Understanding Insulator Bands: Implications & Applications
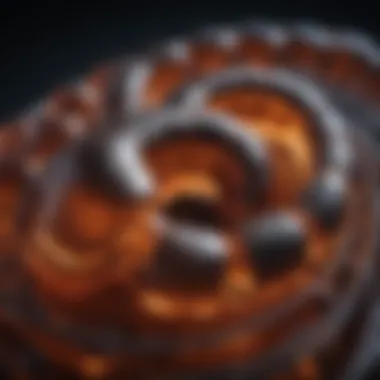
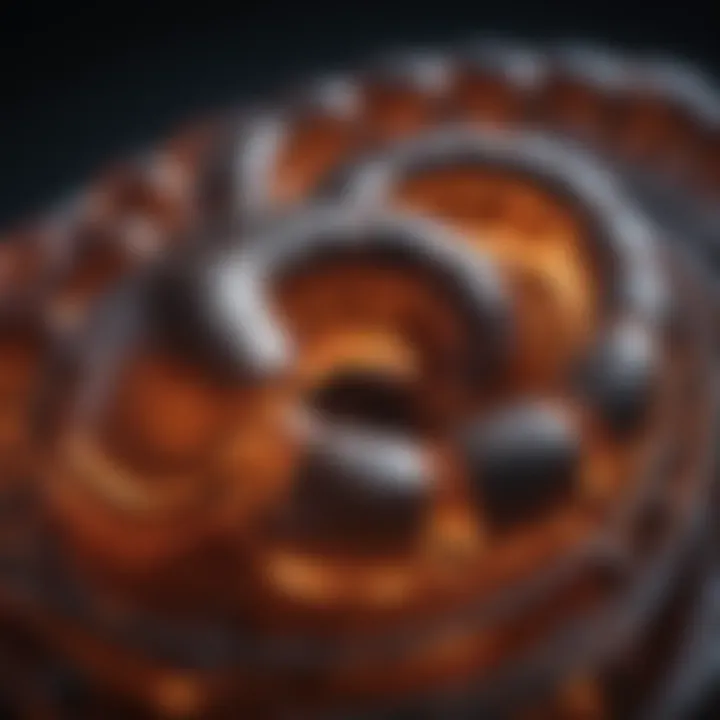
Intro
The study of insulator bands has become increasingly important in solid-state physics. This area of research not only helps to explain the behavior of various materials but also has significant implications for modern technology. Understanding insulator bands is essential for advancing electronic devices and improving energy transfer mechanisms. Researchers in the field are delving deeper into these concepts to uncover new possibilities.
Overview of Research Topic
Brief Background and Context
Insulator bands are a fundamental aspect of solid-state physics. They occur in materials that do not conduct electricity under normal conditions. These bands are characterized by a gap between the valence band and the conduction band. This gap determines the electrical behavior of the material. The study of these bands involves examining how their formation affects the material's properties.
Importance in Current Scientific Landscape
In today's scientific landscape, insulator bands play a crucial role. They impact a wide range of technologies, particularly in electronics. As the demand for more efficient and effective devices increases, understanding insulator bands becomes even more vital. Ongoing research aims to manipulate these bands to enhance the performance of various applications, from semiconductors to insulator-based electronics.
Methodology
Research Design and Approach
The methodological framework for studying insulator bands often involves both theoretical and experimental approaches. Simulation techniques such as density functional theory are used to analyze the electronic structure of materials. Experimental techniques, including spectroscopy, help to validate these theoretical predictions.
Data Collection Techniques
Data collection in this field typically involves gathering results from both computational models and experimental setups. Measurements often include electrical conductivity, and optical properties. These results contribute to a comprehensive understanding of how insulator bands function in real-world applications.
Prelims to Insulator Bands
In the domain of solid-state physics, insulator bands hold significant importance. Understanding them is pivotal for advancing technology in electronics and beyond. Insulator bands represent the range of energies that electrons cannot occupy in insulating materials. This concept is foundational in discerning the behavior of various materials in both practical applications and theoretical explorations.
One major aspect of insulator bands involves their crucial role in energy transfer mechanisms. These bands determine how materials conduct or prevent electrical charge flow. This understanding is vital for developing efficient devices for computing and communication.
