Understanding Space Charge Limited Current in Physics
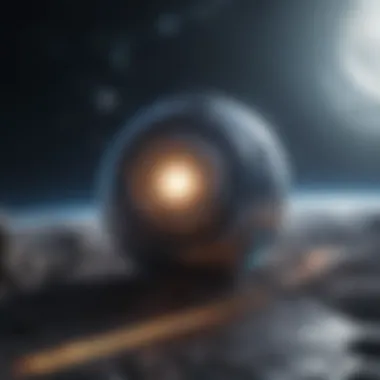
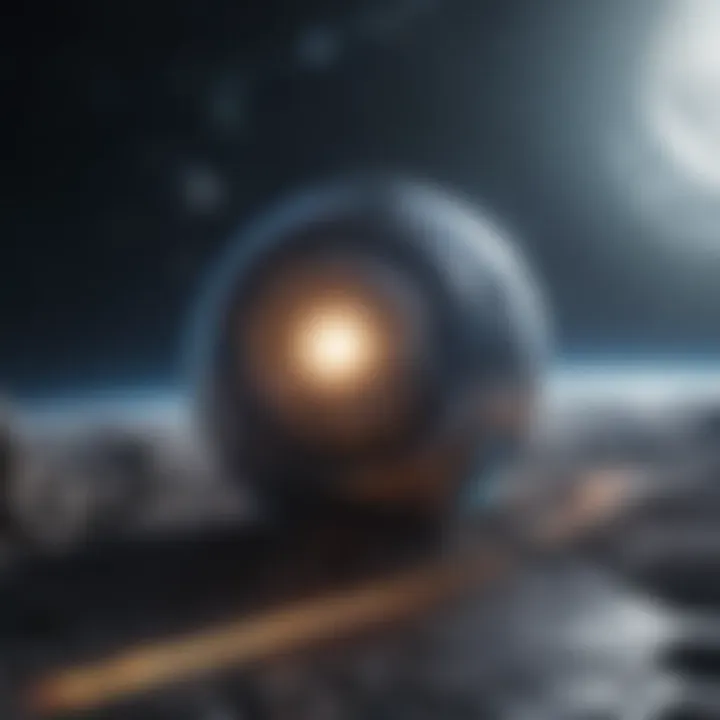
Overview of Research Topic
Brief Background and Context
Space charge limited current (SCLC) is a notion that arises from the behavior of charges in semiconductors and insulators. It refers to the current that flows through a material when the density of charge carriers, such as electrons or holes, becomes high due to an applied electric field. This scenario often occurs when there are insufficient free charge carriers to sustain the current at higher voltages. Historically, SCLC was emphasized during the mid-twentieth century with the advent of semiconductor physics. Researchers sought to understand how the current capacity of materials could be optimized for various applications, especially in electronic devices and optoelectronic components.
Importance in Current Scientific Landscape
Today, SCLC holds significant relevance in various advanced materials research. It plays a crucial role in the development of devices like organic light-emitting diodes (OLEDs), solar cells, and organic field-effect transistors (OFETs). Given the fast-growing field of renewable energy and flexible electronics, understanding SCLC is essential. Researchers are constantly looking for ways to improve efficiency and performance, and SCLC analysis offers valuable insights in optimizing potential materials.
Methodology
Research Design and Approach
The methodology used in examining SCLC often involves both theoretical and experimental approaches. Theoretical frameworks might include the Boltzmann transport equation or drift-diffusion models to derive predictions about current behavior under varying electric fields. Experimentally, techniques like current-voltage (I-V) measurements are conducted to enable direct observation and assessment of SCLC in materials. Researchers also apply analytical techniques to decipher the effects of material composition and geometry on current flow.
Data Collection Techniques
To effectively study SCLC, different data collection techniques are employed. Common practice includes:
- I-V Characterization: This technique gives insight into the electrical characteristics of materials spanning different voltage ranges.
- Charge Transport Studies: Researchers investigate the mobility and distribution of charge carriers under various conditions.
- Spectroscopic Methods: These methods help to understand material defects which play a vital role in the charge transport behavior.
Collectively, these methods enhance the understanding of SCLC and contribute to the optimization of materials in electronics and optoelectronics.
Intro to Space Charge Limited Current
The concept of space charge limited current (SCLC) is fundamental in understanding how charge carriers move through various materials. This phenomenon plays a crucial role in the performance and efficiency of electronic and optoelectronic devices. By examining SCLC, researchers and practitioners can gain insights into how materials behave under different conditions, which is particularly valuable in fields such as material science and condensed matter physics.
