Understanding Ultrasound Imaging Modalities
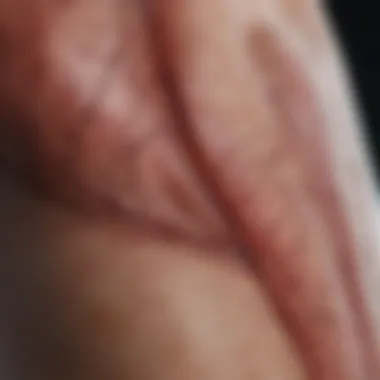
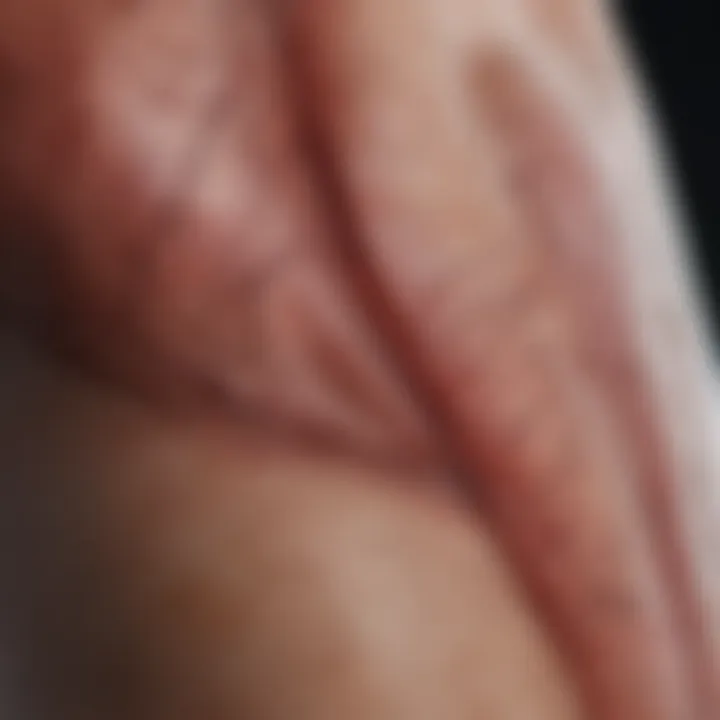
Intro
Ultrasound imaging has revolutionized the way medical professionals visualize the internal workings of the human body. This non-invasive technique leverages high-frequency sound waves to produce detailed images of organs, tissues, and even blood flow. As technology has advanced, various modes of ultrasound have emerged, each tailored to specific diagnostic needs.
In this article, we’ll sift through the intricacies of the primary ultrasound modalities: Doppler, B-mode, and A-mode. Each mode serves distinct purposes that are vital for accurate medical assessments. This guide will shed light on how these different modalities operate, their respective advantages and limitations, and their roles in modern diagnostics.
Overview of Research Topic
Brief Background and Context
Ultrasound technology traces back to the late 19th century, but it was only in the 1950s that it found a foothold in clinical applications. Initially used for fetal monitoring, it has since expanded into various fields, including cardiology, musculoskeletal imaging, and vascular studies. With ongoing developments, ultrasound is not merely confined to diagnostics; it’s also pivotal in therapeutic applications.
Importance in Current Scientific Landscape
- Enhancement of Diagnostic Accuracy: Advances in ultrasound imaging techniques have dramatically improved the detection rates of various conditions, including tumors and cardiac abnormalities.
- Real-Time Imaging Capabilities: Unlike some imaging modalities, ultrasound provides instantaneous feedback, which is crucial during procedures that require precision.
- Minimally Invasive: Ultrasound avoids the drawbacks often associated with radiation exposure found in X-rays and CT scans, making it the preferred choice for many patients.
In an era of personalized medicine, understanding the nuances of ultrasound imaging can provide professionals with the insights they need to make well-informed decisions. As we delve deeper, we'll explore the diverse modes of ultrasound, starting with B-mode, which serves as the foundation for most ultrasound imaging today.
Prelims to Ultrasound Technology
Ultrasound technology stands as a cornerstone in the field of medical imaging, offering invaluable insights into the human body without the need for invasive procedures. By harnessing the power of sound waves, ultrasound creates live images that provide critical information for diagnosis and treatment. This section lays the groundwork for understanding how ultrasound operates and underscores its significance across various medical disciplines.
One of the key benefits of ultrasound technology is its ability to produce real-time imagery. This feature is particularly advantageous in urgent care settings where immediate decision-making is essential. Unlike other imaging modalities such as MRI or CT scans, ultrasound is often more accessible and involves less expenditure. This makes it an appealing option not just for patient diagnosis but also for monitoring and guiding procedures.
