Exploring the Chemical Structure of Titanium Dioxide
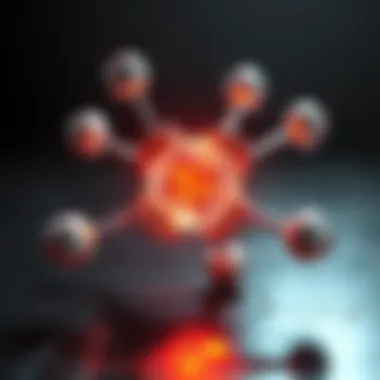
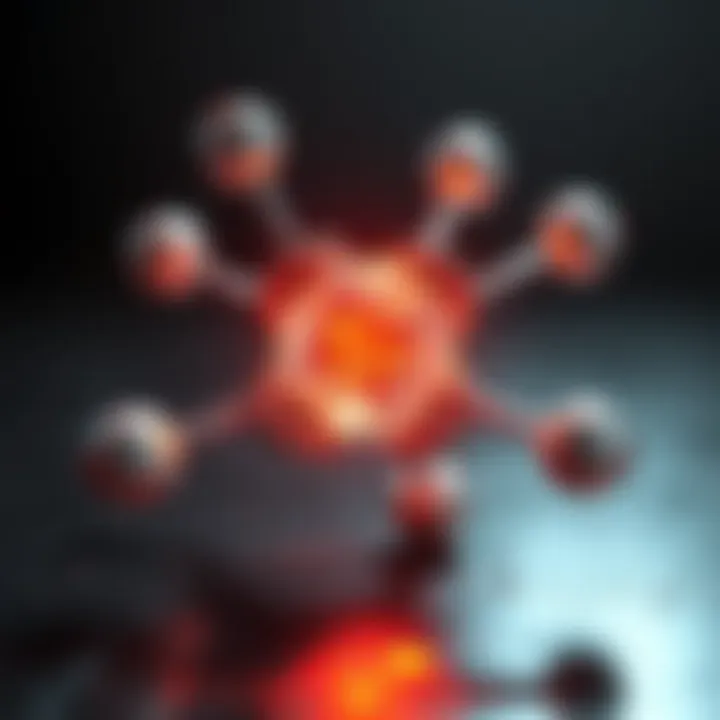
Intro
The chemical landscape is filled with compounds that shape our lives in numerous ways. Among these, titanium dioxide emerges as a versatile yet often overlooked substance. It's prevalent in many industries, owing to its remarkable properties and safe profile. Understanding its chemical structure isn't just an academic exercise; it unearths the reasons behind its widespread usage and its behaviors in various environments. This exploration will shed light not only on the makeup of titanium dioxide but also on its pivotal role in practical applications across the globe.
Overview of Research Topic
Brief Background and Context
Titanium dioxide, also known as TiO₂, is a compound that has sparked the interest of scientists and engineers alike. It garners attention for its ability to scatter light, making it a critical ingredient in paints, coatings, and even sunscreens. Found naturally in minerals like rutile and anatase, titanium dioxide is prized for its high refractive index and non-toxicity.
The structural nuances of titanium dioxide demonstrate a fascinating interplay of the titanium atoms with oxygen. This relationship underpins many of its unique properties, such as its effectiveness as a photocatalyst and its application in environmental remediation technologies.
Importance in Current Scientific Landscape
In today's scientific discourse, titanium dioxide represents a bridge between everyday consumer products and advanced technological applications. From enhancing the brightness of paint to its role in photocatalysis for wastewater treatment, the implications of studying its chemical structure are vast. Researchers are continuously probing its ability to interact with the environment—especially regarding its photocatalytic properties that can break down pollutants under UV exposure.
Furthermore, titanium dioxide's impact on nanotechnology and its potential applications in solar cells illustrate how deeply this compound intersects with modern science. As the quest for sustainable and environmentally friendly materials escalates, titanium dioxide's importance cannot be overstated.
“Titanium dioxide has become an integral part of several sectors due to its unique characteristics, and exploring its structure helps us unpack its potential.”
Methodology
Research Design and Approach
Studying the chemical structure of titanium dioxide requires a multi-faceted methodological approach. Using a combination of quantum chemistry, spectroscopy, and crystallography allows researchers to map out its molecular arrangements and bonding interactions accurately. Techniques such as X-ray diffraction and nuclear magnetic resonance play vital roles in elucidating the compound's solid-state structure.
Data Collection Techniques
Gathering data on titanium dioxide includes:
- Spectroscopic Analysis: Involves Infrared (IR) and Raman spectroscopy to understand vibrational modes.