Definition of Space Charge Limited Current
Space charge limited current refers to the current that flows through a material under the influence of an electric field, limited by the space charge created by the accumulation of charge carriers. When the current density reaches a level where it is no longer linear with the applied voltage, space charge effects become significant. This results in a nonlinear increase in current, primarily due to the ability of charge carriers to recombine or become trapped in the material.
The mathematical expression for SCLC can often be represented using Shockley's equation, which describes the current density as a function of applied voltage in diodes and other semiconductor devices. This relationship is critical for designers and engineers to optimize the performance of various devices. The critical aspect of SCLC is its dependence on material properties and the geometry of the device, influencing how current is conducted and how efficiently devices operate.
Historical Development and Background
The understanding of space charge limited current has evolved over the last century. Early research on charge carrier dynamics in semiconductors laid the foundation for what would become a key area in electronic device physics. In the 1930s and 1940s, scientists like William Shockley began to formalize the concepts surrounding carrier movement and recombination within semiconductor materials.
As semiconductor technology advanced, so did the comprehension of SCLC. The invention of the transistor in 1947 marked a pivotal moment, allowing researchers to delve deeper into the behavior of charge carriers in real-world applications. Further empirical studies in the following decades highlighted the role of SCLC in influencing device performance, leading to significant advances in the design of diodes and transistors.
Today, the study of SCLC continues to be relevant. Innovations in materials science, such as organic semiconductors and nanomaterials, have expanded the landscape of SCLC research and applications. Understanding this phenomenon is essential for the development of more efficient electronic and optoelectronic components, making it a crucial area of study for scientists and engineers alike.
"Space charge limited current is a defining characteristic of electronic devices, influencing their efficiency and performance across various applications."
Fundamental Principles of SCLC
Understanding the fundamental principles of space charge limited current (SCLC) is crucial for comprehending its behavior and applications. These principles form the backbone of how electrical current behaves in different materials under the influence of electric fields, particularly when charge carriers are in excess.
Charge Carrier Dynamics
Charge carrier dynamics involves the movement of charge carriers, such as electrons and holes, within a material. These carriers play a pivotal role in the generation and conduction of current. In SCLC, the concentration of charge carriers influences current flow significantly.
Factors affecting carrier dynamics include:
- Carrier Mobility: The speed at which carriers move through the material. Higher mobility generally leads to higher current levels.
- Concentration Gradient: Changes in the density of charge carriers affect how they diffuse and drift. A steep gradient often results in a high current density.
- Recombination Processes: When carriers meet opposite charges, they can recombine. This process reduces the number of available carriers for conduction.
Accurate modeling of charge carrier dynamics is essential for predicting performance in various applications, from electronic devices to photovoltaic systems.
Drift and Diffusion Mechanisms
The drift and diffusion of charge carriers are key processes that dictate how SCLC manifests.
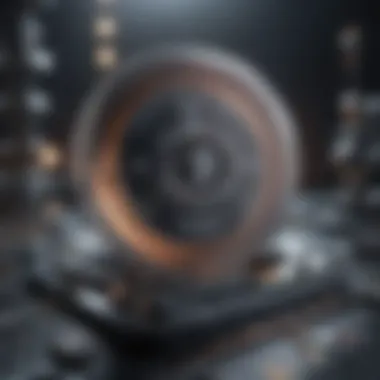
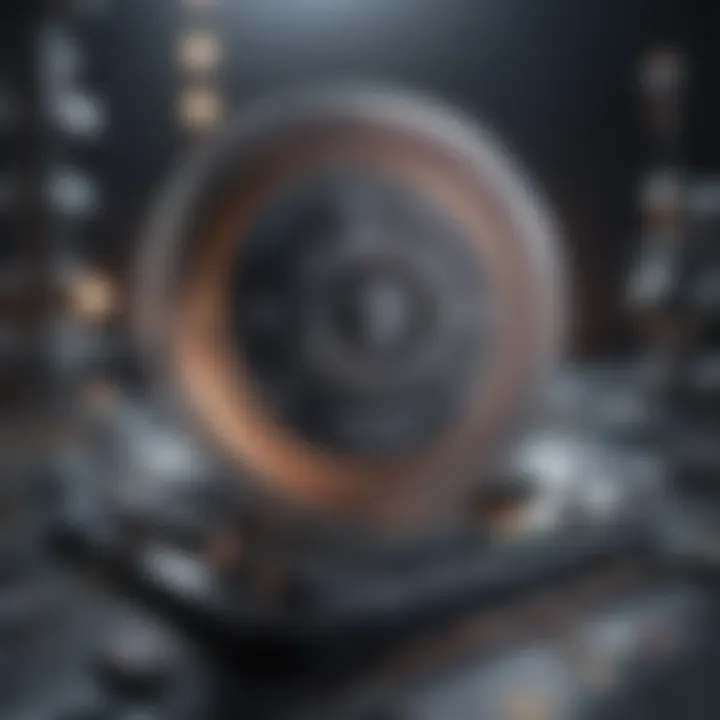
- Drift occurs when carriers move in response to an electric field. The direction and velocity depend on the strength of the field and the carrier mobility. This movement can be thought of as a direct response to external forces.
