Exploring Reverse PCR Testing: Techniques and Uses
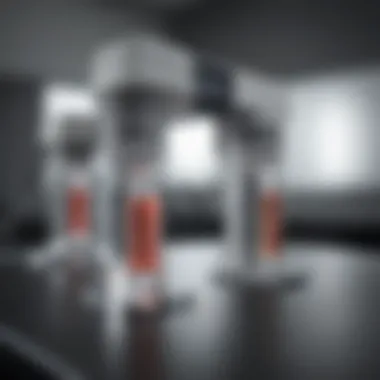
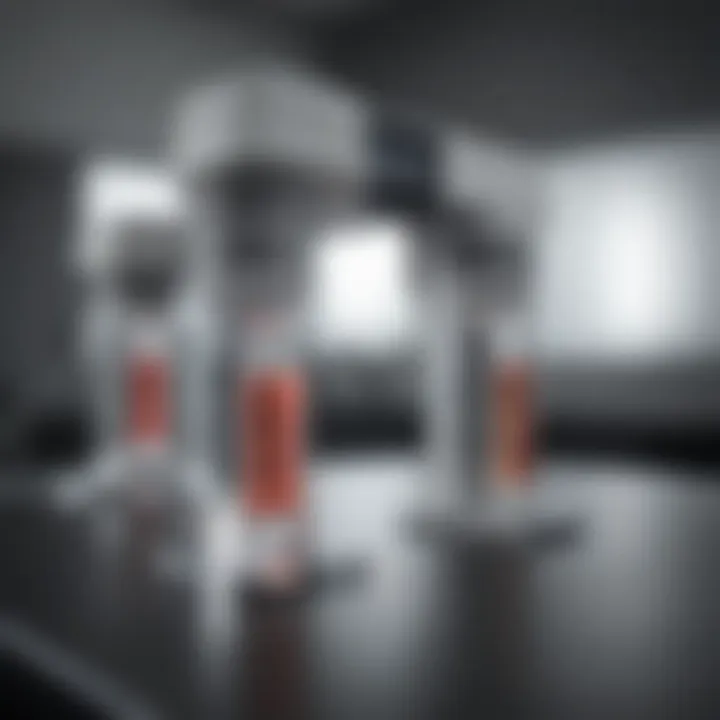
Overview of Research Topic
Reverse PCR testing serves as a pivotal tool in molecular biology, enabling researchers to obtain specific DNA sequences from complex mixtures. This method diverges from traditional PCR methods by focusing on amplifying sequences from cDNA. Understanding the intricacies of reverse PCR is crucial, given its applications in various biological and clinical settings.
Brief Background and Context
PCR, or Polymerase Chain Reaction, emerged in the 1980s, revolutionizing the field of genetic analysis. Traditional PCR techniques typically target DNA templates directly. However, reverse PCR introduces the unique concept of starting with RNA templates, which are first converted into cDNA. This initial step allows for the amplification of specific RNA sequences, thus offering a broader scope in studying gene expression and regulation.
Importance in Current Scientific Landscape
In today's rapidly evolving scientific environment, the significance of reverse PCR cannot be understated. With growing interest in genetic research, personalized medicine, and diagnostics, reverse PCR provides a means for scientists to analyze gene expression levels in various conditions. This method is not only valuable in academic research but also holds potential in clinical diagnostics, pathogen identification, and even therapeutic monitoring.
Methodology
The methodology of reverse PCR bears distinct features that differentiate it from traditional approaches. A well-structured design is essential for successful outcomes.
Research Design and Approach
In conducting reverse PCR, a systematic approach is required. Here are key aspects of the research design:
- Selection of RNA Samples: Careful consideration must be given to the RNA source, as the quality and integrity directly affect the results.
- cDNA Synthesis: The choice of reverse transcriptase enzyme plays a crucial role in the efficiency of cDNA synthesis. Typically, enzymes like M-MuLV or AMV are employed.
- Amplification Process: Following cDNA synthesis, specific primers are used in the amplification stage. Primer design is critical; mismatches can lead to non-specific amplification.
Data Collection Techniques
Data collection in reverse PCR involves several steps:
- Time and Temperature Testing: It is essential to optimize the temperature and cycle times for the specific reaction. Too high temperatures can lead to enzyme denaturation, while too low can yield incomplete reactions.
- Quantitative Analysis: Techniques such as real-time PCR can be integrated to quantify gene expression levels effectively.
- Validation Processes: Including standard curves and control samples during analysis helps to ensure the reliability and reproducibility of results.
"Every detail in protocol design matters. Small variations can significantly impact the amplification results."
Prelims to Reverse PCR
Reverse PCR represents a significant advancement in molecular biology, particularly for those engaged in genetic research and diagnostics. Understanding reverse PCR involves grasping its distinct mechanisms and applications that set it apart from traditional PCR methods. The unique capability of this technique to amplify DNA from reversed templates makes it a critical tool in various biological investigations.