Moreover, insulator bands serve as a basis for exploring new materials. Advances in this field allow researchers to manipulate these bands through techniques such as doping and structural engineering. These manipulations enhance material capabilities in devices, leading to better performance and new functionalities.
The implications extend further into energy storage systems as well. Unique properties of insulator bands can influence how energy is stored and transferred, which is key for innovations in renewable energy technologies.
Definition of Insulator Bands
Insulator bands are characterized by the energy levels that are forbidden for electrons to occupy. In simpler terms, they denote the gap in energy levels that exists between the occupied electronic states and the unoccupied states in a material. These bands affect the behavior of materials when exposed to electric fields or thermal energy. In insulators, the band gap is large enough that electrons cannot transition to the conductive band, preventing conduction under normal conditions.
This behavior differentiates insulators from conductors and semiconductors, where electrons can bridge this gap under specific conditions. Understanding insulator bands is thus essential for examining the conductive properties of materials.
Historical Context and Development
The study of insulator bands dates back to the early 20th century when quantum mechanics began to illuminate the behavior of electrons in solids. Early theories laid the groundwork for understanding how energy levels are distributed in materials. One significant contributor to this field was Paul Dirac, whose work in quantum physics provided insights into electronic structures.
As research advanced, scientists like Walter Heitler and Fritz London contributed to the understanding of band theory, elucidating the nature of bonding and antibonding states in solids. These early developments formed the basis for more detailed investigations into how insulator bands influence electrical and thermal properties in materials.
In recent decades, the emergence of new materials and fabrication techniques has opened fresh avenues for research. Materials such as perovskites and 2D materials, like graphene, demonstrate unique insulation properties, prompting further exploration into their band structures. As research continues, the definition and application of insulator bands evolve, indicating their relevance in both theoretical and practical contexts.
The progress in understanding insulator bands has implications not just for academic research but also for practical applications in various fields of technology.
Fundamental Principles of Insulation
Understanding the fundamental principles of insulation is essential for grasping how insulator bands function in various materials. Insulation is crucial in controlling electrical and thermal conductivity, allowing materials to function effectively in electronics, energy storage, and thermal applications.
Band Theory Overview
Band theory forms the backbone of our comprehension of semiconductor and insulator behaviors. According to this theory, atoms form energy bands, which are ranges of energy levels that electrons can occupy. These bands include the valence band, where electrons are usually found, and the conduction band, where electrons can move freely, allowing conductivity. An energy gap, or band gap, separates these bands in insulators.
In insulators, the band gap is wide enough that electrons cannot easily jump from the valence band to the conduction band under ordinary conditions. This results in their insulating properties. Understanding the specifics of band theory provides insight into why certain materials resist the flow of electric current while others permit it.
Energy Bands and Band Gaps
Energy bands and band gaps are critical concepts in understanding the insulating capability of materials. In insulators, the lower energy states are densely populated, and higher states are empty. This configuration is essential for insulation because it prevents electron flow under normal circumstances.
The size of the band gap is indicative of a material's insulating properties. Insulators boast large band gaps, typically greater than 4 electronvolts (eV). For example, materials like quartz and rubber exhibit significant band gaps, resulting in their effectiveness as insulators in various applications.
Compared to semiconductors, which have narrower band gaps (usually around 1-3 eV), insulators require much higher energy input for electrons to move into the conduction band. This distinction is essential for developing electronic components that rely on predictable insulating behaviors.
In summary, the principles of band theory and the characteristics of energy bands and band gaps form the foundation of our understanding of insulator bands. Delving into these fundamental aspects enhances the comprehension of their impacts on technology and innovation.