- Diffusion represents random movement driven by concentration gradients. Carriers tend to spread out from regions of high concentration to low concentration, influencing current flow in the absence of an electric field.
These mechanisms interact significantly. In regions where both drift and diffusion occur, understanding their balance is vital for accurately calculating current flow through materials.
Poisson's Equation and the Electric Field
Poisson's equation is a mathematical framework used to relate the electric potential in a material to its charge density. This equation becomes crucial when studying SCLC, as it helps to establish the electric field generated by space charges.
The electric field is a significant factor that influences how charge carriers move within the material. Changes in electrics fields can alter current flow, which makes understanding Poisson's equation essential for accurate predictions and modeling. Key points include:
- The electric field strength affects carrier drift, directly impacting the SCLC behavior.
- Accurate potential solutions from Poisson’s equation allow researchers to predict how materials will respond under various electric conditions.
"Understanding the underlying equations is essential to leverage the properties of materials effectively and optimize their use in technology."
Mathematical Modeling of SCLC
Mathematical modeling of space charge limited current (SCLC) is fundamental to fully grasp the behavior and characteristics of charge transport in various materials. These models offer insights into how charge carriers interact, move, and contribute to the overall current in a device. By understanding mathematical formulations, researchers can better predict performance under different conditions, enhancing the design and optimization of electronic and optoelectronic devices. Furthermore, accurate models can inform experimental setups and drive innovation in material science.
Basic Equations Governing SCLC
The foundation of SCLC theory lies in several key equations that describe the current density in terms of electric field and charge carrier mobility. The most notable among these is the Child–Langmuir Law, expressed as:
Here, ( J ) is the current density, ( \epsilon_0 ) is the permittivity of free space, ( \epsilon_r ) is the relative permittivity of the material, ( V ) represents the applied voltage, and ( d ) denotes the gap distance. This equation highlights the nonlinear relationship between current density and applied voltage, which is a hallmark of SCLC behavior.
In many cases, including higher charge injection scenarios, it's essential to consider
This parameterization captures how current density increases sharply as voltage rises, reflecting the limitations presented by space charges.
Approximation Techniques in Modeling
Creating mathematical models for SCLC often entails complex equations that may not always yield straightforward solutions. Hence, approximation techniques become invaluable. One common approach is the use of series expansions to linearize certain aspects of the equations. For example, linear approximations enable easier manipulation of the differential equations governing charge transport.
Another approach is the quasi-static approximation, allowing for the assumption that the electric field remains static while charges migrate. This greatly simplifies the calculations and provides a useful benchmark for analyzing experimental data.
Numerical Simulations and Computational Approaches
Given the intricate nature of SCLC, numerical simulations serve as powerful tools in studying this phenomenon. Techniques such as finite element analysis and Monte Carlo simulations are often deployed to numerically solve the governing equations. These methods facilitate a deeper exploration of SCLC behavior under various scenarios and help validate theoretical predictions.
Moreover, employing software such as COMSOL Multiphysics or MATLAB can streamline the process of generating and testing various models. Computational approaches enable researchers to visualize the impact of different material properties, electric fields, and boundary conditions on the SCLC characteristics.
The integration of complex mathematical modeling with experimental research paves the way for the advancement of SCLC applications in modern electronics.
In summary, mathematical modeling is essential when investigating SCLC. It provides a framework for understanding the interactions of charge carriers, simplifies complex phenomena through approximations, and combines with computational techniques to enhance predictive capabilities.