- X-ray Diffraction: Used to determine crystal structures, shedding light on how TiO₂ exists in both rutile and anatase forms.
- Nanostructuring Techniques: Facilitates the physical characterization of the material at the nanoscale, crucial for applications in coatings and composites.
By employing these varied techniques, researchers can paint a more comprehensive picture of titanium dioxide’s molecular architecture, which in turn unlocks its potential in real-world applications.
Prelims to Titanium Dioxide
Titanium dioxide is far from a mere ingredient found at the back of a cosmetics label or in the mix of a paint bucket. Its significance as a chemical compound reverberates through a wide array of industries. This section aims to illuminate the multitude of roles this compound plays, from acting as a mere pigment to a key player in advanced photocatalytic processes.
The importance of titanium dioxide lies not just in its chemical properties but also in its adaptability and effectiveness in various applications. This white powder serves as a fundamental element in products ranging from household paints to high-tech solar cells. Understanding this compound goes beyond recognizing its basic function; it's about grasping its chemical intricacies and how they correlate with real-world applications.
Historical Background
The journey of titanium dioxide dates back to the mid-19th century. Discovered by the British chemist William Gregor in 1791, it was initially found in a mineral called ilmenite. However, the compound really gained traction in the early 20th century, particularly during World War I. At this point, its ability to scatter light and provide brightness caught the attention of industries seeking effective white pigments.
As years rolled on, titanium dioxide's utility blossomed, finding a firm foothold not only in the paint and coatings industry but also in plastics, paper, and textiles. Furthermore, its safety profile made it exceptionally appealing for applications in food products and cosmetics, further embedding the compound into everyday goods.
Contemporary Significance
Fast forward to today, titanium dioxide holds a revered position in various sectors, reflecting its versatility and importance. As a fundamental component in sunscreens, it works by reflecting harmful UV rays, thus providing protection that is both effective and safe for human use.
On the environmental front, titanium dioxide has carved a niche as a photocatalyst. Its utilization in breaking down organic pollutants illustrates its role not only in commercial settings but also in fostering eco-friendlier practices. The increasing concern over sustainable materials and greener technologies places titanium dioxide at the forefront of innovation, especially in developing clean energy solutions.
In sum, titanium dioxide is much more than a simple pigment; it’s a compound that has evolved through time, demonstrating both historical importance and contemporary relevance. Its diverse applications underscore a need for continued research and understanding, as we venture further into an era where its chemical properties can lead to groundbreaking advancements.
Chemical Composition of Titanium Dioxide
The chemical composition of titanium dioxide plays a pivotal role in defining the various properties and applications of this compound. Titanium dioxide, known by its chemical formula TiO₂, consists of titanium and oxygen elements. Understanding this composition reveals insights into its behavior in reports across numerous industries including paints, cosmetics, and environmental applications. The significance of studying the chemical makeup extends from fundamental research to practical applications, highlighting its importance not just in academic settings, but also in real-world implementation.
Elemental Configuration
The elemental configuration of titanium dioxide showcases a simple yet profound arrangement. Titanium, an element classified under the transition metals, has atomic number 22 and an electronic configuration of [Ar] 3d² 4s². This unique configuration allows titanium to form stable bonds with oxygen, leading to the creation of TiO₂. The properties of titanium dioxide can be attributed to its atomic arrangement at the microscopic level.
- Titanium (Ti): A key player with high strength and low density, where its metallic character allows it to participate in various bonding interactions, enhancing the versatility of titanium dioxide.
- Oxygen (O): An essential non-metal, oxygen in TiO₂ forms two stable bonds, resulting in a solid structure that demonstrates durability and function across applications.
This tight-knit configuration results in a compound that possesses several photochemical and catalytic properties, making it beneficial in applications such as sunscreen formulations and photocatalysis.
Oxidation States of Titanium
Examining the oxidation states of titanium is fundamental for grasping the behavior of titanium dioxide in chemical reactions. Titanium can exist in multiple oxidation states, primarily +4 and +3, although it can exhibit other states like +2 and +5 under specific conditions.
- +4 Oxidation State: This is the most stable and common state in TiO₂. The titanium ion in this state has lost all four valence electrons, giving it a positive charge. This stability is crucial in applications requiring durability, such as in paints and coatings.
- +3 Oxidation State: Less stable than Ti(IV), the Ti(III) state often acts as a reducing agent in chemical reactions. This oxidation state plays a role in catalysis, particularly in photochemical reactions where titanium dioxide acts on pollutants.
