scientific progress aligns with societal values.
Safety Concerns
Safety is a prominent concern connected to the LHC. It involves both the physical safety of scientists and the broader implications of the experiments conducted.
- Accidents and Hazards: The large-scale nature of collider experiments raises fears of accidents, though extensive safety protocols are in place. The Collider has been thoroughly analyzed to prevent catastrophic failures.
- Environmental Impact: The LHC consumes vast amounts of energy. This raises questions about its carbon footprint and environmental effects, prompting discussions about sustainable scientific practices.
- Potential for Unexpected Phenomena: There have been speculative concerns regarding the creation of mini black holes or strange matter. While expert consensus assures that these scenarios are improbable, the very nature of high-energy experiments invokes public anxiety and scrutiny.
“The intersection of complex science and public apprehension necessitates transparent communication about risks and benefits.”
Through ongoing safety assessments and public outreach, CERN aims to alleviate concerns and foster trust in scientific progress.
Philosophy of Science and Research
The philosophy of science encompasses the foundational questions about knowledge and inquiry. In the context of the LHC, several philosophical issues merit consideration.
- Nature of Scientific Knowledge: What constitutes valid knowledge in particle physics? The LHC challenges traditional paradigms by seeking to uncover truths about matter and the universe at fundamental levels.
- Reductionism vs. Holism: There is a debate on whether understanding particle interactions directly leads to profound insights about the universe as a whole or if other, broader perspectives are required.
- Role of Ethics in Science: The ethical responsibilities of scientists extend beyond their immediate research. They are expected to consider how their findings impact society, ethics of funding, and implications of knowledge gained.
The LHC represents not just a technological triumph but also a philosophical endeavor that reflects humanity's quest to understand its place in the cosmos. The discussions surrounding its ethical implications serve as a reminder that science does not exist in a vacuum, and moral considerations are essential in shaping research agendas.
Engagement with these ethical and philosophical aspects assures that the LHC and similar projects proceed in a manner that is cognizant of their broader societal impact.
Culmination
The conclusion of this article encapsulates the journey through the intricacies of the Large Hadron Collider (LHC) and its unparalleled significance in the realm of particle physics. It synthesizes the essential findings, portraying how this monumental instrument stands as a beacon of scientific progress, illuminating the path toward deeper understanding of the universe.
Summary of Key Findings
Throughout the exploration of the LHC, several key insights stand out:
- Higgs Boson Discovery: The identification of the Higgs boson has profound implications for the Standard Model of particle physics. It confirmed the mechanism that gives mass to elementary particles, validating long-held theories.
- Dark Matter Investigation: The LHC's ability to generate high-energy collisions has opened avenues to probe the nature of dark matter, a mysterious component that constitutes a vast majority of the universe's mass.
- Global Collaboration: The international effort at CERN exemplifies the power of global research partnerships. Thousands of scientists and engineers from around the world contribute their expertise to unravel the complexities of fundamental particles.
- Technological Innovations: The engineering feats achieved in building and operating the LHC have pushed technological boundaries, resulting in advances that benefit various fields, including medical imaging and data processing.
The Road Ahead for Particle Physics
The future of particle physics, particularly in relation to the LHC, is both promising and challenging. Several factors will shape this trajectory:
- Upgrades to the LHC: Upcoming enhancements, such as the High Luminosity LHC, aim to increase the collision rate, which will produce more data and potentially uncover new physics.
- New Experiments and Facilities: Future projects like the Future Circular Collider (FCC) may follow the LHC, potentially allowing exploration of energy scales beyond the current capabilities.
- Theoretical Developments: Research continues on theories like supersymmetry or string theory. These concepts may find validation or refutation through experiments conducted at the LHC and other facilities.
Exploring the LHC is not merely a scientific endeavor but a reflection of humanity's pursuit of knowledge. The impact of its discoveries resonates beyond particle physics, offering profound implications for our understanding of the universe. The path ahead is laden with potential, and as researchers build upon the findings of the LHC, the realm of possibilities seems limitless.