Definition and Overview
Reverse PCR, or Reverse Polymerase Chain Reaction, is a modification of the standard polymerase chain reaction (PCR). This technique is primarily used to amplify specific DNA fragments that reverse transcribe nucleic acids, particularly in the context of detecting particular sequences in mixed populations or low-abundance samples. For researchers and scientists, the definition is straightforward: it is a powerful method that allows for the efficient extraction of genetic material from complex sources, thus enabling detailed genetic analysis. The technology capitalizes on the enzyme reverse transcriptase, which synthesizes complementary DNA (cDNA) from RNA, making it indispensable in gene expression studies.
Historical Context
The inception of reverse PCR can be traced back to the broader development of PCR technologies in the 1980s. While the standard PCR, developed by Kary Mullis, captured significant attention due to its efficiency in amplifying DNA, the challenge of working with RNA remained. Researchers needed innovative approaches to study gene expression and genetic mutations, especially in cellular environments rich in RNA. This demand led to the evolution of reverse transcription methodologies.
In the years following its introduction, reverse PCR witnessed advancements that improved its sensitivity and applicability across various fields, such as genetics and virology. The combination of reverse transcription and PCR opened new avenues for not just detecting but also quantifying genetic material, subsequently influencing how researchers approached complex biological questions.
Principles of Reverse PCR
Understanding the principles of reverse PCR is essential for comprehending how this technique plays a pivotal role in genetic analysis. Reverse PCR is designed to amplify specific DNA sequences, allowing for detailed examination of genetic material. This method provides unique advantages in various research applications, including genotyping, disease diagnosis, and expression studies.
Basic Mechanism
The basic mechanism of reverse PCR involves several critical steps that allow it to function effectively. First, reverse transcription is performed to convert RNA into complementary DNA (cDNA). This step is crucial because it forms the basis for the subsequent amplification process. After cDNA synthesis, the amplification phase begins. Using specific primers, the cDNA is then amplified through standard PCR thermocycling steps: denaturation, annealing, and extension.
The enzymes usually employed in this process are reverse transcriptase and DNA polymerase. Reverse transcriptase facilitates the conversion of RNA into cDNA, while DNA polymerase amplifies the cDNA produced. The specificity of primers ensures that only the desired sequence is amplified during the process, enhancing the technique's power and accuracy.
Key Differences from Conventional PCR
When comparing reverse PCR to conventional PCR, several key differences should be noted. First, unlike conventional PCR, which starts with DNA as a template, reverse PCR requires RNA samples. This distinction is fundamental as it enables the analysis of gene expression and the study of various RNA transcripts.
Another difference lies in the role of reverse transcriptase. In reverse PCR, the enzyme is critical for synthesizing cDNA from RNA, whereas traditional PCR only uses DNA polymerase.
Other differences include:
- Target Input: Reverse PCR often requires RNA inputs, while conventional PCR uses existing DNA templates.
- Application Focus: Reverse PCR emphasizes studying RNA expression levels, making it suitable for functional genomics.
- Output Variability: The amplification in reverse PCR can sometimes yield results reflecting various RNA species, while traditional PCR generally produces straightforward amplification products.
Understanding these fundamental principles allows researchers and professionals to utilize reverse PCR effectively in their work. Through this technique, they can gain insights into molecular biology that may not be achievable with standard PCR methods.
Methodologies of Reverse PCR
The methodologies of reverse PCR are fundamental to understanding how this technique can be applied effectively in various contexts, including research and clinical settings. These methodologies provide essential insight into the necessary steps that researchers must follow to achieve reliable and reproducible results. Reverse PCR techniques are not just variations of standard PCR. They involve unique approaches that enhance our ability to manipulate and analyze DNA in sophisticated ways. In this section, we will explore the specific techniques involved in reverse PCR, shedding light on their significance and practical applications.