In addition, ultrasound poses minimal risk to patients, as it uses non-ionizing radiation. This aspect makes it favorable for a variety of patient populations, including pregnant women and infants, who are especially susceptible to the harmful effects of radiation. Moreover, the portability of ultrasound machines allows their use in diverse environments, from large hospitals to remote clinics.
The fundamental principles governing ultrasound technology revolve around sound wave propagation and reflection. Grasping these principles is essential for appreciating how imaging modes function at a more granular level. The examination of ultrasound technology also involves a historical overview, revealing the innovative leaps that have transformed basic sonographic practices into the advanced imaging techniques we utilize today.
Understanding the various modes of ultrasound imaging can dramatically improve diagnostic accuracy and patient outcomes. So, let’s take a deeper dive into the historical context of ultrasound development and explore how far we've come in this dynamic field.
Historical Context of Ultrasound Development
The story of ultrasound traces back to the early 20th century when sonar technologies were initially developed for marine navigation. During the 1940s and 1950s, scientists began to explore the potential of using sound waves for medical imaging. It was a stroke of ingenuity that linked this technology, originally designed for ships, to healthcare.
In 1958, Dr. Karl Dussik became one of the first to apply ultrasound in medicine, using it to visualize brain tumors. Soon after, advancements in ultrasonic equipment and techniques proliferated, paving the way for the ultrasound machines we know today. The 1970s saw the introduction of real-time imaging capabilities, which was nothing short of a game-changer. During this era, Dr. Ian Donald led significant research that established obstetrical ultrasound as a standard practice, fundamentally changing how prenatal care is administered.
Through the decades, ultrasound technology has continued to evolve, shifting from simple two-dimensional imaging to sophisticated three-dimensional and four-dimensional modalities. This evolution has ceased to be just a technological leap; it has turned into a movement toward enhancing patient experience and diagnostic efficacy in healthcare.
Basic Principles of Ultrasound Waves
At the core of ultrasound technology are sound waves, specifically those that operate at frequencies greater than the upper audible limit of human hearing, typically above 20 kHz. When a transducer emits these sound waves, they travel through various tissues in the body. Different tissues, like muscle, fat, and fluid, impact the sound waves in distinct ways.
The essential principle behind ultrasound imaging is the reflection of these sound waves. Upon encountering a boundary between two types of tissue (like muscle and fat), part of the sound wave bounces back to the transducer. This phenomenon produces echoes, which are then converted into visual data that doctors can interpret. The intensity and timing of the returning echoes allow for the construction of detailed images across different modalities.
Key characteristics of ultrasound waves include:
- Frequency: Determines the resolution and penetration depth of the waves.
- Wavelength: Affects the detail of the image; shorter wavelengths lead to better detail.
- Reflection and Refraction: Critical for interpreting images, as different tissues will reflect sound waves differently.
In summary, the basic principles of ultrasound are not just scientific theories; they shape the functionalities and applications of ultrasound in clinical practice. Understanding these fundamentals prepares one to appreciate the nuances of each imaging mode that will be explored in subsequent sections.
Understanding Imaging Modes
Understanding imaging modes is crucial in the realm of ultrasound technology, as it lays the foundation for grasping how imaging techniques function and their implications in clinical practice. Each mode, despite serving a unique purpose, collectively contributes to a comprehensive diagnostic toolkit. For medical professionals, delving into these modes enhances interpretative skills and informs choices in clinical applications. Let’s break this down into its core components.
Definition and Importance of Imaging Modes
Imaging modes refer to the various techniques by which ultrasound equipment captures and presents data from within the human body. Imaging modes play a pivotal role in determining the clarity, accuracy, and utility of ultrasound diagnostics. They are not just a technical aspect; instead, they significantly influence the diagnostic quality and patient outcomes.
- B-mode imaging provides a two-dimensional grayscale image and is widely used because of its effectiveness in visualizing soft tissues.
- Doppler imaging offers insights into blood flow and movement, crucial for cardiovascular assessments.
- A-mode imaging, although less common today, is instrumental in specific applications such as measuring the distance to tissues.
The understanding of these modes helps practitioners recognize when to use each technique, ensuring that the right mode fits the clinical scenario. Hence, thorough knowledge of imaging modes is indispensable for professionals in fields such as radiology, obstetrics, and cardiology.