SCLC in Different Materials
The exploration of Space Charge Limited Current (SCLC) in different materials is crucial for understanding its impact on device performance and applications. This phenomenon is significant in semiconductors, organic materials, and dielectric substances. Each of these material classes presents unique considerations and benefits when analyzed through the lens of SCLC, ultimately influencing various technologies in electronics and optoelectronics.
SCLC in Semiconductors
In semiconductors, SCLC plays a vital role in determining the efficiency and performance of devices such as diodes and transistors. The special characteristic of semiconductors is their ability to conduct electricity under certain conditions. As charge carriers are injected into the semiconductor, the current can become limited by the space charge. This often happens when a high density of charge carriers is present. In this case, the current density becomes proportional to the square of the electric field, highlighting the need for adequate electric field management to optimize current flow.
Key factors affecting SCLC in semiconductors include:
- Material properties such as mobility and trap states.
- Temperature dependencies that can influence carrier dynamics.
- The geometry and layer thickness of the semiconductor.
Understanding these factors can help in designing better semiconductor devices.
SCLC in Organic Electronics
Organic electronics have emerged as a significant field, drawing attention for their flexible applications in displays and solar cells. The significance of SCLC in organic materials is paramount. These materials typically have lower carrier mobilities compared to traditional semiconductors. As a result, SCLC can dominate at higher voltages. The mobility of charges in organic films impacts how well devices can operate under varying conditions.
Benefits of SCLC in organic electronics include:
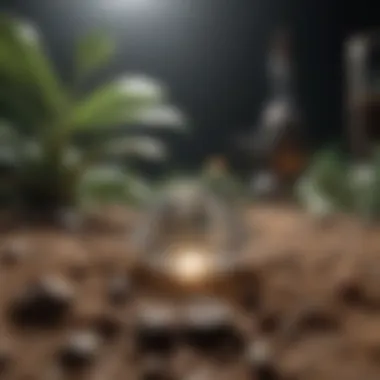
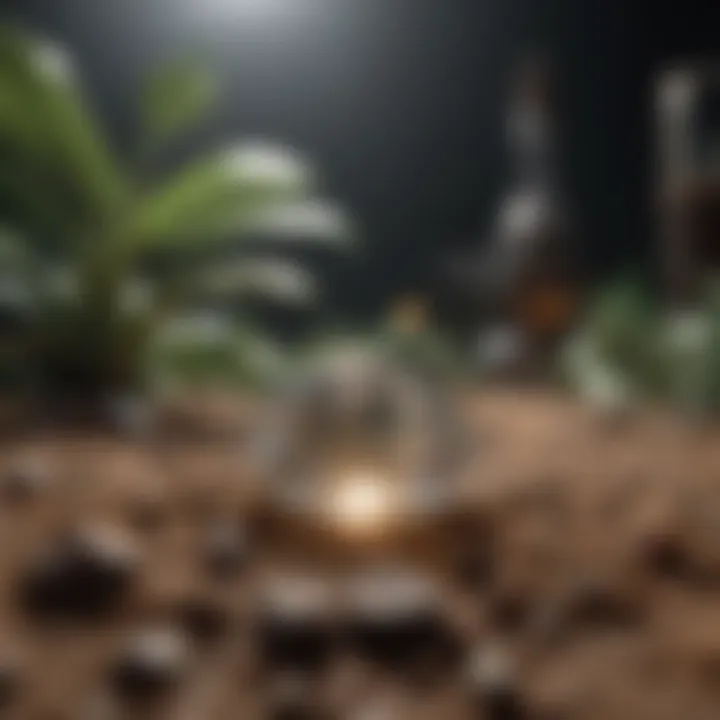
- Improved charge transport in devices designed for organic photovoltaics.
- Enhanced performance in organic light-emitting diodes (OLEDs).
- The ability to explore new materials that facilitate better charge injection and transport.
The integration of SCLC considerations into the design of organic devices is essential for optimizing their performance.
SCLC in Dielectric Materials
Dielectric materials, known for their insulation properties, also exhibit SCLC behavior under certain conditions. When an electric field is applied, some dielectrics can allow a limited degree of charge transport through space charge accumulation. This behavior is particularly important in capacitors and insulators in electronic devices.
Important aspects of SCLC in dielectric materials are:
- Breakdown voltage, which determines the material's limit before it becomes conductive.