Sample Preparation Techniques
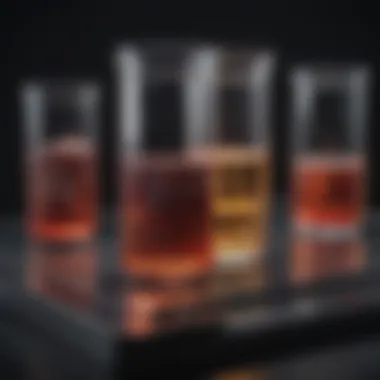
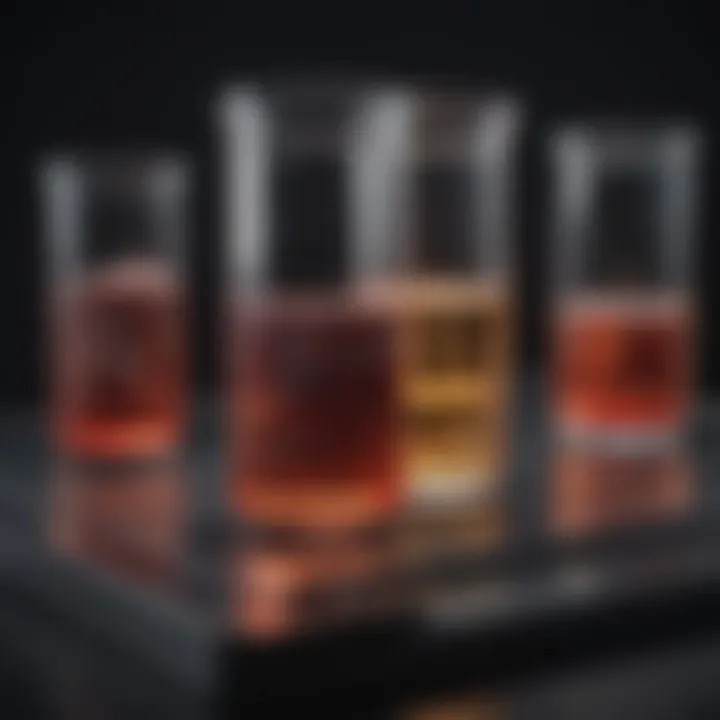
Sample preparation is a crucial phase in the reverse PCR process. A well-prepared sample can significantly influence the outcome of any molecular biology experiment. In reverse PCR, the initial step involves isolating the target nucleic acid, which often includes RNA or fragmented DNA. Researchers must use reliable methods such as:
- Column-based purification: This method ensures that only the desired nucleic acids are collected, while contaminants are removed efficiently.
- Silica gel extraction: A cost-effective technique for extracting DNA from samples, ensuring high purity levels.
- Magnetic beads: These provide a quick way to isolate nucleic acids while minimizing the risk of contamination.
Proper sample preparation sets the stage for successful enzymatic reactions and amplification procedures. Failure to prepare samples adequately can lead to inaccurate results and prolong experimentation time.
Enzymatic Reactions Involved
Enzymatic reactions are the heart of reverse PCR. They are responsible for synthesizing the complementary DNA from RNA and for any subsequent amplification of nucleic acids. The two primary enzymes used in reverse PCR are:
- Reverse transcriptase: This enzyme converts RNA into complementary DNA (cDNA). It is pivotal for analyzing gene expression since it allows researchers to study RNA indirectly by measuring the cDNA produced.
- DNA polymerase: This is essential in amplifying the cDNA during PCR. Different DNA polymerases vary in their fidelity and efficiency, influencing the speed of the reaction and overall yield of the product.
Each enzyme has specific requirements regarding buffer conditions and temperature that must be precisely controlled to ensure success. Understanding the role of these enzymes helps in optimizing the overall reverse PCR process.
Amplification Procedures
Amplification is where reverse PCR shows its strength. The process is similar to traditional PCR, but it focuses on the newly synthesized cDNA. The amplification protocol typically involves:
- Denaturation: The double-stranded cDNA is heated to separate it into single strands.
- Annealing: Specific primers bind to their complementary sequences on the cDNA.
- Extension: The DNA polymerase synthesizes the new DNA strand from the primers.
These steps are repeated for several cycles, usually 25-35 times, resulting in an exponential amplification of the target nucleic acid sequence. Success in amplification is highly dependent on optimal reaction temperature, primer design, and cycling parameters.
"The success of reverse PCR can often hinge on the details involved in the amplification procedures—meticulous attention to protocol can yield outstanding results."
By mastering these methodologies, researchers can leverage reverse PCR for a variety of applications, from gene expression studies to genetic mutation analysis, ultimately enhancing their capability in molecular investigations.
Applications in Research
The applications of reverse PCR in research are vast and critical for advancing genetic analysis. This technique allows for deeper insights into genetic mutations, gene expression, and even epigenetic mechanisms. Each of these areas can greatly benefit from the unique characteristics of reverse PCR, such as its high sensitivity and ability to amplify cDNA from RNA templates. Researchers rely on this technique not only for basic studies but also for applied sciences, including medical diagnostics and therapeutic developments.
Genetic Mutations Analysis
Reverse PCR plays a pivotal role in the analysis of genetic mutations. By amplifying specific regions of DNA, researchers can identify mutations linked to various genetic disorders. This technique enables the detection of insertions, deletions, and single nucleotide polymorphisms (SNPs) that may otherwise be challenging to pinpoint.
One significant advantage of reverse PCR in mutation analysis is its capacity to work with low quantities of DNA. This is particularly useful in clinical settings where samples may be limited. Furthermore, reverse PCR allows for the direct analysis of RNA, which is crucial in understanding mutations at the transcription level.