Understanding these oxidation states not only helps with synthetic approaches but also provides crucial insight into the reactions and transformations titanium dioxide can undergo in environmental contexts.
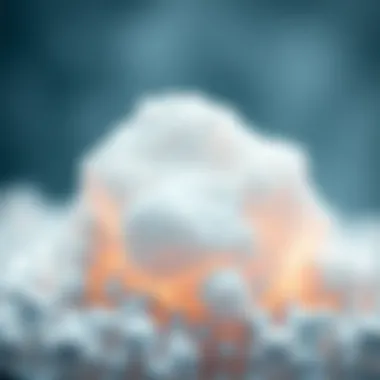
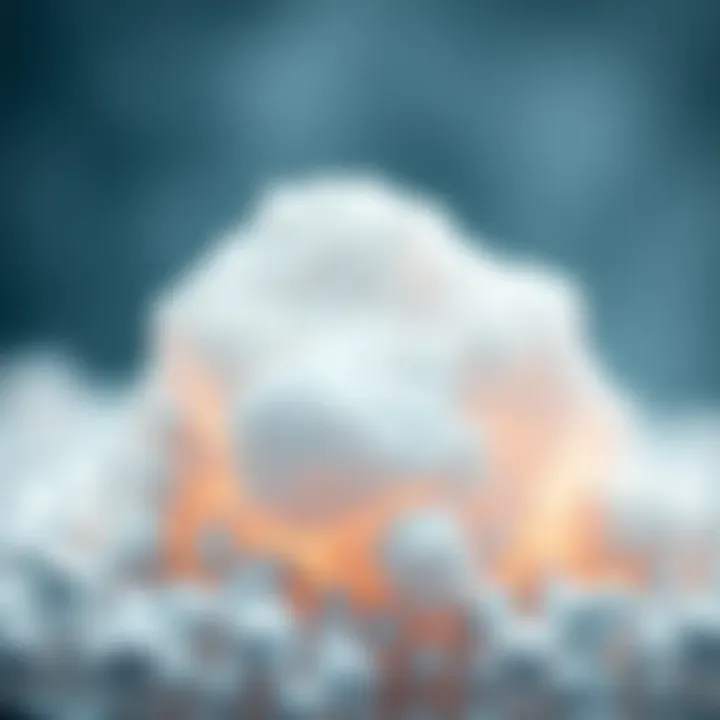
"The oxidation state of an element is often likened to its personality in the chemical world—flipping between states can greatly change its interactions and role in reactions."
In summary, the elemental configuration and oxidation states of titanium dioxide are not just mere academic details; they serve as a foundation for its diverse applications and significance in various industries. By exploring these aspects, we enhance our overall comprehension of its chemical structure and, consequently, its utility in the modern world.
Molecular Structure Analysis
The study of molecular structure is pivotal in grasping the significance of titanium dioxide in various industrial applications. Titanium dioxide exhibits a unique structural composition that influences its physical and chemical properties, making it essential in products ranging from paints to sunscreens. Understanding its molecular arrangement not only sheds light on performance factors but also enables researchers and manufacturers to innovate and enhance formulations.
Crystal Structures of Titanium Dioxide
Titanium dioxide predominantly exists in three crystal forms, each possessing distinct characteristics and functionalities that make them suitable for different applications.
Rutile Phase
The rutile phase of titanium dioxide is perhaps the most well-known and widely used crystal form. This structure is characterized by its tetragonal arrangement, which allows for a high degree of stability.
- Key Characteristic: The high refractive index of rutile makes it a preferred choice for pigments in coatings and plastics, giving these materials bright, opaque colors.
- Unique Feature: Rutile's excellent UV light absorption capacity is another reason for its preference in applications such as sunscreens and protective coatings.
- Advantages/Disadvantages: The primary advantage of rutile is its robust physical and chemical stability. However, its more expensive processing method compared to the anatase phase can be a drawback in some cases.
Anatase Phase
Anatase represents another significant crystal form of titanium dioxide that is often favored for its photocatalytic properties. This structure, also tetragonal yet with different lattice parameters than rutile, is essential in various environmental applications.
- Key Characteristic: Anatase displays higher photocatalytic activity than rutile. This feature makes it particularly valuable in air and water purification processes.
- Unique Feature: Under ultraviolet light, anatase generates reactive oxygen species, enabling it to break down organic pollutants effectively.
- Advantages/Disadvantages: One advantage is its lower cost of synthesis compared to rutile, making it accessible for sustainable applications. However, its lower thermal stability may limit its utility in high-temperature scenarios.