- The dielectric constant, affecting overall current flow.
- The role of defects and impurities that can impact charge distribution.
In summary, the study of SCLC across different materials highlights its multifaceted impact on technology. The understanding of this phenomenon is essential for improving existing devices and innovating new applications in the fields of electronics and optoelectronics.
"SCLC is not just a concept; it is a fundamental guideline in material science for optimizing device performance."
By addressing these factors, researchers can refine their approaches and enhance the efficacy of various material applications.
Applications of SCLC
The concept of space charge limited current (SCLC) finds significant applications across various fields of electronics and material science. Its relevance stems from the ability to improve the efficiency and performance of numerous devices. The understanding of SCLC leads not only to enhanced device design but also to optimization in material usage. Exploring its applications reveals valuable insights into how this phenomenon plays a critical role in advancing technology.
Role in Photovoltaic Devices
The photovoltaic cell, a cornerstone in the development of renewable energy sources, heavily relies on SCLC principles. In these devices, the charge carriers must navigate through various materials to reach the electrodes. SCLC helps determine how well these charges can move. When the intensity of light increases, the generation of electron-hole pairs enhances, but SCLC outlines the limits at which these carriers can flow.
Some critical benefits include:
- Increased Efficiency: Proper understanding of SCLC can lead to designs that minimize losses, thereby boosting energy conversion efficiency.
- Material Selection: Understanding how different materials behave under SCLC conditions can help engineers choose the optimal components for photovoltaic cells.
- Improved Lifetime: By addressing issues related to charge accumulation, manufacturers can extend the lifespan of solar devices.
"SCLC not only impacts performance but also influences the long-term reliability of photovoltaic systems."
Influence on OLED Performance
Organic light-emitting diodes (OLEDs) are another area where SCLC plays a vital role. In OLEDs, the injection and transport of charges directly affect how well the device operates. The SCLC phenomenon helps to understand charge dynamics, which are crucial for light emission.
Key considerations include:
- Maximizing Light Output: SCLC can be optimized to achieve uniform charge distribution, which results in better light emission.
- Design Strategies: Understanding SCLC enables engineers to innovate in layer structure and material choice, enhancing overall device efficiency.
- Reducing Energy Costs: Improved charge injection means less energy wasted, making OLEDs more sustainable.
Impact on Transistor Technology
In the context of transistors, especially organic field-effect transistors (OFETs), SCLC is essential for determining operation characteristics. The flow of current through the semiconductor material is severely influenced by SCLC behavior, thus altering its performance metrics.
Important impacts to note:
- Performance Stability: The ability to model SCLC allows for more reliable operation in varying conditions, which is crucial for commercial applications.
- Scaling Dimensions: As transistors continue to shrink, SCLC insights provide guidance on how to maintain effective charge transport at smaller scales.
- Material Innovations: Knowledge derived from SCLC studies encourages researchers to explore new materials that may perform better than traditional options.
Recent Advances in SCLC Research
Recent advances in space charge limited current (SCLC) research play a pivotal role in enhancing our understanding of charge transport in various materials. These developments not only expand the theoretical framework governing SCLC but also provide practical applications in modern electronics and optoelectronics. As the field evolves, significant emphasis has been placed on exploring novel materials and innovative methods that could revolutionize device performance.
Nanostructured Materials and SCLC
Nanostructured materials have emerged as a significant focus in SCLC research due to their unique electronic properties. The reduction in dimensionality alters charge carrier dynamics, leading to an increased efficiency in devices. For instance, materials like graphene and carbon nanotubes demonstrate remarkable conductive properties, making them suitable candidates for SCLC applications.
Research indicates that these nanostructures facilitate both enhanced charge mobility and reduced recombination rates. Consequently, integrating nanostructured materials into devices can significantly boost performance and efficiency. Notably, the study of nanostructures also aids in understanding fundamental processes in charge transport, which has implications in semiconductor physics and material science.
Integration of SCLC in Flexible Electronics
Flexible electronics represent a burgeoning area where SCLC plays a crucial role. The demand for lightweight, flexible, and efficient devices has driven the integration of SCLC principles into this domain. Flexible substrates, such as polymers, allow for the creation of bendable and lightweight components, essential for modern applications like wearables and portable electronics.