To illustrate:
- Identification of Disease-Related Mutations: Reverse PCR is essential for identifying mutations in genes associated with conditions like cystic fibrosis or sickle cell anemia.
- Tracking Inherited Mutations: Scientists can monitor how mutations are passed down through generations, aiding in hereditary disease research.
"The ability to explore genetic mutations using reverse PCR enables researchers to facilitate personalized medicine, tailoring treatments based on individual genetic profiles."
Gene Expression Studies
Gene expression studies are another vital area where reverse PCR proves its worth. The technique allows researchers to quantify the levels of specific RNA transcripts in a sample. Understanding gene expression is crucial for uncovering diseases caused by dysregulated genes.
Through reverse PCR, it's possible to derive complementary DNA (cDNA) from mRNA, allowing for precise measurement of gene expression levels. This is particularly useful for:
- Comparative Expression Analysis: By comparing gene expression in healthy versus diseased tissues, researchers can identify key changes that may contribute to disease progression.
- Normalization and Reference Genes: Reverse PCR allows for the use of reference genes to normalize expression data, providing more reliable results.
By utilizing reverse PCR, researchers gain insights into how genes respond to different stimuli, which is essential for developing new therapeutic approaches.
Epigenetic Research
Epigenetic research is increasingly important as scientists seek to understand how environmental factors can influence gene expression without altering the underlying DNA sequence. Reverse PCR helps investigate these complex mechanisms.
This technique allows for the study of various epigenetic modifications, such as DNA methylation and histone modification. Here are some applications within this field:
- Methylation Studies: Reverse PCR can amplify specific DNA regions to assess their methylation status, shedding light on gene regulation.
- Transcriptional Regulation: Researchers can explore how epigenetic changes affect transcription levels, directly linking environmental influences to gene expression outcomes.
Clinical Applications of Reverse PCR
The clinical applications of reverse PCR are critically important in the realm of modern medicine and diagnostics. As a powerful tool in molecular biology, reverse PCR allows for the detection and quantification of specific nucleic acid sequences, making it invaluable for various medical applications. It serves as a diagnostic aid that can provide insights into genetic disorders, infectious diseases, and even personalized treatment approaches. This section delves into the specifics of reverse PCR's clinical use, highlighting its techniques and benefits.
Diagnostic Techniques
Reverse PCR plays a pivotal role in diagnostic techniques, especially in identifying pathogenic organisms and genetic disorders. By amplifying specific DNA or RNA sequences, clinicians can detect the presence or absence of targeted genetic material. This is particularly significant for early diagnosis and treatment.
Some common scenarios where reverse PCR is used include:
- Viral Infections: It can identify viral genetic material in patient samples, providing rapid results for infections like HIV and Hepatitis.
- Bacterial Pathogens: Physicians can utilize reverse PCR to detect bacteria, which is crucial in cases of sepsis or other serious infections.
- Genetic Mutations: For patients with genetic disorders, reverse PCR aids in identifying mutations in genes, allowing for precise diagnosis and management strategies.
Overall, the efficiency and accuracy of reverse PCR in these diagnostic applications make it a significant step forward in clinical microbiology and genetic testing.
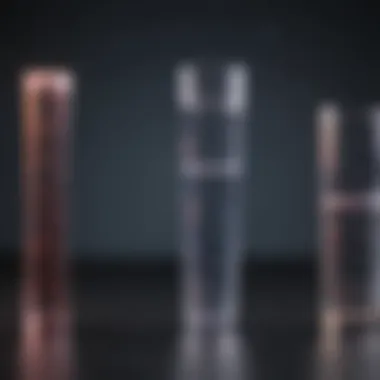
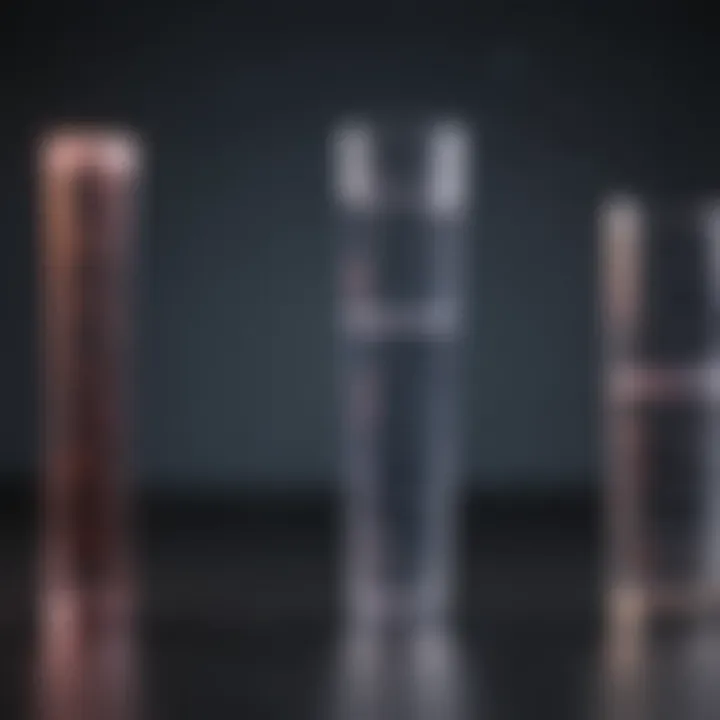
Strain Typing in Pathogen Detection
Strain typing is another significant utilization of reverse PCR in clinical settings. This method enables microbiologists to distinguish between various strains of pathogens, which is essential in outbreak management and understanding transmission dynamics of infectious diseases.