Overview of Common Ultrasound Modes
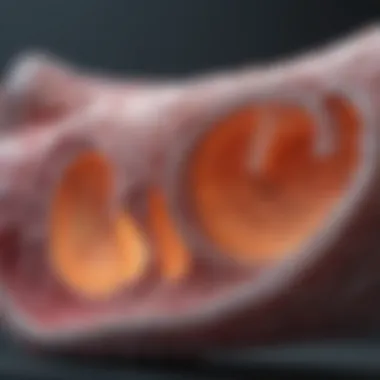
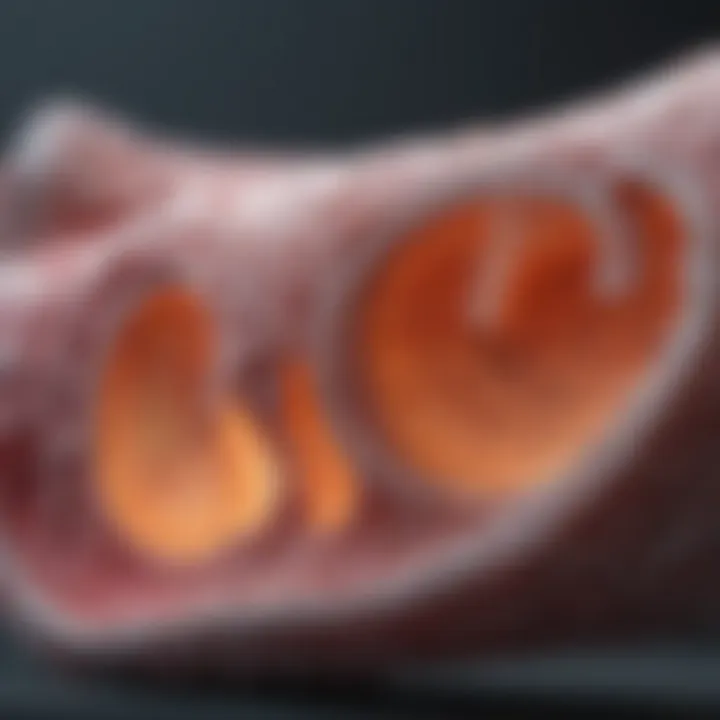
Ultrasound technology encompasses several imaging modes, each possessing distinct characteristics that tailor how images are produced and interpreted. Here’s a closer look at the primary modes used today:
- B-mode (Brightness mode): This mode captures a cross-sectional view of the body, displaying echoes from tissues as a grayscale image. It is essential in revealing structural anomalies.
- Doppler mode: Employs the Doppler effect to assess blood flow. This mode is divided into:
- A-mode (Amplitude mode): Primarily used for measuring distances rather than imaging. It displays a one-dimensional plot, commonly utilized in ophthalmology and assessing cardiac structures.
- 3D and 4D Ultrasound: These advanced modes allow for three-dimensional visualization and real-time imaging, enhancing the assessment of complex structures. They are particularly beneficial in obstetric imaging, offering parents a clearer picture of fetal development.
- Applications: Obstetrics, organ examinations, and soft tissue evaluation.
- Continuous Wave Doppler: Best for high-velocity blood flow measurements but does not provide depth information. Ideal for cardiac evaluations.
- Pulsed Wave Doppler: Allows for depth localization, making it effective for various vascular assessments.
Each imaging mode comes with its own set of advantages and considerations, shaping the pathway for what can be visualized and understood during an ultrasound examination.
Understanding these distinct modes enables practitioners to make informed decisions, ensuring optimal imaging and accurate interpretations—critical elements in delivering quality healthcare.
B-mode Ultrasound Imaging
B-mode ultrasound imaging plays a pivotal role in modern medical diagnostics. It is often the go-to mode for obtaining detailed cross-sectional images of the human body, providing a two-dimensional representation of a structure. This method relies on the reflection of ultrasound waves to generate images, illuminating details that are critical for accurate diagnosis. Given its wide array of applications, B-mode imaging serves as a backbone in various fields of medicine, from obstetrics to cardiology. The benefits of this modality are myriad, including the ability to visualize soft tissues and organs in real-time, making it invaluable for monitoring, diagnosis, and treatment planning.
Mechanism of B-mode Imaging
The mechanism behind B-mode imaging hinges on the transmission of ultrasound waves through various tissues. When the ultrasound probe emits these sound waves, they travel through the body and bounce back, or reflect, when encountering boundaries between different types of tissues. The reflections are captured by the same probe, which then processes these signals into images.
- The depth of the tissue is calculated based on the time it takes for the sound waves to return.
- Each reflection strength contributes to the pixel intensity, creating a grayscale image that depicts the anatomy being examined.
This technique allows for the visualization of structures in a range of densities, enabling healthcare providers to differentiate between normal and abnormal tissues effectively. For a clearer picture, clinicians often augment B-mode images with color Doppler techniques to assess blood flow, thereby enhancing diagnostic accuracy.
Applications in Clinical Settings
B-mode ultrasound is widely utilized across numerous clinical settings due to its versatility and real-time imaging capabilities. Some key applications include:
- Obstetrics and Gynecology: Here, it assists in monitoring fetal development, evaluating placental position, and diagnosing potential complications during pregnancy.