Incorporating SCLC in these flexible devices helps optimize their performance under various mechanical strains. Researchers are focusing on enhancing charge transport in flexible photovoltaic cells and organic light-emitting diodes (OLEDs) by applying SCLC principles. This approach increases stability and prolongs the operational lifespan of such devices.
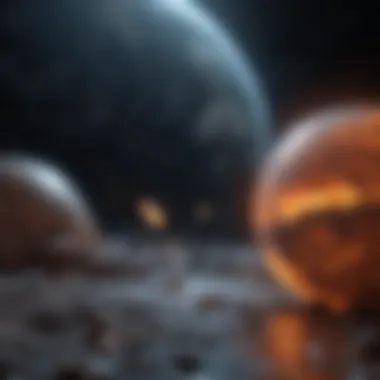
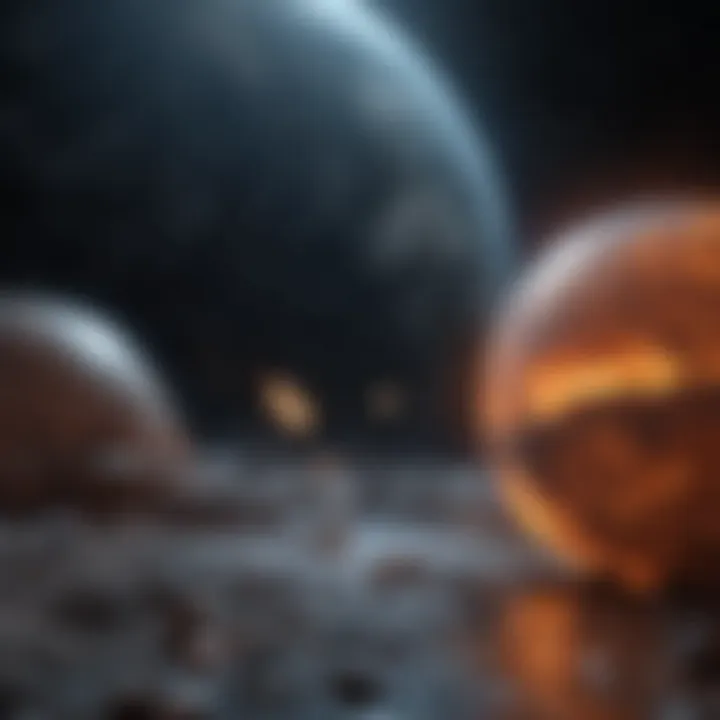
Innovations in Measurement Techniques
Advancements in measurement techniques are crucial for the accurate investigation of SCLC phenomena. Traditional measurement methods often face limitations due to environmental factors or material properties. Innovative techniques such as time-of-flight measurements and electrochemical impedance spectroscopy have gained popularity.
These methods enable researchers to gather more precise data on charge carrier dynamics and to investigate material responses under different conditions. The emphasis on reproducibility and reliability in measurements ensures that empirical data can be effectively compared with theoretical models. By improving measurement accuracy, scientists can deepen their understanding of SCLC behaviors and drive further innovations in material and device design.
"Understanding the principles of SCLC in nanostructured materials and flexible devices is essential for future technological advancements."
Theoretical and Experimental Challenges
Limitations of Current Models
Theoretical models provide a fundamental basis for predicting SCLC behavior, but several inherent limitations can undermine their effectiveness. One significant limitation is the simplification often applied in model assumptions. Many models assume uniform material properties, neglecting the complexities introduced by variations in material composition or structure.
Moreover, real-world conditions, such as temperature fluctuations or defects within the material, can considerably influence charge transport. Most existing models may fail to accurately capture these effects, leading to discrepancies between predicted and observed behaviors.
The validity of models is also challenged by their reliance on idealized parameters. For instance, models may operate under the assumption of constant electric fields or homogenous charge carrier distributions. In reality, these conditions are rarely met in practical scenarios, which can result in significant deviations from expected current levels under actual operating conditions.
"Models serve as a starting point, but the reality of material behavior seldom matches theoretical predictions precisely."
Experimental Difficulties in Measurement
On the practical side, measuring SCLC presents unique challenges. One of the primary difficulties is the setup required for accurate measurements. Precision instrumentation is often necessary to apply and control external electric fields while monitoring the resulting current. This process can be hindered by noise, thermal fluctuations, and other environmental factors that can distort readings.
Additionally, samples used for SCLC testing must be meticulously prepared. The presence of impurities or defects can alter the carrier dynamics significantly. A clean sample is essential for accurate results, yet achieving such purity in real-world conditions can be exceedingly complex.