By analyzing specific genetic markers, reverse PCR allows for the classification of strains based on their unique genetic profiles. This application can lead to:
- Traceability: Identifying the source of infection in outbreak scenarios helps in controlling disease spread.
- Epidemiological Studies: Understanding the variations within pathogen strains contributes to research and public health policies.
- Vaccine Development: Knowledge of specific strains aids vaccine formulation against prevalent or emerging pathogens.
The precision provided by reverse PCR in strain typing enhances the ability to respond effectively to public health challenges.
Personalized Medicine Approaches
In the context of personalized medicine, reverse PCR is an essential tool. It supports the tailoring of medical treatments based on the genetic profile of individual patients. This approach not only improves treatment efficacy but also minimizes adverse effects by selecting the best possible course of action depending on a patient's unique genetic makeup.
Key aspects of personalized medicine utilizing reverse PCR include:
- Targeted Therapies: Identifying genetic mutations helps in selecting effective targeted medicines, particularly in cancer treatment where tumor genetic profiles dictate therapeutic efficacy.
- Pharmacogenomics: Understanding how a patient's genetic background affects drug metabolism allows for dosage adjustments or alternative drug selection.
- Preventative Strategies: Genetic predisposition assessments can inform screening practices and preventive measures in at-risk individuals.
Adopting reverse PCR in personalized medicine signifies a shift towards more individualized healthcare, aligning treatment with genetic insights for improved patient outcomes.
Advantages of Reverse PCR
Reverse PCR provides several significant advantages that make it a valuable technique in molecular biology. Understanding these benefits is crucial for researchers and practitioners aiming to optimize their genetic analysis methods. The following subsections elaborate on these advantages in detail.
High Sensitivity and Specificity
One of the primary strengths of reverse PCR is its high sensitivity and specificity. This technique allows for the detection of minute quantities of target nucleic acids within complex samples. High sensitivity is particularly important in scenarios where the amount of target DNA is low, such as in clinical diagnostics of infections or genetic mutations. Moreover, reverse PCR can differentiate between closely related sequences, reducing the chances of false positives.
In practice, the design of specific primers plays a crucial role in enhancing sensitivity. By ensuring that primers are complementary to the target region, researchers can minimize the amplification of non-target sequences. This property makes reverse PCR an ideal method for applications that require precise detection, such as in oncogenes studies. Furthermore, the ability to identify specific mutations or sequences directly contributes to improvements in disease diagnostics and management.
Capacity for Complex Input Samples
Reverse PCR exhibits a remarkable capacity for handling complex input samples. This characteristic is especially valuable when working with biological samples that contain various types of nucleic acids, such as RNA, DNA, and other cellular material. The method allows researchers to extract relevant genetic information from these intricate mixtures without extensive preprocessing.
For example, when examining environmental samples like soil or water, it is common to encounter diverse microbial communities. Reverse PCR's ability to differentiate and analyze specific genetic markers from such mixtures provides significant insights into biodiversity and environmental health.
Also, the technique can be used effectively with clinical samples, such as blood or tissue biopsies, which may contain a mixture of healthy and diseased cells. The robustness of reverse PCR allows for effective analysis of samples that may otherwise be too challenging for traditional PCR.
"The inherent sensitivity and specificity of reverse PCR make it a powerful tool for both clinical diagnostics and fundamental research."
Limitations of Reverse PCR
While reverse PCR offers significant advantages in genetic analysis, it is essential to acknowledge its limitations. Understanding these limitations allows researchers to make informed decisions when selecting techniques for their projects. The drawbacks of reverse PCR can impact the accuracy, reliability, and overall effectiveness of the results obtained.
Potential for Non-Specific Amplification
A considerable challenge in reverse PCR is the potential for non-specific amplification. Unlike conventional PCR, where primer specificity is relatively straightforward, reverse PCR employs primers designed to bind to their respective targets. However, these primers may also bind to similar sequences within the sample, resulting in non-specific products. Such amplification can lead to ambiguous results. This is particularly problematic when analyzing complex samples, as the presence of unintended products makes interpretation cumbersome. To mitigate this risk, optimizing the primer design is essential. Ensuring high specificity means considering factors such as melting temperature and secondary structures. Additionally, performing controls using known templates can help verify the specificity of the amplified products.