Brookite Phase
Brookite is the least common of the three main crystal structures of titanium dioxide. Its orthorhombic structure lends some unique properties that set it apart from rutile and anatase.
- Key Characteristic: Brookite is recognized for its distinct optical properties, which can be beneficial in some specialized applications like sensors and catalysis.
- Unique Feature: Unlike its more stable counterparts, brookite is often more challenging to synthesize, which can impact its availability for commercial use.
- Advantages/Disadvantages: A significant advantage is its varied reactivity, which can potentially lead to novel applications. However, its instability makes it less appealing for standard commercial uses compared to rutile and anatase.
Bonding Characteristics
The bonding characteristics in titanium dioxide directly influence its chemical behavior and, consequently, its applications in various fields.
Ionic Bonds
The ionic bonds in titanium dioxide arise mainly between titanium and oxygen atoms. This type of bonding contributes to the compound's stability and structural integrity.
- Key Characteristic: The ionic character of these bonds provides titanium dioxide with its high melting point and mechanical strength.
- Unique Feature: The nature of ionic bonding allows titanium dioxide to dissolve in certain alkaline solutions, which can be beneficial for specific processing techniques.
- Advantages/Disadvantages: One clear advantage is the overall stability it confers. However, this stability may limit titanium dioxide's reactivity in some chemical processes.
Covalent Bonds
Covalent bonding in titanium dioxide plays a vital role in its crystal structure and influences its material properties as well. Titanium and oxygen share electrons, contributing to the unique features of the material.
- Key Characteristic: The covalent bonds enhance the electrical properties of titanium dioxide, making it useful in electronic applications, such as semiconductors.
- Unique Feature: The directional nature of covalent bonds can foster specific interactions, affecting how titanium dioxide engages in chemical reactions.
- Advantages/Disadvantages: Covalent bonds elevated stability in various environments, which can be a boon. On the other hand, the covalent nature may limit some dissociative reactions, affecting its performance in photocatalytic applications.
Synthesis Methods
Synthesis methods play an essential role in comprehending the chemical structure of titanium dioxide. The way this compound is created significantly influences its characteristics, purity, and overall performance in various applications. This section outlines both natural sources and synthetic techniques, emphasizing the advantages and considerations tied to each method. Understanding these processes not only sheds light on the material itself but also enhances our grasp of its environmental impacts and utility in different industries.
Natural Sources
Titanium dioxide can be sourced from natural minerals, mainly ilmenite and rutile. Ilmenite is often a heavy, dark mineral found in sand deposits, while rutile presents itself in a red-brown hue. These minerals contain titanium in substantial amounts, typically above 40%. Extracting titanium dioxide from these natural sources involves processes such as roasting and leaching, which converts the minerals into pure titanium dioxide.
One key element of using natural sources is their inherent availability. They provide an eco-friendly alternative compared to synthetic methods. Extracting titanium dioxide from these minerals reduces the need for chemicals often used in lab settings. However, this process tends to be less controlled than synthetic methods, sometimes leading to impurities or inconsistent qualities, which can affect the end products.
Synthetic Techniques
The synthetic pathways to creating titanium dioxide yield high-purity results, which are notably desirable in industrial and technological applications. Several methods stand out:
Sol-Gel Method
The Sol-Gel method is a widely used technique for synthesizing titanium dioxide. In this process, a liquid solution undergoes a transformation into a solid gel phase, allowing precise control over the material's characteristics. One of the prime advantages of the Sol-Gel method is its ability to produce nanoparticles with varied shapes and sizes, making it suitable for a range of applications, from pigments to photocatalysts.
The unique feature of this approach lies in its versatility. Precursors can be mixed in an aqueous or alcoholic medium, leading to different morphological properties. However, one drawback is that the process can be time-consuming and labor-intensive, requiring meticulous control over environmental conditions.
Hydrothermal Synthesis
With Hydrothermal Synthesis, the reaction occurs in an aqueous solution at high temperatures and pressures. This technique allows for crystal growth in a controlled environment, which can result in well-defined titanium dioxide structures, like the anatase phase. This method is valued for producing high crystallinity and uniform particle size.
The key characteristic of hydrothermal synthesis is its effectiveness at altering particle morphologies by adjusting temperature and pressure conditions. Yet, while it offers these advantages, it also demands specialized equipment, which can be a hurdle for some research facilities and labs.