Another noteworthy challenge is the extraction of meaningful data from experiments. The data obtained must be analyzed properly to draw reliable conclusions. This involves sophisticated signal processing techniques that can be resource-intensive and require expertise in data interpretation.
Future Directions in SCLC Studies
The field of space charge limited current (SCLC) research is continuously evolving, reflecting the advances in materials science and electronics. Understanding future directions in SCLC studies is vital for both theoretical developments and practical applications. This exploration goes beyond established models, pointing towards innovative materials and technologies that can shape electronic devices. Researchers are keenly interested in several emerging trends that promise to enhance device efficiency and performance.
Emerging Materials and Technologies
The innovation in materials for SCLC applications is substantial. Emerging materials, such as perovskite semiconductors, are becoming crucial as they offer an excellent balance between efficiency and flexibility. These materials are capable of achieving high charge carrier mobility while maintaining a low energy barrier. Additionally, 2D materials like graphene and transition metal dichalcogenides are also under examination. Their unique properties could open new pathways for SCLC efficiency.
Some important considerations in the selection of these materials include:
- Charge Carrier Mobility: High mobility can improve current flow and device response.
- Stability: Long-lasting materials are essential for practical electronics.
- Manufacturing Cost: Economical production methods will promote widespread application.
Exploring organic-inorganic hybrid materials could also yield significant advancements. Combining organic components with inorganic counterparts can produce materials with desirable electronic properties and structural flexibility. This integration is promising for future applications, particularly in flexible electronics.
Role of SCLC in Quantum Computing
As quantum computing technology develops, SCLC's role is becoming more prominent. The behavior of charge carriers in quantum devices can significantly affect coherence times and overall performance. SCLC mechanisms can provide insight into how these carriers interact under various conditions typical for quantum systems.
- Quantum Dots: The integration of SCLC concepts can improve the efficiency of quantum dot-based systems by optimizing charge carrier transport.
- Superconducting Qubits: Understanding space charge interactions can help enhance qubit performance and coherence, which is critical for reliable quantum computers.
The relationship between SCLC and quantum computing is still unfolding. Ongoing research may reveal novel insights into how space charge effects impact quantum devices and their scalability. Researchers must remain attuned to the implications of these findings as they could lead to breakthroughs in computing technologies.
In summary, exploring these future directions will not only enrich theoretical understanding but also pave the way for revolutionary applications in electronics and computing. Continued investigation of emerging materials and their role in quantum systems remains pivotal in the journey forward.
End
The conclusion section plays a vital role in encapsulating the crucial elements discussed throughout this article. It serves as a comprehensive summary, allowing readers to consolidate their understanding of space charge limited current (SCLC) and its far-reaching implications in various fields. In essence, this section not only revisits significant points but also underscores the relevance of SCLC in material science and device performance.
Summary of Key Insights
In summary, the exploration of SCLC reveals several key insights:
- Definition and Context: Space charge limited current is defined by the dynamics of charge carriers, where the current is constrained by the presence of charge in a material medium. It manifests in diverse materials like semiconductors and organic electronics.
- Underlying Principles: Fundamental principles such as the interplay of drift and diffusion mechanisms, governed by Poisson's Equation, elucidate the factors impacting current flow in these systems.
- Materials: The article highlights the behavior of SCLC across different types of materials, stressing its importance in contemporary applications, especially in devices like photovoltaics and organic light-emitting diodes.
- Challenges and Future Directions: The limitations of current models and the complexities of experimental measurements present ongoing challenges. Addressing these will pave the way for future research, facilitating advancements in technologies, particularly in quantum computing.
Final Thoughts on SCLC's Impact
SCLC's importance cannot be overstated. As material science progresses, understanding the nuances of space charge effects becomes crucial for optimizing electronic devices. With ongoing research into innovative materials and measurement strategies, SCLC continues to impact fields such as optoelectronics and flexible electronics. The knowledge gained from SCLC studies not only advances academic inquiry but also enhances practical applications in technology.
"The understanding of SCLC is indispensable for harnessing the full potential of emerging electronic and optoelectronic devices."
Recognizing these elements helps students, researchers, and professionals appreciate the complexities and capabilities associated with space charge limited current. The future directions highlighted pave the way for exciting developments that might redefine how we approach device engineering in the years to come.