Technical Challenges in Protocol Optimization
Protocol optimization plays a vital role in the success of reverse PCR. Researchers often face technical challenges that can hinder the amplification process. For example, variations in reaction conditions—such as magnesium ion concentration, annealing temperature, and enzyme choice—can produce inconsistent results. These factors must be finely tuned to achieve the desired amplification without compromising specificity.
Moreover, the presence of inhibitors within the sample can significantly reduce the efficiency of reverse PCR. Compounds like polysaccharides or proteins may interfere with the enzymatic activity of DNA polymerases. Identifying and removing these inhibitors often require additional sample preparation steps, complicating the overall process. The necessity for meticulous protocol optimization demands considerable time and resources, which may deter some researchers from utilizing reverse PCR in their work.
Efficient optimization of the reverse PCR protocol is crucial for accurate and reproducible results.
Comparative Analysis with Traditional PCR
The comparative analysis between reverse PCR and traditional PCR is essential to understanding their respective roles in molecular biology. Both techniques serve the purpose of amplifying DNA, but they differ in their methodologies, efficiencies, and applications. Evaluating these differences can illuminate why researchers might opt for one approach over the other depending on their specific needs. The importance of this section lies in its potential to clarify when reverse PCR is more beneficial than its traditional counterpart and vice versa.
Efficiency and Throughput
One of the primary points of comparison between reverse PCR and traditional PCR is efficiency. Traditional PCR is well-known for its ability to amplify known sequences, but it can struggle with complex samples where target and non-target DNA are closely related. Reverse PCR shines in this aspect. Its design allows for the amplification of sequences even when the target DNA is present in low concentrations or is part of a complex mixture. This capability significantly improves sensitivity.
In terms of throughput, reverse PCR can adapt more readily to high-throughput settings. This is especially relevant in environments such as genomics laboratories where vast amounts of samples need to be processed quickly. By employing reverse PCR in a high-throughput manner, laboratories can conduct numerous analyses simultaneously without compromising the quality of amplification. This potential for increased throughput also leads to cost-effectiveness over time.
Data Interpretation and Results Comparison
Data interpretation stands as another critical factor in comparing reverse PCR with traditional PCR. The outputs of reverse PCR can produce results that are more straightforward to analyze, particularly when it comes to identifying mutations or understanding gene expression levels. Traditional PCR results can sometimes require additional steps to differentiate between specific amplified products, whereas reverse PCR minimizes ambiguity through its method of targeting specific sequences.
Another consideration is the robustness of the comparative results. In a scenario where both methods are used to analyze the same genetic sequence or mutation, reverse PCR may yield clearer and more consistent results. This reproducibility can have significant implications for research validity and reliability.
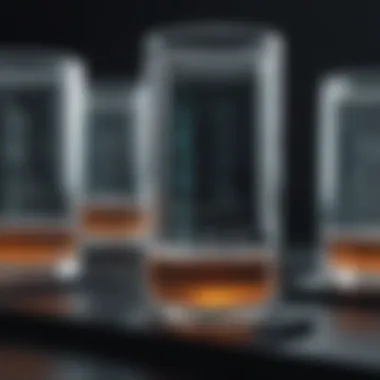
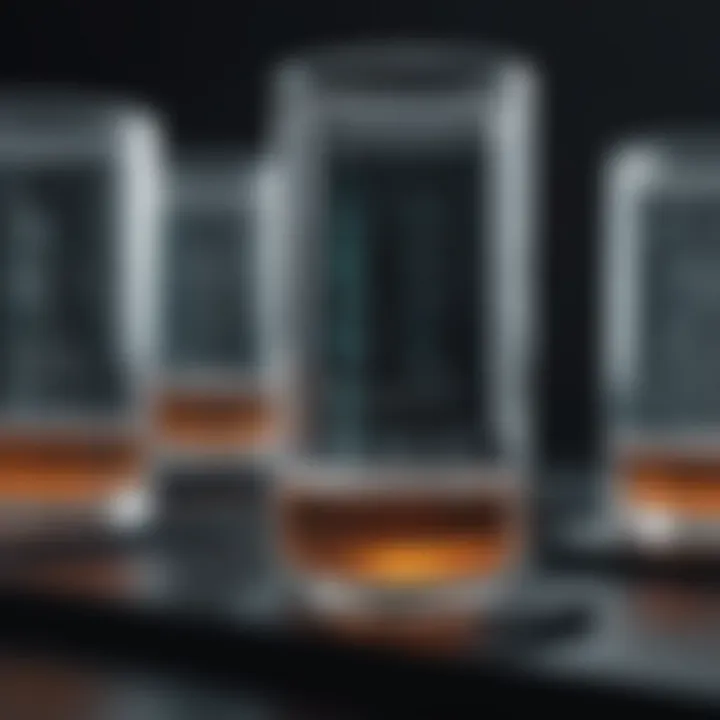
In summary, understanding efficiency, throughput, and data interpretation when comparing reverse PCR with traditional PCR highlights the advantages that reverse PCR can bring to certain applications. The specific elements discussed here not only benefit researchers in their methodological choices but also underscore the importance of adapting these technologies to suit new challenges in genetic analysis.