Precipitation Method
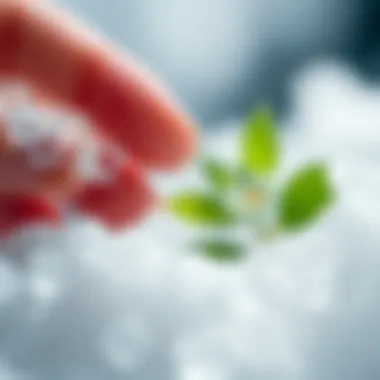
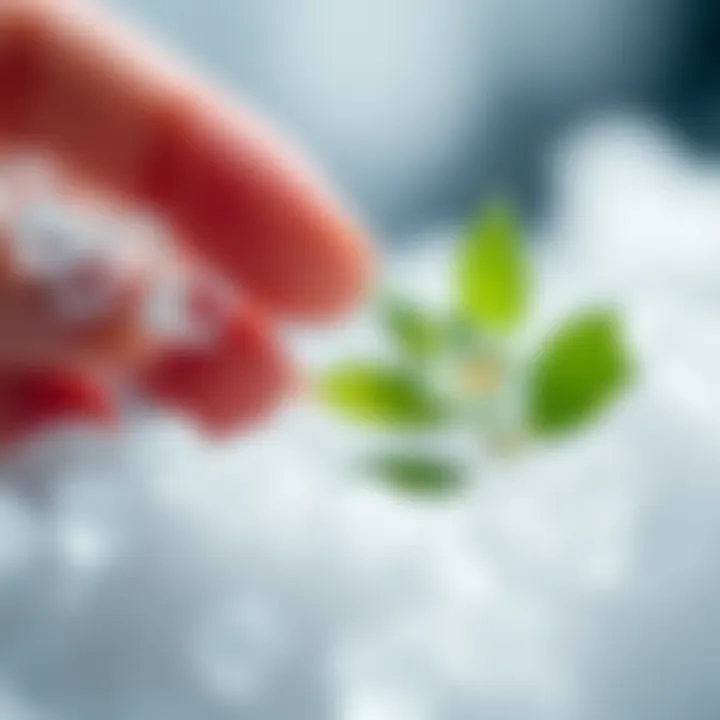
The Precipitation Method is a straightforward and efficient way to synthesize titanium dioxide. In this approach, titanium salts are reacted with alkaline solutions, leading to the formation of titanium dioxide precipitate. This method is appreciated for its scalability and simplicity, often employed in large-scale production environments.
One of the notable features of this method is the minimal equipment required compared to others. This attracts many industries looking for cost-effective solutions. But, like any method, it also has its downsides, such as the potential for impurities due to the raw materials used or incomplete reactions.
"Understanding the synthesis methods of titanium dioxide gives insight into its applications, quality, and environmental impact, ultimately shaping the future of this fundamental compound in technology and industry."
These synthesis methods, both natural and synthetic, provide a comprehensive overview of how titanium dioxide can be produced, each with its unique qualities and trade-offs. Addressing these aspects thoroughly allows researchers and professionals alike to choose the most effective synthesis route for their specific applications.
Properties of Titanium Dioxide
The properties of titanium dioxide are central to its versatility and effectiveness across various industries. Understanding these properties helps to uncover its unique role in applications ranging from pigments in paint to catalysts in environmental processes. Titanium dioxide’s characteristics significantly influence its applications and interactions with the environment. By examining its optical and catalytic properties, one can appreciate its standing in both industrial and emerging technology sectors.
Optical Properties
Optical properties of titanium dioxide include its ability to absorb and reflect light, which makes it critical for numerous applications.
Light Absorption
Light absorption in titanium dioxide is fascinating. The compound can absorb ultraviolet light, effectively acting as a barrier to harmful radiation. This is especially crucial in sunscreens, where protecting the skin from UV damage is a priority. The key characteristic here is the bandgap of titanium dioxide, which falls within the UV range. When titanium dioxide absorbs this light, it can excite electrons, contributing to various photochemical processes. This makes it a popular choice in UV-filter formulations.
Some might argue that the disadvantage of such high absorption is its limited effectiveness in the visible spectrum. However, this characteristic often enhances its utility in industrial applications where UV light exposure is more common.
Reflectivity
Reflectivity is another vital optical property of titanium dioxide. This compound exhibits exceptional reflectivity in the visible range, allowing it to scatter and reflect light effectively. This property is particularly beneficial in applications like paints and coatings, where brilliant white finishes are desired. Rutile form of titanium dioxide, in particular, provides superior reflectivity compared to other white pigments.
Yet, the unique feature of titanium dioxide's reflectivity is that it does not just bounce light; it can also enhance the brightness and opacity of coatings. One downside is that excessive reflectivity can lead to an overly bright appearance under certain lighting conditions, which may not be desirable in all contexts.