Formation of Insulator Bands
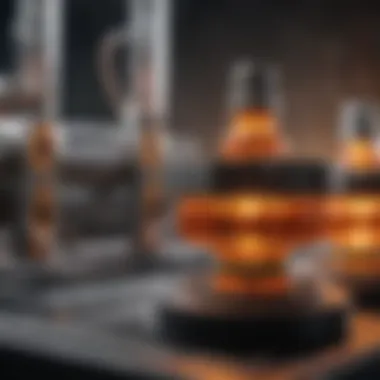
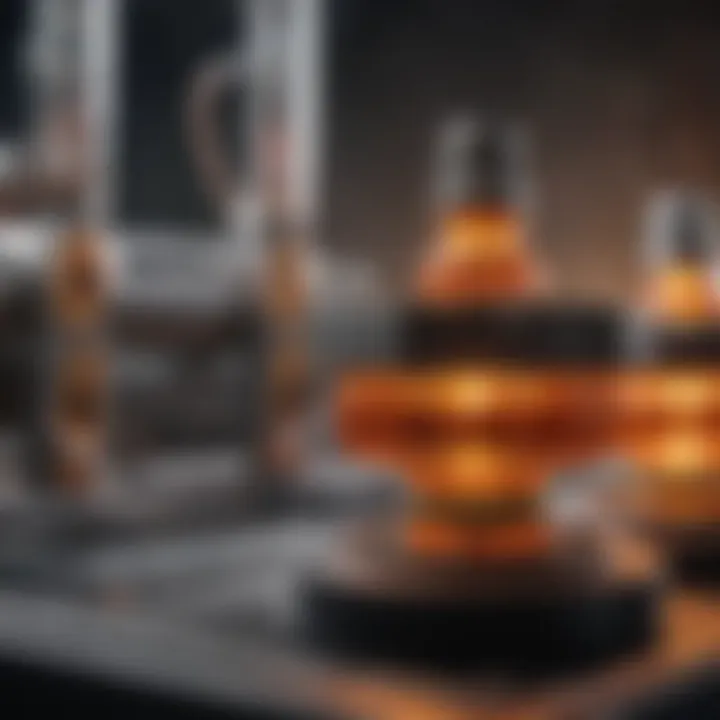
The formation of insulator bands is a critical aspect of understanding electrical and thermal properties of materials. This topic sheds light on how certain materials are classified as insulators and the fundamental reasons behind their unique characteristics. By grasping the formation of insulator bands, researchers and professionals can better predict material behavior in various applications, especially in electronics and energy systems. The electron configuration, defects, and impurities play significant roles in determining the effectiveness of insulators in different environments.
Electron Configuration in Insulators
In insulators, electron configuration is a determining factor for their properties. Electrons are arranged in specific energy levels or shells, which define how they interact with external energy. In insulators, the outermost electrons are tightly bound to their atomic cores due to large band gaps between the valence band and the conduction band. This architectural layout means that it requires substantial energy to liberate an electron for conduction.
For instance, materials like silicon dioxide or alumina exhibit a stable electron configuration that reinforces their insulating qualities. Their valence bands are filled, while their conduction bands remain empty at room temperature. The high energy requirement to move electrons into the conduction band prevents the flow of electric current, making these materials excellent insulators.
The significance of electron configuration cannot be overstated. It not only informs us about a material’s ability to conduct electricity but also indicates thermal behaviour. As electrons remain bound within their positions, insulators often exhibit low thermal conductivity.
Role of Impurities and Defects
Impurities and defects present in a material can greatly alter the formation of insulator bands. These unintentional additions can change the electronic structure, leading to either enhanced or diminished insulating properties. For example, when atoms foreign to the host lattice are introduced, they can create localized states within the band gap.
If these states are close enough to the conduction band, they may allow some electrons to move freely, compromising the insulating property.
- Common impurities include:
- Cation substitution: When one type of metal ion is replaced by another in the crystal lattice.
- Anion vacancies: Absence of an anion which can disrupt the charge balance.
Additionally, defects such as dislocations or grain boundaries may create pathways for electrical conduction. Understanding these factors is essential for material scientists looking to optimize insulation for electrical components or thermal barriers in high-performance products.
"Impurities and defects can either enhance or decrease the insulating properties, depending on their nature and concentration."
To sum up, the formation of insulator bands is influenced by intrinsic electron configurations and extrinsic factors such as impurities and defects. This comprehensive understanding is crucial for advancing material applications in tech-driven industries.
Characteristics of Insulator Bands
Understanding the characteristics of insulator bands is crucial in solid-state physics and materials science. These properties define how insulators behave under various conditions and their interactions with electrical and thermal energy. Two primary aspects are essential: the electrical properties and thermal properties.