Future Directions in Reverse PCR
Future directions in reverse PCR present a significant area of interest for researchers. As advancements in technology continue to evolve, the significance of improving the protocol of reverse PCR cannot be overstated. This is crucial for a variety of applications ranging from academics to clinical practices. Increased accuracy, efficiency, and the ability to analyze more complex samples are some of the key benefits anticipated from ongoing improvements. These developments could refine the methods for genetic testing and diagnostics, leading to more effective health management and genomic research strategies.
Technological Innovations
Technological innovations stand at the forefront of future directions for reverse PCR. Emerging tools and equipment are likely to enhance the precision and speed of reverse PCR processes. For instance, next-generation sequencing, advances in bioinformatics, and automation technologies can play pivotal roles. High-throughput systems can enable scientists to conduct a greater number of tests simultaneously, thus significantly reducing the work time involved. Furthermore, newer reagents may lead to superior specificity and sensitivity, addressing existing limitations in conventional protocols.
One exciting development is the potential application of CRISPR technology within reverse PCR methodology. By integrating CRISPR, researchers may be able to enhance the targeting of specific genetic sequences, thereby increasing both the sensitivity and robustness of the assays. Additionally, portable devices for rapid PCR tests are also gaining interest, which can facilitate on-site testing for various applications.
Potential New Applications
Potential new applications of reverse PCR could extend beyond genetic analysis and into areas such as environmental monitoring and food safety analysis. By utilizing reverse PCR, scientists can better detect genetically modified organisms and pathogens in food products. This can be crucial for ensuring food safety and maintaining public health standards.
In clinical settings, reverse PCR may become integral in identifying rare diseases that have a genetic component. It can also be applied in cancer research to identify mutations specific to different tumor types, thus aiding in more personalized treatment strategies. The emergence of liquid biopsies, which require minimally invasive methods, may benefit significantly from reverse PCR techniques as well.
In summary, as reverse PCR continues to evolve, embracing fresh technologocal advances and identifying new applications will lead to more effective and diverse uses in various sectors.
"The future of reverse PCR lies significantly in harnessing technology to improve not just its accuracy, but also its accessibility and range of use in the scientific community."
Ethical Considerations
In the realm of molecular biology and genetic testing, ethical considerations play a crucial role. Reverse PCR testing, as a technique, can elicit a range of ethical queries. These questions revolve around the implications of genetic analysis and how it may affect individuals and populations. One must recognize the need for maintaining transparency, confidentiality, and consent throughout the process of genetic testing using reverse PCR.
Impact on Genetic Testing
Genetic testing can enhance medical decisions. The power of reverse PCR lies in its ability to confirm or deny genetic mutations. This can directly influence treatment options for patients. However, it is vital to approach these decisions with care. Potential implications arise if a genetic predisposition to certain conditions is revealed. Patients must be fully informed about what the results signify. Additionally, ethical dilemmas can occur regarding the use of this data. Concerns often relate to privacy and how the genetic data might be shared or misused. This emphasizes the importance of strong ethical guidelines in the implementation of genetic testing protocols.
Use in Clinical Settings
In clinical settings, reverse PCR can prove invaluable for diagnostics. It assists in detecting specific pathogens or genetic markers. Still, the ethical dimension cannot be overlooked. Patients often rely on clinicians to understand their genetic information. Therefore, it is the responsibility of healthcare providers to offer consultations that respect the patients' sense of autonomy while providing accurate and easily understood information. Furthermore, issues about potential discrimination arise, especially in employment or insurance scenarios if genetic information is mishandled. Establishing clear policies regarding the use of genetic testing results can help mitigate these risks, ensuring that individuals are not unfairly disadvantaged due to their genetic makeup.
"Ethics in genetic testing is not just about compliance; it is about fostering trust and safeguarding individual rights."
Navigating ethical issues in reverse PCR usage requires a commitment to ethical principles that prioritize the safeguarding of patient rights. This is critical, as the advancement of testing technologies introduces added complexity to ethical discussions.