- Cardiology: In this field, B-mode imaging is essential for assessing heart structures and functions, helping identify issues such as congenital defects or valve dysfunction.
- Abdominal Imaging: Clinicians employ B-mode ultrasound to visualize organs like the liver, kidneys, and pancreas, allowing for the detection of lesions or abnormalities.
- Musculoskeletal: It’s also employed for examining musculoskeletal conditions, helping visualize muscles, ligaments, and joints to diagnose tears or inflammation.
These applications underline the adaptability and reliability of B-mode ultrasound, making it a crucial tool in diagnostic medicine.
Advancements and Innovations in B-mode
Recent advancements in B-mode ultrasound technology are revolutionizing its applications and effectiveness. Notable innovations include:
- Enhanced Image Quality: Developments in transducer technology have improved resolution and depth penetration, allowing for clearer and more detailed images.
- Portable Ultrasound Devices: The rise of pocket-sized ultrasound machines has made B-mode imaging more accessible, especially in remote or emergency settings.
- Artificial Intelligence Integration: AI algorithms are now being used to assist in interpreting ultrasound images, which could reduce human error and enhance diagnostic accuracy.
- Fusion Imaging: The combination of B-mode with other imaging modalities such as CT or MRI is gaining traction, enabling comprehensive assessments of complex conditions.
These advancements suggest a promising future for B-mode ultrasound, ensuring it remains at the forefront of diagnostic imaging.
Doppler Ultrasound Techniques
Doppler ultrasound techniques play a crucial role in the medical imaging landscape, particularly for assessing the movement of blood and other fluids within the body. Understanding these techniques allows practitioners to gather valuable insights into circulatory dynamics, which can be pivotal in diagnosing various conditions. Doppler ultrasound has shifted the paradigm of patient monitoring by offering a non-invasive means to evaluate hemodynamics, thus enhancing clinical decision-making. The incorporation of Doppler methods into routine practice has made a significant mark on both diagnostics and treatment.
Fundamentals of Doppler Effect
At its core, the Doppler Effect is a fascinating phenomenon that relates to changes in frequency or wavelength of waves in relation to an observer moving relative to the source of the waves. This principle is elegantly simple: when a sound source approaches, the waves are compressed, leading to a higher frequency; conversely, as the source moves away, the waves are stretched, resulting in a lower frequency. In the realm of ultrasound, this translates into the ability to measure the velocity of moving blood cells. The frequency shift can be calculated, showing how fast the blood flows in arteries or veins, which is invaluable in both diagnosing conditions and monitoring ongoing treatments.
Doppler Modes: Continuous Wave vs. Pulsed Wave
Doppler ultrasound is primarily divided into two modes: Continuous Wave (CW) and Pulsed Wave (PW). Each comes with distinct characteristics and applications:
- Continuous Wave (CW) Doppler: This mode offers the advantage of measuring high-velocity blood flow without aliasing, which can occur at high rates in pulsatile flow. Since it emits ultrasound continuously, it can detect blood flow velocities up to 3 m/s and beyond. However, it can be challenging to pinpoint the exact location of flow, which can be a limitation. This is often used in echocardiography and situations where speed is paramount.
- Pulsed Wave (PW) Doppler: In contrast, this mode emits ultrasound pulses intermittently, which allows for precise depth localization of the flow measurement. The major advantage here is the ability to assess flow at specific depths, useful for diagnosing conditions like stenosis. The PW Doppler is more versatile but limited by the aliasing phenomenon, especially at higher velocities.
Both modes find their place in clinical settings and are invaluable in assessing vascular health.
Clinical Applications and Benefits
Doppler ultrasound exhibits a wealth of clinical applications that bolster its relevance in everyday medical practice. Here are a few critical applications:
- Cardiology: Evaluating heart valve function and chamber pressures.
- Obstetrics: Monitoring fetal health by assessing blood flow in the umbilical artery.
- Vascular Health: Detecting abnormal blood flow patterns that might signal thrombosis or other vascular pathologies.
- Neurology: Assessing blood flow in the brain to identify risks of stroke.