Electrical Properties
Insulator bands exhibit distinct electrical properties that differentiate them from conductors and semiconductors. A significant characteristic is their high resistivity, which limits the flow of electric current. This is due to the large band gap present, typically exceeding three electron volts. This gap prevents electrons from freely moving under an applied voltage.
The insulator's ability to resist electric flow makes it vital for numerous applications, particularly in electronics and telecommunications. For instance, materials such as silica and ceramics are widely used for substrates and housing in electronic devices. Their insulating nature protects sensitive components from unwanted current and interference.
Also, the dielectric strength of insulator bands contributes to how they perform under high electric fields. A high dielectric strength can reduce the risk of breakdown, ensuring reliability in electrical applications.
Some additional electrical properties to consider include:
- Charge Carrier Dynamics: In insulators, charge carriers (like electrons) are minimal, limiting conductivity.
- Field Dependence: Insulator behavior can change under varying field strengths, affecting their applications in capacitive systems.
- Polarization Effects: Insulating materials often exhibit polarization when exposed to electric fields, influencing their behavior in real-world applications.
Insulating materials form the backbone of safe electronic design, providing essential stability and performance.
Thermal Properties
The thermal properties of insulator bands further illustrate their significance in various functions. Insulators play a vital role in thermal management, helping to maintain the performance of electronic devices. They have low thermal conductivity, which limits heat transfer between components. This characteristic protects sensitive electronic parts from overheating, significantly extending the lifespan of devices.
Insulator materials like polymeric composites are valuable for thermal insulation in both consumer and industrial applications. They ensure that systems operate within their desired thermal limits, especially in energy-sensitive applications like energy storage systems.
Key thermal characteristics include:
- Thermal Stability: Good insulators can maintain performance under temperature fluctuations.
- Heat Capacity: High heat capacity can be beneficial for materials that need to absorb and dissipate heat slowly.
- Thermal Expansion: Insulator materials often have low thermal expansion coefficients, reducing the risk of stress or failure during heating.
Applications of Insulator Bands
The realm of insulator bands is integral to modern technologies, particularly in electronics and energy storage. Understanding the applications of these bands provides valuable insights into material selections and innovative designs. Insulator bands help define how materials manage electrical and thermal properties.
Insulating Materials in Electronics
Insulating materials play a pivotal role in electronic devices. They represent a barrier for electrical current, ensuring that it flows only through designated paths. The semiconductor industry leverages various insulating materials to enhance device performance. Common insulators include silicon dioxide, aluminum oxide, and polymers like polyimide.
Using insulator bands, engineers can develop components with reduced signal loss. This is particularly vital in high-frequency applications, where unwanted capacitance can degrade performance. Insulators are also crucial in preventing short circuits, which can lead to device failure. Proper selection of insulating materials contributes to miniaturization in electronics, as thinner insulators occupy less space while maintaining effectiveness.
Key benefits include:
- Enhanced reliability in devices
- Improvement in energy efficiency
- Minimization of cross-talk between components
The effectiveness of these materials depends on their band gap characteristics. A larger band gap typically indicates better insulating properties. Research continues into new materials with improved characteristics to meet the growing demands of the electronic industry.
Use in Energy Storage Systems
Energy storage systems, such as batteries and capacitors, also rely on insulator bands. Insulators are essential in maintaining stability and performance in these systems. For instance, lithium-ion batteries utilize insulating materials for separators, which prevent internal short circuits. This allows for efficient energy storage and delivery.
