Understanding Surface Plasmon: Principles and Applications
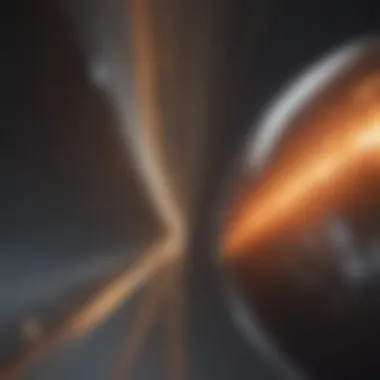
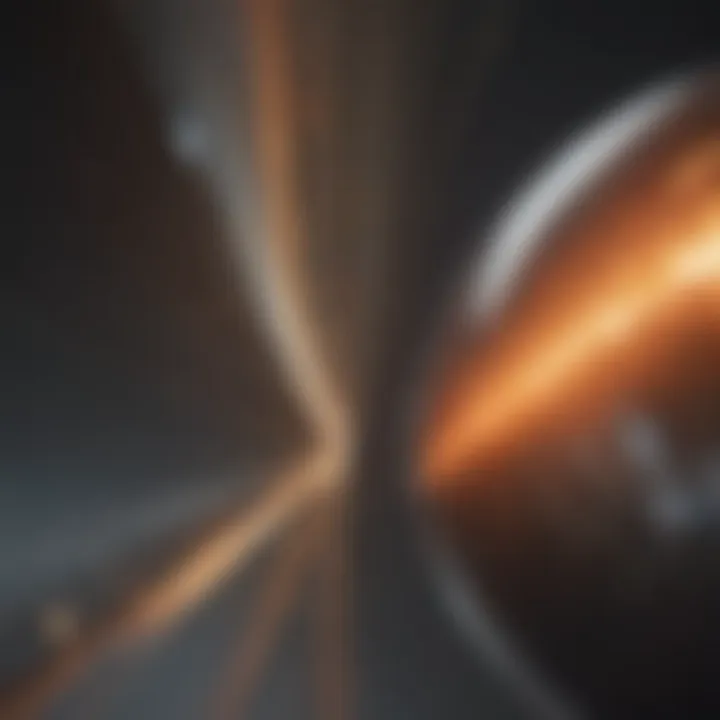
Overview of Research Topic
Brief Background and Context
Surface plasmons represent a pivotal concept in the realm of nanophotonics and materials science. They are coherent oscillations of free electrons at a metal-dielectric interface, coupled with electromagnetic fields. This phenomenon allows light to be confined to a nanometer scale, leading to unique optical properties that have far-reaching implications. Originating from the intricate relationship between light and matter, surface plasmons offer insights into various fields, such as sensing technologies, imaging systems, and photonic devices.
Importance in Current Scientific Landscape
The growing interest in surface plasmon research can be attributed to its diverse applications in science and technology. Its potential for enhancing analytical methods in biosensing is particularly noteworthy. The capacity to detect minute quantities of biological molecules has transformative implications for medical diagnostics. Furthermore, the integration of surface plasmons into photonic circuits could lead to the next generation of high-speed data processing capabilities. Understanding surface plasmons is, therefore, crucial for advancing contemporary scientific inquiry and fostering innovation.
Methodology
Research Design and Approach
To attain a deeper understanding of surface plasmons, a multi-faceted research approach is adopted. The investigation typically encompasses both theoretical frameworks and experimental investigations. Theoretical models often rely on Maxwell's equations to describe the behavior of electromagnetic waves at surfaces. In contrast, experimental methods involve techniques such as surface plasmon resonance (SPR) to observe and manipulate these phenomena.
Data Collection Techniques
Data collection in surface plasmon research employs various techniques, enhancing the reliability of findings. Common methods include:
- Surface Plasmon Resonance (SPR): This technique is widely used for real-time analysis of biomolecular interactions, providing quantitative data on binding affinities and reaction kinetics.
- Scanning Near-field Optical Microscopy (SNOM): This method offers spatial resolution beyond the diffraction limit, allowing researchers to map plasmonic structures at nanometer scales.