The benefits of using Doppler techniques include:
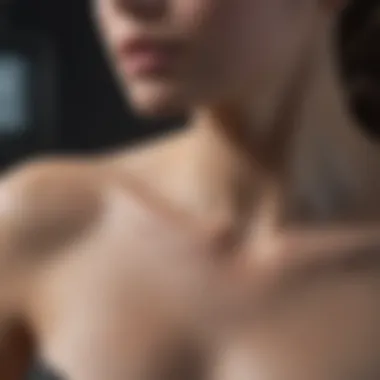
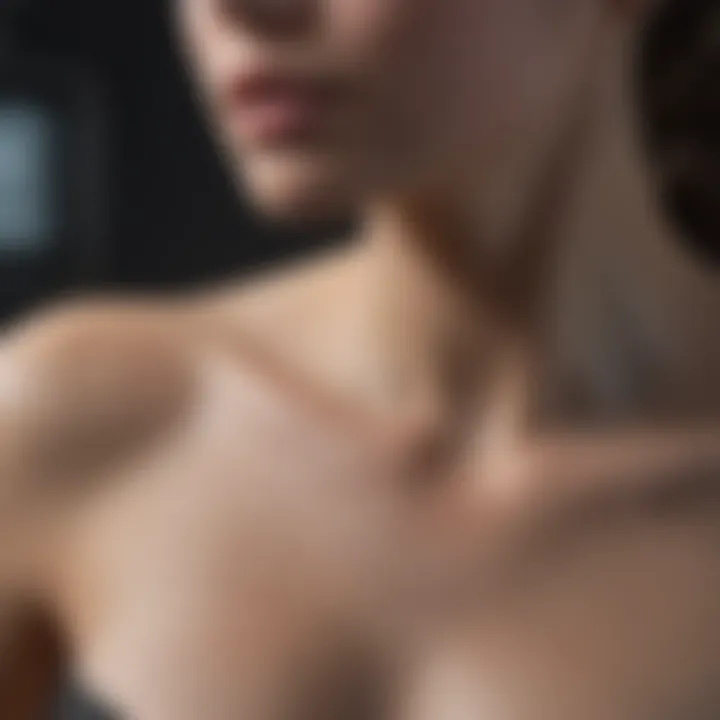
- Non-invasive Nature: Unlike some other imaging modalities, Doppler ultrasound does not require invasive procedures, thus minimizing risks to the patient.
- Real-time Imaging: Practitioners gain immediate insights into blood flow dynamics, enabling quicker decision-making in emergency situations.
- Comprehensive Assessment: With the ability to evaluate both speed and direction of flow, Doppler techniques provide a holistic picture of vascular health.
Doppler ultrasound is revolutionizing patient care by merging technology with methodologies that enhance diagnostics and patient monitoring.
In summary, Doppler ultrasound techniques serve as an indispensable tool in modern medicine, facilitating a deeper understanding of physiological and pathological processes. Their capacity to offer detailed analyses of blood flow—whether it's assessing cardiac function or ensuring fetal well-being—underscores their vital importance in clinical practice.
A-mode Ultrasound Applications
A-mode ultrasound, short for amplitude modulation, holds a unique position within the realm of ultrasound imaging. While it may not be as commonly employed in clinical settings compared to B-mode or Doppler techniques, its specific applications still render it invaluable in certain contexts. Understanding A-mode imaging is essential for grasping the foundational concepts behind various ultrasound modalities and recognizing the niche it occupies.
Preamble to A-mode Imaging
A-mode imaging presents data in a one-dimensional format where peaks in amplitude correspond to the interfaces of various tissues within the body. This mode provides a clear and direct presentation of depth information, making it straightforward for practitioners to analyze the distances between reflective boundaries.
In practice, the A-mode display features a horizontal axis indicating depth (or distance) and a vertical axis representing the amplitude of the returning echo. This way, medical professionals can gauge the structure's characteristics based on the heights of the peaks.
While A-mode imaging may seem simple, it fundamentally enables more complex imaging methods by serving as its building block. It’s essential for various areas, particularly in assessing body cavities or identifying tumors, thus reinforcing its importance in ultrasound imaging.
Comparative Analysis with Other Modes
When placing A-mode alongside B-mode and Doppler techniques, notable distinctions emerge.
- Complexity: Unlike B-mode, which offers two-dimensional imaging and color, A-mode doesn’t provide a visual representation of tissue structure; it communicates information through spikes that represent differences in echogenicity.
- Application Scope: While B-mode is more widely used for general imaging and Doppler for assessing blood flow, A-mode shines in specific niches like ophthalmology. Here, it assists in measuring the eye's length, crucial for cataract surgery and or intraocular lens placement.
- Speed and Efficiency: A-mode's simplicity allows for quicker assessments when compared to the detailed data required for B-mode and the calculations for Doppler techniques. As a result, it may be preferable in situations where rapid depth measurements are necessary.
This comparative analysis underscores A-mode's significant role in particular specialized fields rather than dismissing it as an inferior option compared to other imaging methods.
Current Uses in Medicine
A-mode ultrasound applications continue to find relevance in today’s medical practices, especially in situations requiring precision in depth measurement. Here are several notable uses:
- Ophthalmology: A-mode imaging provides essential measurements of axial length within the eye, guiding surgeries like cataract removal.
- Cardiology: A-mode can assist in measuring chamber dimensions and ventricular wall thickness, offering insights into patient heart health.