Catalytic Properties
Catalytic properties of titanium dioxide showcase its transformative potential, especially in environmental applications.
Photocatalysis
Photocatalysis is a standout feature of titanium dioxide, enabling it to facilitate chemical reactions under light exposure. This characteristic is pivotal in applications like air purification and wastewater treatment. The ability to harness light for driving reactions adds to its appeal as a sustainable solution. Essentially, titanium dioxide generates reactive species when exposed to UV light, breaking down pollutants into less harmful products.
The advantage here is not just limited to efficiency; it also promotes a greener approach to industrial processes. However, the dependency on UV light can be seen as a downside, especially in environments where UV exposure is limited, thereby reducing efficiency.
Environmental Applications
Environmental applications of titanium dioxide leverage its properties for pollution control and energy production. Its ability to decompose organic contaminants makes it an essential player in photocatalytic processes. This property serves as a double-edged sword; while it offers remarkable effectiveness in breaking down pollutants, it can also lead to the formation of by-products that need further assessment.
The key characteristic here lies in its potential to contribute to cleaner air and water. This quality underscores its significance not just in the industrial landscape but also in sustainability efforts. Understanding and managing the trade-offs involved in its use will be crucial as communities look towards greener solutions.
In summary, titanium dioxide’s properties shape its role in both traditional and innovative applications, making it an invaluable asset in addressing contemporary challenges.
Applications of Titanium Dioxide
Titanium dioxide plays a significant role across a plethora of industries, serving both functional and aesthetic purposes. Its versatility is one of the major facets that contributes to its demand, making it essential in several applications. From paints to cosmetics, titanium dioxide is valued for its unique properties. This section dives into two main areas of application: industrial uses and emerging technologies.
Industrial Uses
Pigments in Paints
When it comes to paints, titanium dioxide is the gold standard for pigmentation. The compound is prized for its superior opacity and brightness, which makes colors pop more than they would with other pigments. The reason for this is straight-forward; it scatters light effectively due to its high refractive index. This characteristic supports manufacturers in achieving a richer visual product.
The use of titanium dioxide in paints also leads to increased durability. Its resistance to discoloration under UV light means that exterior paints maintain their color longer, making it a cost-effective choice for homeowners and industrial applications alike.
However, it’s not all sunshine and rainbows—handling titanium dioxide vapor can pose health risks if inhaled in large amounts. Thus, manufacturers must follow stringent guidelines so that workers remain safe during production.
Cosmetic Formulations
In the world of cosmetics, titanium dioxide shines again. Its broad-spectrum UV protection makes it a popular ingredient in sunscreens and foundations. The presence of this compound not only protects skin from harmful radiation but also enhances the texture, lending products a smooth finish. Moreover, since it's non-toxic, it's a favorable option for formulations aimed at sensitive skin types.
Another feature worth mentioning is its ability to create a matte effect, which is especially appealing in makeup products. However, with popularity comes scrutiny; concerns about the long-term effects of nanoparticles in cosmetics have emerged. Some consumers are wary, calling for transparency in labeling and ingredient sourcing.
Emerging Technologies
Nanotechnology
The field of nanotechnology exploits the properties of materials at an astonishingly tiny scale. In the case of titanium dioxide, nanostructures have been shown to enhance its photocatalytic properties. What this means is that when exposed to UV light, nano-sized titanium dioxide can facilitate chemical reactions that break down organic pollutants. Therefore, its utility in environmental cleanup technologies cannot be overstated.
These nanostructures also exhibit other capabilities, such as improving the functionality of anti-fogging and self-cleaning surfaces. However, there is an ongoing debate about the environmental impact of nanomaterials, leading to calls for rigorous assessment protocols to ensure safety.