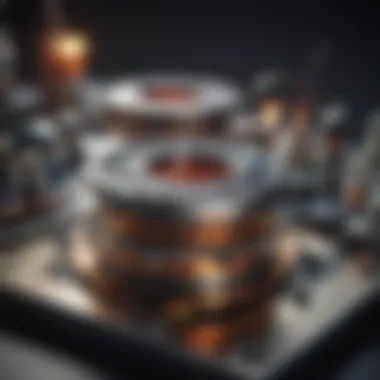
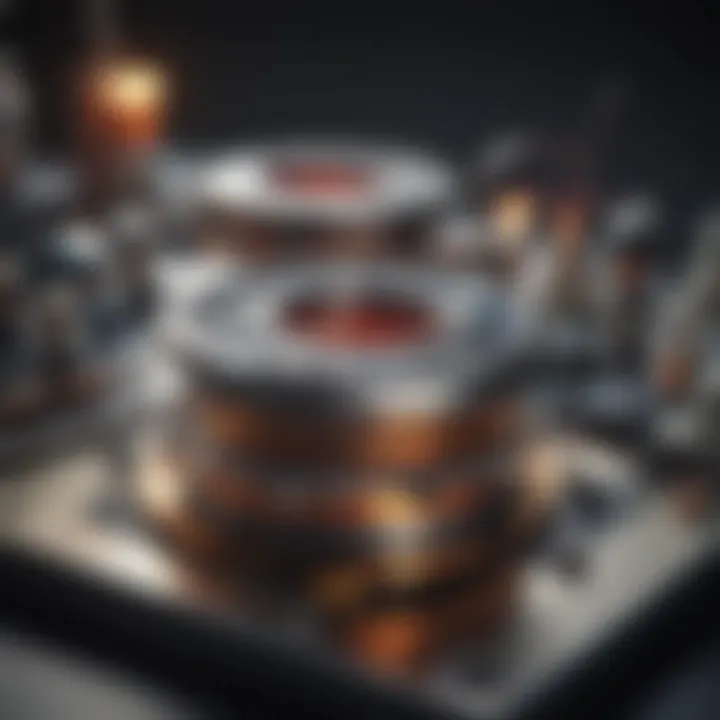
The role of insulators extends to preventing unwanted reactions that can occur in high-energy environments. In supercapacitors, insulator bands help optimize the energy storage capabilities by enhancing the dielectric properties. This leads to increased energy density and improved performance.
Additionally, in the context of renewable energy, the development of insulative materials can facilitate better integration of energy storage solutions. Efficient insulators can enhance the durability and longevity of these systems, leading to sustainable practices.
In summary, the application of insulator bands in electronics and energy storage systems underscores their significance in modern technology. Their efficient use ensures operational stability, longevity, and performance, making them essential components in ongoing technological advancements.
Impact on Modern Technology
The impact of insulator bands on modern technology cannot be emphasized enough. Understanding how these bands function provides insight into the development of advanced electronic devices. Insulator bands determine how materials behave in electronic applications, influencing both performance and efficiency. Researching insulator bands enhances our comprehension of electrical properties in materials, which can lead to innovations in the field.
Role in Semiconductor Devices
Semiconductor devices rely heavily on insulator bands. The presence of these bands defines how semiconductors conduct electricity. Insulator bands create an energy barrier that affects electron movement. In devices like transistors and diodes, this behavior is crucial.
- Enhancement of Performance: Insulator bands facilitate better control over the flow of electrons. This results in improved switching speeds and energy efficiency.
- Miniaturization of Devices: As technology advances, there is a constant push to create smaller devices. Insulator bands allow for tighter integration of circuits, leading to more compact semiconductor devices.
Achieving balance in the band gap design is key. A narrower band gap can lead to higher conductivity, but may also result in more instability. Thus, careful engineering of insulator bands is fundamental for the innovation of reliable semiconductor technology.
Advancements in Insulating Composites
The use of insulating composites is expanding, driven largely by advancements in technology. These materials, which combine different components, optimize the insulating properties. The role of insulator bands in these composites helps in minimizing thermal and electrical conductivity.
- Improving Material Properties: Composites like silicone and epoxy resin exhibit enhanced insulating properties. Insulator bands within these materials dictate their thermal stability and resistance.
- Broadening Application Scope: The versatility of insulating composites means their use extends across various industries. From electronics to construction, understanding insulator bands gives insights into new applications.
"Insulator bands are not just barriers; they are also gateways to innovation in materials science."
Research Trends in Insulator Bands
The exploration of insulator bands has gained significant attention in recent years. As technology advances, understanding the nuances of insulator behaviors becomes fundamental. Research trends in this area focus on unveiling the complexities that govern insulator properties. This section discusses emerging materials, innovations associated with band gap engineering, and the implications these developments have on technology.
Emerging Materials and Technologies
Recent research highlights the need for innovative materials that can be harnessed in various applications. Among these materials are perovskites, which are explored extensively for their electronic properties.