- Fetal Monitoring: In obstetrics, A-mode is sometimes used to determine fetal size and growth during pregnancy by analyzing the depth of echoes from the fetus.
"Despite its reduced prominence in everyday medical imaging, A-mode remains a crucial tool in specialized circumstances, showcasing its unique benefits and simplicity."
In summary, while A-mode ultrasound may not grab the spotlight often, its specialized applications highlight its importance within select medical fields. Understanding these applications not only enriches one’s knowledge of ultrasound technology but also underscores the integration of various imaging modalities in enhanced patient care.
The Role of 3D and 4D Ultrasound
The role of 3D and 4D ultrasound in modern medical imaging is one of considerable significance. These modalities extend the capabilities of traditional 2D imaging significantly, providing deeper insight into anatomical structures and functions. For instance, the ability to visualize a fetus in three dimensions not only enhances anatomical detail but also offers dynamic observation through 4D imaging, depicting movements in real time. This capacity has transformed prenatal care, allowing clinicians to evaluate fetal development and health more thoroughly than ever before.
Distinct Features of 3D and 4D Modes
3D ultrasound captures multiple 2D images from different angles, which are then reconstructed into a single three-dimensional image. This approach allows for detailed visualization of intricate structures that would often disappear in standard 2D views. One of the standout characteristics of 3D imaging is its ability to render surfaces and volumes, offering an almost lifelike representation of anatomical features.
Conversely, 4D ultrasound takes this a step further by adding time as the fourth dimension. With 4D, clinicians can observe dynamic processes — for example, a fetus moving, swallowing, or yawning. This adds an invaluable layer to assessment, especially in detecting potential anomalies. The difference often comes down to the depth of information available: while 3D provides a static snapshot, 4D allows for real-time observation.
"The leap from 2D to 3D and 4D ultrasound is akin to going from a flat, black-and-white photo to a vibrant, moving film; it profoundly alters our perspective on what we can see and understand about human biology."
Applications in Obstetrics and Gynecology
In obstetrics and gynecology, the applications of 3D and 4D ultrasound are manifold. One of the primary uses is in the assessment of fetal development during pregnancy. Healthcare professionals can monitor growth patterns, detect physical anomalies, and even evaluate fetal organs. This becomes especially important when parents are faced with higher risks of congenital conditions.
Additionally, in gynecology, these imaging modalities can assist in evaluating ovarian and uterine pathologies. For instance, 3D ultrasound is often employed to investigate uterine fibroids and polyp formations. These techniques provide more accurate measurements and spatial representations of such conditions, allowing for better planning of potential treatments or procedures.
Impact on Patient Experience
The impact on patient experience when using 3D and 4D ultrasound is undeniably positive. Many expectant parents express heightened engagement and emotional connection when they can see their unborn child’s features in such detail. This more interactive experience can alleviate anxiety surrounding pregnancy, particularly for those who may have concerns about fetal health.
Furthermore, the possibility of capturing 3D images to take home as keepsakes adds a unique sentimental value not present in traditional 2D imaging. In many clinics, parents can view the motions of their baby in real-time, further facilitating bonding.
In summary, as ultrasound technology continues to advance, the incorporation of 3D and 4D modes offers remarkable improvements in both diagnostic capabilities and patient experience, expanding the horizons of what medical imaging can achieve.
Challenges and Limitations of Ultrasound Modes
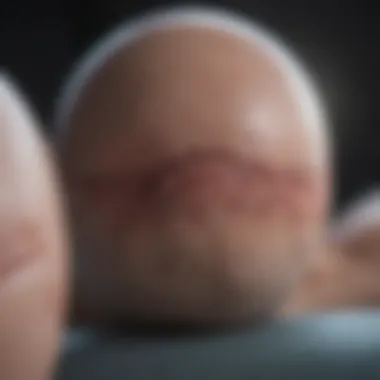
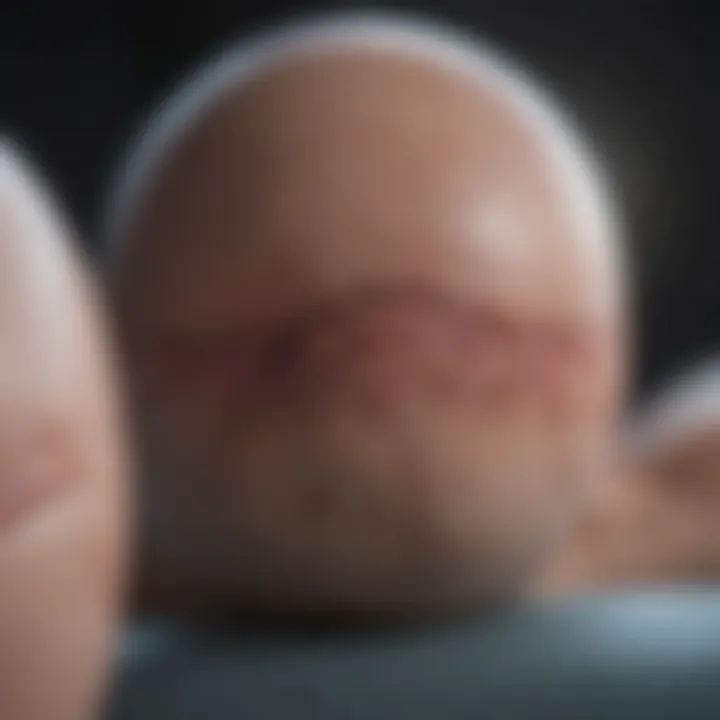
Ultrasound imaging, while a revolutionary tool in the medical field, is not without its hurdles. Understanding the difficulties posed by various ultrasound modes is crucial. Such knowledge aids in optimizing their application while acknowledging their limitations. This section delves into the technical constraints and interpretative challenges that practitioners might face when employing different ultrasound modalities. These insights are vital, ensuring that clinicians remain critical in assessing ultrasound images and choose the most suitable methods for accurate diagnostics.