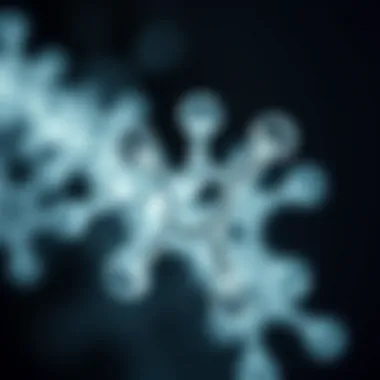
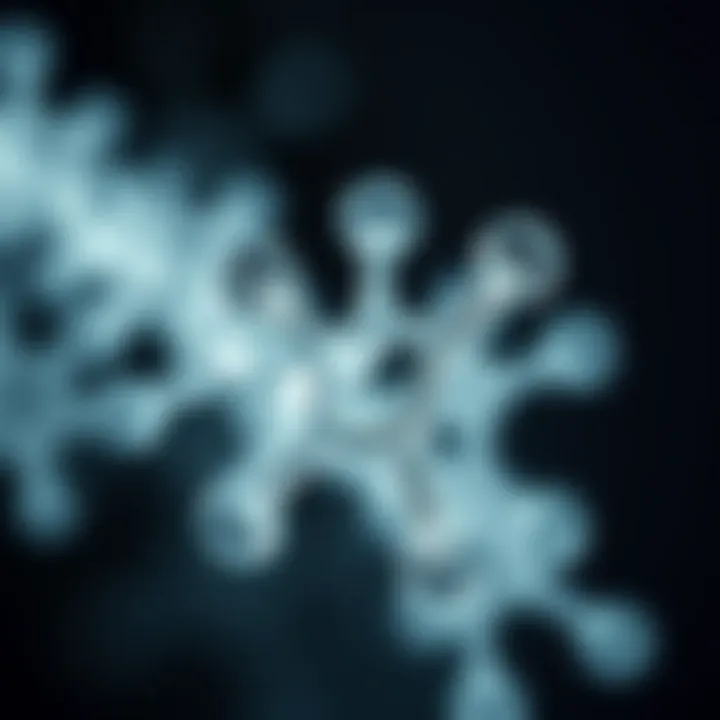
Solar Cells
Solar energy solutions are becoming increasingly mainstream, and titanium dioxide is making a mark in this arena. In the fabrication of dye-sensitized solar cells, it acts as a semiconductor. Its ability to absorb sunlight efficiently translates into higher energy conversion rates, which is pivotal for sustainable technology.
This application not only reduces dependency on fossil fuels but also paves the way for a more sustainable future. But, the initial costs of these solar cells can be relatively high, which poses a barrier for wider adoption. Investment and innovation in this area are necessary to drive down costs and improve performance.
Key takeaway: Titanium dioxide is not just a pigment; it is a cornerstone for current and future technologies that aim to innovate and protect our environment.
Environmental Impact
Understanding the environmental impact of titanium dioxide is essential in today's context, where industrial processes continuously face scrutiny over their ecological footprints. As titanium dioxide is widely used in various fields like pigments, cosmetics, and photocatalysis, it’s important to assess how its production and application influence both human health and natural ecosystems.
Toxicology and Safety
The toxicological profile of titanium dioxide is a focal point of research within environmental health fields. Various studies indicate that while titanium dioxide is generally considered safe for use in a range of products, concerns arise when nanoparticles of this compound are involved. Its ultrafine particles may cause adverse effects when inhaled or ingested, potentially leading to respiratory problems or cytotoxicity in cells.
- Studies show pulmonary issues after prolonged exposure in animal models, raising questions on workplace safety.
- Regulatory agencies such as the U.S. Environmental Protection Agency (EPA) have initiated assessments to determine safe exposure levels.
The safety of titanium dioxide in consumer products appears satisfactory when used as intended, yet the conversation surrounding its potential risks is ongoing. The recommended approach includes ensuring strict compliance with manufacturing guidelines and enhancing product transparency to equip consumers with essential knowledge to make safe choices.
Sustainability Considerations
Moving beyond immediate safety concerns, titanium dioxide poses multiple sustainability challenges, especially in its production processes. Traditional methods often involve mining practices that can lead to habitat destruction and biodiversity loss. Therefore, the need for sustainable extraction and production techniques becomes vital.
Some noteworthy aspects include:
- Alternative Synthesis: New avenues such as sol-gel methods and biomimetic approaches are emerging. They reduce environmental pressure by utilizing non-toxic precursors and energy-efficient processes.
- Waste Management: Implementing practices to manage waste produced during the synthesis of titanium dioxide, including recycling and proper disposal methods, is crucial for minimizing negative environmental effects.
- Eco-Friendly Applications: Leveraging titanium dioxide for sustainable technologies, such as in solar cells or photocatalytic coatings, boosts its potential as a green solution rather than merely an industrial compound.
In summary, while titanium dioxide holds significance in numerous applications, balancing its benefits against potential environmental hazards and promoting sustainability within its lifecycle has become a pressing necessity. The dual focus on human health and environmental integrity ultimately guides the future direction of this versatile compound's industry usage.
"Sustainable practices must not only protect immediate environments but also ensure the health of future generations, emphasizing the long-term consequences of today's choices."