These materials present unique characteristics, such as high defect tolerance and tunable band gaps. Research suggests their potential in photovoltaic applications and solid-state lighting. More than just their insulating properties, the adaptability of perovskites opens avenues for hybrid devices that blend insulation with other functionalities.
In addition, two-dimensional materials, like transition metal dichalcogenides, are making waves in insulator band studies. These materials showcase how confinement at nanoscale changes their electrical properties. Researchers are keen on determining how these two-dimensional insulators can function effectively at room temperature as well as in extreme conditions.
For instance:
- Perovskites: High efficiency in solar cells.
- Transition metal dichalcogenides: Potential for low-power electronic devices.
These trends underline a movement toward sustainability and efficiency in materials science. Researchers concentrate on not just finding new materials but also processing techniques that are less energy-intensive.
Innovations in Band Gap Engineering
Band gap engineering represents a frontier in insulator band research. The control of the band gap is crucial since it dictates the conductivity and suitability of materials for various applications. Researchers now employ several techniques to modify the band gap of materials, allowing for the development of devices that operate under specific conditions.
One notable approach includes alloying different materials to achieve desired electronic characteristics. This method enables the customization of properties for specific applications in electronics or optoelectronics.
Additionally, the advent of advanced computational methods allows scientists to simulate and predict the outcomes of band gap adjustments before conducting experiments. This predictive capability accelerates the innovation cycle and minimizes resource usage.
"Advancements in band gap engineering not only refine existing technologies but also pave way for novel applications in areas ranging from energy storage to electronic devices"
These innovations signal a transformative phase in material science where creativity meets precision science. The ongoing research holds promise not just for academic advancement but practical applications that could revolutionize how we interact with technology.
Theoretical Modeling and Simulations
Theoretical modeling and simulations are essential in the study of insulator bands. This branch of research provides a framework to understand the complex behaviors of electronic materials. As insulator bands play a critical role in determining the properties of materials, modeling their behavior helps researchers predict how materials will perform in various applications. The usage of computational techniques allows for the exploration of materials that might be too costly or impractical to synthesize in a laboratory setting.
The importance of these simulations lies in several key areas:
- Predictive Power: They allow for predictions of material behavior under different conditions. This can save time and resources in experimental efforts.
- Accessibility of Data: Theoretical models provide data about properties that may be difficult to measure directly, including electronic structure and band gaps.
- Guiding Experimentation: Simulation results guide experimentalists in focusing their efforts on potentially fruitful areas of research. This synergy between theory and experiment accelerates advancements in the field.
Density Functional Theory Applications
Density Functional Theory (DFT) is a pivotal tool in materials science for understanding insulator bands. DFT calculates the electronic structure of materials based on the density of electrons rather than the many-body wave function. This makes it computationally feasible to study large systems and complex materials.
DFT's applications include:
- Band Structure Calculations: DFT can determine band gaps accurately, providing insight into whether a material is a conductor, insulator, or semiconductor.
- Study of Defects: It helps in understanding how defects in a material can alter its electronic properties and contribute to its insulating behavior.
- Thermodynamic Properties: DFT also aids in predicting properties like enthalpy and free energy, essential for thermodynamic analysis of materials.