Technical Limitations
Despite the remarkable advances in ultrasound technology, there exist inherent technical limitations.
- Resolution Constraints: One of the most significant issues is the resolution limits that can hinder the ability to visualize fine structures. For instance, while high-frequency ultrasound provides better resolution, its ability to penetrate tissues diminishes, making it a balancing act. When clinicians are pressed to see finer details, the choice of frequency is crucial.
- Depth Penetration: Navigating through various tissues can affect image quality. Deeper structures may be less clear due to attenuation of the ultrasound waves. Certain organs or masses may reside at a depth that challenges effective imaging. This situation may necessitate the use of specialized transducers or techniques.
- Artifact Complications: Artifacts are another area of concern that can muddle interpretation. They can arise from a myriad of sources, including motion from the patient or surrounding tissues. For instance, reverberation artifacts can lead to misinterpretation if not recognized and accounted for during analysis. This aspect adds an additional layer of complexity that practitioners need to consider.
To summarize, while ultrasound imaging plays a pivotal role in diagnostics, professionals must be aware of its technical limitations to mitigate issues that may arise during assessments or treatment decisions.
Interpretation Challenges
The interpretation of ultrasound images can be fraught with confusion and uncertainty, which may lead to suboptimal patient outcomes.
- Operator Dependency: One glaring challenge is that the interpretation of ultrasound images is often highly operator-dependent. This means that a skilled practitioner’s proficiency can greatly influence results. Lack of experience can lead to misdiagnosis or missed findings. This factor highlights the necessity for continuous education and skill refinement in the field.
- Varied Anatomy: Human anatomy can be quite variable, leading to different presentations of disease. What may appear as a benign finding in one patient could signify serious pathology in another due to anatomical differences. This variability necessitates a thorough understanding of normal anatomy and pathology, requiring a blend of knowledge and intuition on the part of healthcare providers.
- Limited Access to Comprehensive History: Unlike other imaging modalities, ultrasound may be used in acute settings where complete patient history is unavailable, complicating interpretation. Without full background info, a sonographer might struggle to distinguish between normal and abnormal findings, leading to potentially drastic consequences.
In closing, both technical limitations and interpretation challenges highlight the intricate dynamics involved in ultrasound imaging. Acknowledging these factors equips professionals with a framework to navigate complexities effectively, ultimately enhancing patient care.
Future Perspectives in Ultrasound Technology
The potential of ultrasound technology continues to blossom as we navigate the uncharted waters of medical innovation. The future of ultrasound imaging is not just about enhancements; it's about revolutionizing how we understand and approach medical diagnostics. The importance of this topic in our discussion of ultrasound modes is paramount. As this article has detailed the operational principles of various ultrasound modes, understanding future directions sets the stage for grasping their full potential in the medical field.
A few essential elements stand out when discussing future perspectives. Firstly, the integration of artificial intelligence (AI) into ultrasound technology is on the horizon. This entails advanced algorithms that can help in automating image interpretation. Imagine a future where sonographers can rely on AI to provide instant analysis while they focus more on patient care. Moreover, the potential for increased accuracy is tremendous. AI could reduce human error and provide standardized results, making diagnoses more reliable.
Another critical element is miniaturization. Portable ultrasound devices are already emerging, allowing for bedside diagnostics in hospitals or even remote areas. This shift emphasizes improved access to healthcare services, particularly in underprivileged regions. Modified ultrasound systems may be used in emergency settings or for routine check-ups, and this brings enhanced diagnostic capabilities right to the patient's door.