Best Practices in Reverse PCR Protocols
Implementing effective reverse PCR protocols is crucial for achieving accurate and reliable results. These best practices help to minimize errors and optimize the performance of the technique, which is essential for successful genetic analysis. Ensuring that the procedures are followed correctly can vastly increase the sensitivity and specificity of the test, thus avoiding misinterpretations of the data. Here, we explore the recommendations that should be prioritized in reverse PCR applications.
Troubleshooting Common Issues
In reverse PCR, several common problems can arise that may interfere with the intended outcomes. Here are a few prevalent issues and their solutions:
- Non-specific amplification: This problem often occurs due to suboptimal primer design or incorrect annealing temperatures. When primers bind to unintended targets, it can lead to erroneous results. To combat this, redesign the primers and optimize the annealing temperature, which should be tested systematically.
- Low yield of targeted products: Low amplification can happen when the sample contains insufficient template DNA or when reaction conditions are not ideal. Increasing the quantity of the template and optimizing enzyme concentrations can improve yield.
"Implementing troubleshooting measures can save substantial time and resources while enhancing the quality of data derived from reverse PCR tests."
- Contamination: It's crucial to maintain an uncontaminated work environment to prevent cross-contamination that can lead to false positives. Employseparate work areas for pre- and post-PCR activities and use barriers like pipette tips with filters. ]
- Inconsistent results: Variations can occur due to operator error or differences in reagents. To minimize these inconsistencies, always follow standardized protocols and conduct multiple replicates of each sample to confirm results.
Standardization of Procedures
Standardizing reverse PCR protocols can significantly enhance the reproducibility of results. The development and implementation of standard operating procedures (SOPs) ensure that all aspects of the testing process are consistent across different laboratories and experiments. This standardization can lead to more reliable interpretations of genetic data. Consider the following elements for successful standardization:
- Reagent quality: Utilize high-quality reagents sourced from reputable suppliers to maintain consistency between experiments.
- Equipment calibration: Ensure that all equipment used in reverse PCR, such as thermocyclers and pipettes, is properly calibrated and maintained. Regular checks can prevent technical variations that might affect outcomes.
- Detailed documentation: Keeping thorough records of every aspect of the PCR process, including reaction conditions, volumes, and times, allows for effective tracking of performance and helps in pinpointing any deviations that may arise.
- Training and protocols: Having a clear training program for laboratory personnel ensures that everyone is familiar with the protocols and adheres to the same techniques, reducing variance in procedure execution.
Closure and Summary
In any scientific discourse, the conclusion serves as a pivotal component. It is where the key insights achieved throughout the exploration of the subject come together. In this case, the final thoughts on reverse PCR provide a structured reflection on its significance in modern molecular biology. Reverse PCR offers not merely a technique for genetic testing but also a versatile tool that is reshaping research and clinical practice.
The recap encapsulates the essence of this article’s extensive coverage. Readers will find that reverse PCR plays a crucial role in areas such as genetic mutation analysis, gene expression studies, and applications in personalized medicine. Understanding the methodologies and limitations of this technique fosters better implementation and optimization within laboratory settings. For students and professionals alike, grasping these elements can enhance their research capabilities and expand their analytical frameworks.
Overall, reverse PCR is significant not only for its applications but also for its contributions to the larger field of genetic study and related areas. Innovations in this domain open new pathways for scientific inquiry and discovery.
Recapitulation of Key Points
- Definition and Scope: Reverse PCR is a refined approach within the polymerase chain reaction, optimized for amplifying reverse-complemented DNA.
- Principles and Mechanisms: Understanding the core principles helps clarify how this technique differs from traditional methods and its potential productivity.
- Applications: The use in diagnostics and personalized medicine highlights its current relevance and future prospects in healthcare and research.
- Advantages and Challenges: Recognizing both high sensitivity and specific amplification techniques, along with their pitfalls, is vital for any practitioner.
- Future Directions: Anticipating technological innovations allows researchers to remain at the forefront of developments in molecular biology.
Final Thoughts on the Future of Reverse PCR
As the landscape of molecular biology evolves, reverse PCR stands poised for expansion. Its adaptability is one of the most compelling attributes. The integration of new technologies such as CRISPR could enhance its utility even further. Applications in epigenetic research or even plant genetics are potential frontiers that researchers are beginning to explore.
Investment in revising and refining protocols will be essential in addressing the current limitations of reverse PCR, particularly regarding non-specific amplification. As labs continue to standardize procedures, the reliability of the data generated from reverse PCR will only increase.
- Continuous Innovation: The future may bring novel methodologies and improvements in he sensitivity of detection in reverse PCR.
- Broader Applications: Potential new applications may arise, integrating interdisciplinary approaches, influencing fields from forensics to agriculture.
Thus, reverse PCR not only meets the current needs in genetic analysis but is also likely to adapt to emerging scientific challenges, ensuring its role as a cornerstone of genetic research in the future.