Future Perspectives
The future perspectives regarding titanium dioxide (TiO₂) are not just important for academic discourse; they hold substantial implications for a variety of industries and environmental protocols. As we continue to harness titanium dioxide's properties, understanding its future role can lead to innovation in material science and sustainability efforts. What lies ahead could shift how we approach the synthesis and application of this compound, promoting new methodologies that are more efficient and eco-friendly.
Innovations in Synthesis
Recent years have seen a surge in innovative techniques designed to optimize the synthesis of titanium dioxide. Traditional methods, while effective, often consume considerable energy and can be detrimental to environmental health. Newer approaches tap into greener technologies, significantly reducing carbon footprints.
One promising development is the use of solvothermal synthesis, which enables precise control over particle size and morphology. By adjusting parameters like temperature and pressure, researchers can tailor TiO₂ for specific applications, be it in photocatalysis or solar energy conversion.
Another avenue is the exploration of biomimetic synthesis, which employs natural processes or materials to produce titanium dioxide. For instance, using plant extracts that contain titanium compounds could not only simplify the process but also yield nanoparticles with unique traits.
"The future of titanium dioxide synthesis lies in finding harmony between innovation and sustainability."
Incorporating machine learning and artificial intelligence into synthesis planning also stands out. These technologies can predict the best synthesis pathways, thus streamlining research efforts and decreasing trial and error phases. Essentially, these innovations promise to create titanium dioxide with enhanced properties while aligning with modern sustainability goals.
Advancements in Applications
As the technological landscape evolves, so do the potential applications for titanium dioxide. One of the most exciting prospects is its role in photovoltaics. Researchers are constantly looking to improve the efficiency of solar cells; integrating titanium dioxide could be instrumental given its favorable electronic properties.
Furthermore, the beauty industry is seeing transformations as well. New formulations in cosmetic products leverage TiO₂ for its effectiveness as a UV filter while maintaining a commitment to skin safety. Formulations that utilize nano-sized titanium dioxide particles can offer lighter textures and improved skin compatibility, providing consumers with higher-quality products.
In the realm of environmental science, titanium dioxide's photocatalytic properties could become paramount in addressing global challenges like pollution. Enhanced TiO₂ photocatalysts could facilitate the breakdown of harmful substances in water and air, paving the way for more efficient remediation techniques used across various environments.
As we embrace these advancements, we must also consider the balance between technological innovation and environmental stewardship. Groundbreaking applications will require a thorough understanding of the implications titanium dioxide might have on ecosystems and human health. Therefore, continuous research and public awareness will be crucial.
In summary, the future of titanium dioxide is bright. Innovations in its synthesis can lead to superior properties, while advancements in its applications promise to address pressing societal needs. The journey ahead encourages collaboration across disciplines, driving forward the stewardship of a compound that has already proven its worth in numerous applications.
Epilogue
In closing, the exploration of titanium dioxide's chemical structure is much more than a mere academic exercise; it is a vital foundation that informs its broad spectrum of applications and environmental interactions. Understanding the elements that make up this compound allows for deeper insight into why it's favored in industries ranging from automotive to pharmaceuticals.
Summary of Key Points
Titanium dioxide stands out due to its unique molecular arrangement and bonding characteristics. Here are some key takeaways from this discourse:
- Chemical Composition: Comprised mainly of titanium and oxygen, titanium dioxide can appear in various crystal forms, such as rutile and anatase, each exhibiting distinct properties.
- Synthesis Methods: The production processes, whether natural or synthetic, significantly influence the material’s properties and applications.
- Environmental Impact: Being aware of its toxicological aspect as well as sustainability considerations is crucial for responsible usage.
- Applications: Its roles span diverse fields including pigments in paints, applications in cosmetics, and even advancements in nanotechnology and solar cells.
The interplay of these factors reveals the complexities underlying titanium dioxide's roles in both industrial and environmental contexts.
Final Thoughts on Titanium Dioxide
To wrap it up, titanium dioxide deserves its reputation as one of the most multifaceted compounds utilized in modern society. Its chemical structure is not just a collection of atoms and bonds; rather, it's a testament to nature's intricacy and its potential for human innovation. By mastering its composition and properties, researchers and professionals can drive forward innovations, optimizing processes that hinge on this invaluable material.
"The simplest forms of matter, like titanium dioxide, can yield profound effects when properly understood and applied."
With ongoing research and development focused on enhancing its efficacy and minimizing environmental impact, the future landscape of titanium dioxide looks promising. It remains a point of interest not just for those in chemistry but for a wide array of fields looking to harness the benefits this compound offers.