Computational Methods in Materials Science
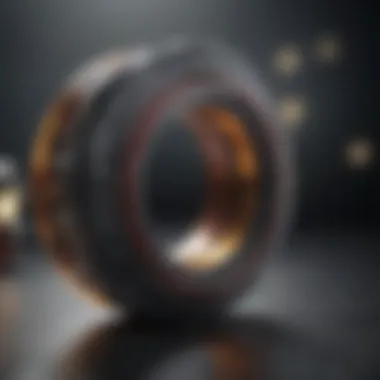
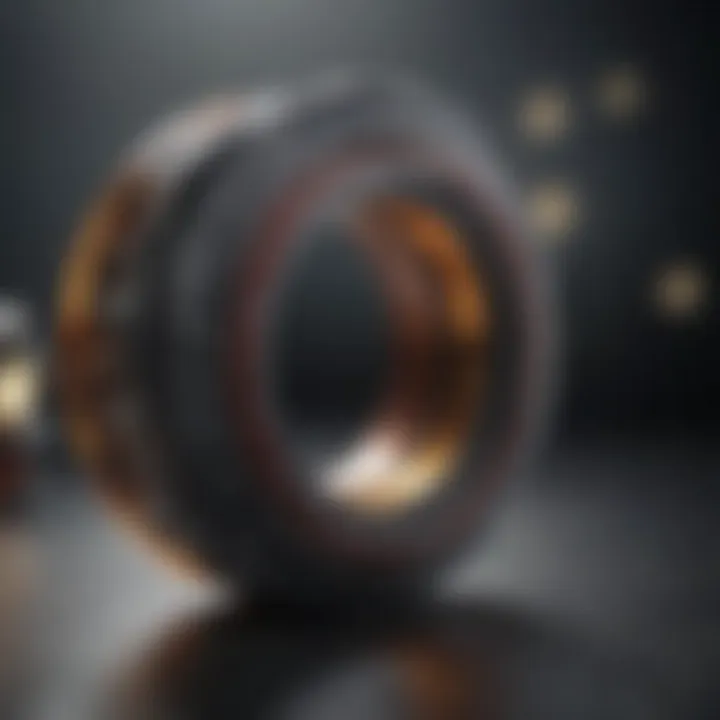
Multiple computational methods supplement the theoretical understanding of insulator bands in materials science. These techniques are instrumental for analyzing different aspects of insulator behavior.
Some critical computational methods include:
- Molecular Dynamics (MD): This technique simulates the motion of atoms over time, providing insights into how thermal fluctuations affect insulator properties.
- Monte Carlo Simulations: Useful for studying systems at thermal equilibrium. These simulations can explore the configurational space of materials and offer statistical insights into their properties.
- Tight-binding Models: These simplified models focus on the interactions between nearest neighbors. They provide good estimates of electronic properties while remaining computationally efficient.
In summary, theoretical modeling and simulations are indispensable in exploring the implications and applications of insulator bands. The integration of various computational methods with experimental approaches fosters a deeper understanding of materials, potentially leading to groundbreaking advancements in technology.
Challenges in Insulator Band Research
Research into insulator bands presents a myriad of challenges, which can significantly influence advancements in both theoretical and applied physics. Understanding these challenges is crucial as they inform the development strategies aimed to enhance material performance in various applications, especially in electronics and energy systems. Exceptions and limitations inherent to current research methodologies can obscure insights into the fundamental behaviors of insulators.
Limitations of Current Models
The current models employed in studying insulator bands often fall short in capturing the complexity of real-world materials. One significant limitation is the oversimplification of physical parameters. Standard band theory assumes ideal conditions that do not always align with experimental observations. This disconnect leads to inaccuracies in predicting how certain materials behave under various conditions.
Additionally, many models do not adequately account for the role of electron interactions. Correlation effects can significantly influence the properties of insulating materials, yet are frequently overlooked in basic theoretical frameworks. As a result, predictions made using these models may not reflect the true characteristics of the materials being studied.
Researchers are thus in need of more sophisticated approaches. Incorporating multiscale modeling techniques can potentially mitigate some of these discrepancies and offer a fuller picture of insulator behavior. Continuous refinement of existing models, combined with the exploration of new methodologies, is essential for advancing the current understanding of insulators.
Understanding Complex Crystal Structures
Complex crystal structures pose another significant challenge in insulator band research. While simpler lattices are often studied, real materials usually exhibit a degree of disorder and complexity that complicates the analysis. Such complexities arise from factors like impurities and defects that can significantly alter band structures. Understanding how these elements affect properties is crucial for both theoretical insights and practical applications.
Research often struggles with accurately modeling these intricacies. Traditional methodologies may not adequately capture the implications of nanoscale behaviors or the effects of local environments. For example:
- Defects can introduce localized states within the gap, affecting electronic transport and overall material performance.
- Disorder from impurities can disrupt periodicity, leading to unexpected changes in conductivity and thermal stability.
To address these issues, researchers are increasingly focused on developing advanced computational techniques, such as density functional theory. Such methods can better account for variations in crystal structures and provide more accurate predictions of material behavior. Ultimately, overcoming these obstacles is vital for advancing the practical usages of insulator bands in technology.