Future research is also anticipated to enhance image resolution and depth penetration through new ultrasonic frequencies and waveforms. With robustness in signal processing technology, we might observe greater clarity in real-time imaging.
"The advances of tomorrow in ultrasound technology hold the promise of transforming the clinical landscape, offering unparalleled benefits to patients and practitioners alike."
Finally, as the healthcare landscape continues evolving, coupling ultrasound with other technologies will enable comprehensive diagnostic solutions. Integrating ultrasound with genomic information or digital health records could lead to personalized care tailored to individual patient needs.
Emerging Technologies and Research
Emerging technologies hold the key to unveiling the next generation of ultrasound practices. In particular, three areas warrant significant attention, namely, contrast-enhanced ultrasound, high-intensity focused ultrasound (HIFU), and portable ultrasound devices. Contrast-enhanced ultrasound employs microbubble contrast agents to provide clearer and more detailed vascular imaging. This technique is gaining traction, especially in identifying hepatic lesions and assessing myocardial perfusion.
High-intensity focused ultrasound (HIFU) is another emerging field. HIFU uses concentrated ultrasound waves to heat and destroy tissue. Its applications in tumor ablation demonstrate promising results. As its use broadens, HIFU could reshape non-invasive surgical approaches, making procedures safer and more efficient.
Lastly, the advancement of portable ultrasound devices embodies the spirit of innovation—smaller, scans at the scene, not just in a hospital. Hospitals can now make the right decisions without delays, particularly in critical care settings. This is a game-changer as it enables timely interventions and saves lives.
Integration with Other Diagnostic Tools
The merging of ultrasound technology with other diagnostic tools heralds a new era in patient care. One significant aspect of this integration is its potential to enhance diagnostic accuracy. When coupled with magnetic resonance imaging (MRI), computed tomography (CT), or even positron emission tomography (PET), ultrasound can provide comprehensive insights into a patient's condition. This synergy allows for contrasting images and modalities that enhance diagnostic confidence.
Additionally, incorporating machine learning into radiological workflows signifies a notable shift. This technology could streamline processes, allowing radiologists to prioritize cases based on urgency. Automated systems could flag anomalies for further investigation, effectively harnessing the strengths of both ultrasound and other imaging modalities.
To put it simply, integrating ultrasound with other technologies isn't just about adding to the toolkit; it’s about constructing a multifaceted approach to patient health. The future may very well see a seamless convergence where a combination of modalities works together to deliver enhanced patient solutions, transforming the landscape of diagnostics.
Culminations
The exploration of ultrasound imaging modes culminates in a diverse understanding of their operation, application, and potential for future developments. As we look at the various techniques, it becomes clear that each mode serves specific purposes and has unique benefits that contribute to the overarching field of medical diagnostics. Understanding these conclusions is not just academic; it has real-world implications that can enhance patient care and influence clinical outcomes.
Summary of Key Findings
What stands out in our investigation is the fact that each mode in ultrasound imaging—B-mode, A-mode, and Doppler—offers advantages tailored to particular medical needs. For instance, B-mode is often the cornerstone for most diagnostic examinations due to its ability to produce detailed cross-sectional images of soft tissues. Doppler imaging, on the other hand, plays a pivotal role in assessing blood flow and cardiovascular conditions, allowing for critical evaluations of vascular health. Moreover, A-mode, while less common today, still finds its niche in specific applications, primarily focusing on measurement rather than detailed imaging.
A key takeaway is how technological advancements are continuously reshaping these modalities. From enhanced imaging software to improved transducer designs, the evolution of ultrasound technology is evident. This innovation not only boosts the precision of diagnoses but also facilitates earlier intervention in medical treatments, ultimately saving lives.
"Ultrasound technology, particularly its diverse modes, serves as a vital component in modern medicine, bridging diagnostics and patient care efficiently."
The Future of Ultrasound Applications
Looking ahead, the potential expansion of ultrasound applications is immense. Emerging technologies continue to redefine the boundaries of what is possible. For example, the integration of artificial intelligence in ultrasound systems is being explored, which could substantially improve the accuracy of interpretations and allow for predictive analytics in patient assessments.
In areas such as obstetrics, 3D and 4D ultrasound capabilities are gaining traction. This transformation enhances prenatal care by providing real-time insights into fetal development. Additionally, researchers are investigating portable ultrasound devices that could democratize access to this essential technology in remote areas or lower-resource settings.
As we consider these future applications, it's crucial to maintain a balance between innovation and training. Continued education for healthcare professionals is vital to ensure they can navigate new technologies effectively, which in turn can improve clinical practices.
In summary, the journey of exploring ultrasound modes reveals not just the current capabilities but also sets the stage for innovations that could profoundly impact medical diagnostics and patient care for years to come.