Future Directions in Insulator Band Studies
The exploration of insulator bands has profound implications in multiple fields such as materials science and electronics. Considering the rapid advancements in technology and materials, future studies focus on not just understanding existing materials but also developing new ones with enhanced properties. Future research aims to uncover innovative approaches to manipulate insulator bands for better performance in various applications.
The viability of integrating cutting-edge techniques into insulator band studies is key. This will help pave the way for novel materials that can achieve unprecedented efficiency and effectiveness.
Integrating AI and Machine Learning
Artificial Intelligence (AI) and machine learning are proving invaluable in accelerating research in solid-state physics. By applying AI algorithms to analyze large datasets, researchers can identify patterns and correlations that may not be immediately apparent through traditional methods.
In the context of insulator bands, machine learning can assist in:
- Predicting properties of new insulating materials, allowing for faster assessment of performance.
- Optimizing synthesis processes to create materials with desired electrical and thermal properties.
- Understanding complex interactions within materials that contribute to the formation of insulator bands.
As a result, we foresee a future where the development cycle for new insulators is significantly shortened, making it feasible to bring enhancements to applications in electronics and energy systems more swiftly.
Sustainable Materials for Future Applications
The importance of sustainability in research cannot be overstated in today’s context. A focus on sustainable materials is essential for future insulation applications. The materials traditionally used have significant environmental impacts when extracted and disposed of. Thus, research is trending toward finding eco-friendly substitutes.
Some critical areas include:
- Biodegradable polymers that can serve similar functions as traditional insulators.
- Recycled materials which reduce waste and leverage existing resources.
- Naturally abundant materials, minimizing the ecological footprint of material sourcing.
By promoting the research and development of sustainable insulator materials, the industry can align itself with global sustainability goals while advancing the performance and efficiency of electronic devices.
"Innovative research in insulator bands could lead to materials that are not only efficient but also eco-friendly, establishing a key aspect of future technological advancements."
Focusing on integrating advanced computational methods along with a commitment to sustainability lays the foundation for transformative improvements in insulator band applications. The direction of future studies in this area is poised to significantly impact both technology and environmental sustainability.
End
The conclusion serves as a pivotal component of any article, synthesizing the key findings and insights that have been discussed. In this article, it encapsulates the significance of insulator bands within solid-state physics and their broader implications within technology and materials science. By revisiting the principal themes, we can appreciate not only the theoretical underpinnings of insulator bands but also their tangible applications across various fields.
Through this exploration, it is clear that insulator bands play a critical role in electronic devices, influencing performance and durability. Understanding the nuances of their formation and properties opens pathways for enhanced functionality. Furthermore, this topic extends beyond the realm of theoretical inquiry; it has profound implications for industries reliant on advanced materials, including consumer electronics and renewable energy systems.
Recapitulation of Key Points
- Role of Insulator Bands: Insulator bands are essential in determining electrical and thermal conductivities across materials, impacting their usefulness in a variety of applications.
- Formation Factors: The conditions leading to the formation of insulator bands include electron configurations, impurities, and defects, which can drastically alter material properties.
- Applications: Insulator bands are crucial in electronic insulation, energy storage, and in the development of advanced composites that are vital for modern technology.
- Future Prospects: Emerging research focuses on innovative materials and methods to tailor insulator bands for specific purposes, which could lead to significant advancements in electronic devices.
Implications for Future Research
The future of insulator band research holds great promise. As technology continues to evolve, there is a need for study that combines theoretical principles with practical applications. Key areas for future investigation include:
- Advanced Materials: Exploration of new insulator materials and their behaviors will enhance comprehension and application across various fields of electronics and manufacturing.
- Machine Learning Integration: Utilizing AI to analyze data can offer predictive insights into band properties and behaviors, leading to developments in material engineering.
- Sustainability: Research focusing on eco-friendly materials is crucial, as the demand for sustainable technologies increases. Identifying natural insulators with minimal environmental impact will be a significant focus.
"The intersection of insulator band properties and advanced technology offers a promising landscape for innovation."
In summary, the comprehensive study of insulator bands is not just academic; it is a critical inquiry that can guide future technological advancements.