- Transmission Electron Microscopy (TEM): TEM is utilized for characterizing nanostructures associated with surface plasmon phenomena.
These techniques not only facilitate the exploration of surface plasmon applications but also reveal fundamental interactions that govern their behavior.
"Understanding the intricacies of surface plasmons is essential for developing novel technologies that harness their unique properties."
By integrating theoretical and experimental components, a thorough grasp of surface plasmons can be achieved, paving the way for remarkable advancements in nanotechnology and related disciplines.
Prelims to Surface Plasmon
The concept of surface plasmon is crucial in the realms of nanophotonics and materials science. Understanding it opens pathways to various applications ranging from biosensing to enhancing energy capture in photovoltaic devices. Surface plasmons occur when light interacts with electrons at the interface between a metal and a dielectric material. This interaction results in collective oscillations of free electrons, yielding unique properties that can be harnessed for innovative technologies.
The benefits of this phenomenon are vast. For one, the ability to manipulate and detect surface plasmons can lead to enhanced sensitivity in sensing applications. Moreover, future advancements in materials and nanotechnology could lead to even more applications not yet considered, making it a subject of great interest and importance.
Research in surface plasmon has implications that extend beyond mere scientific curiosity. By understanding the principles and behaviors associated with surface plasmons, professionals in various fields can explore new solutions to existing problems. The continuous evolution of research in this area signals that surface plasmon has a significant and potentially transformative role in future technological developments.
Definition of Surface Plasmon
Surface plasmons are coherent delocalized electron oscillations that exist at the interface between a conductor and a dielectric. They occur when incident light at a certain frequency excites these oscillations. The waves that result from this excitation are sensitive to the environment, enabling their application in various sensing technologies.
This phenomenon can be understood through the lens of electromagnetism and quantum mechanics. When light hits a metal surface, some photons can transfer their energy to the electrons in the metal. This energy transfer causes the electrons to oscillate collectively, forming a localized wave at the surface of the metal. Surface plasmons can be classified based on the types of metals and dielectrics involved, as well as their propagation properties.
Historical Context
The study of surface plasmon has evolved significantly since its initial discovery. The early theoretical frameworks laid the groundwork for future explorations. The notion of surface plasmons started gaining attention after the advent of modern optics and the recognition of their potential applications. Significant milestones include the development of surface plasmon resonance techniques, which have provided researchers with powerful tools to probe surface phenomena in real time.
The progression from theoretical exploration to practical application was marked by innovations in spectroscopy and microscopy. These advancements have allowed scientists to monitor biological interactions at the molecular level using surface plasmon technologies. As research continues, the historical context of surface plasmon leads to the realization that it is not just a fascinating physical effect, but a cornerstone in the development of cutting-edge scientific methods.
Fundamental Principles of Surface Plasmon
Understanding the fundamental principles of surface plasmon is essential for grasping its implications in nanophotonics and materials science. These principles lay the groundwork for many applications in modern technology. Particularly, the interactions that surface plasmons have with light and matter can lead to significant advancements in various fields.
Interaction of Light with Electrons
Surface plasmons arise from the collective oscillation of electrons at the surface of a conductor. When light illuminates a metallic surface, the electromagnetic field interacts with the surface electrons. This interaction can cause the electrons to oscillate collectively, forming a surface plasmon wave.
The efficiency of this interaction depends on several factors, such as the frequency of the incoming light. Resonance occurs when the frequency of the light matches the natural frequency of the electrons’ oscillation. At this point, the absorption of light is maximized. The resultant plasmons can travel along the surface, enriching the electromagnetic field at the interface, which is instrumental in applications like sensors and imaging systems.
Resonance Condition for Surface Plasmons
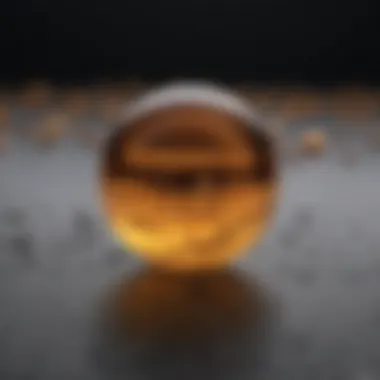
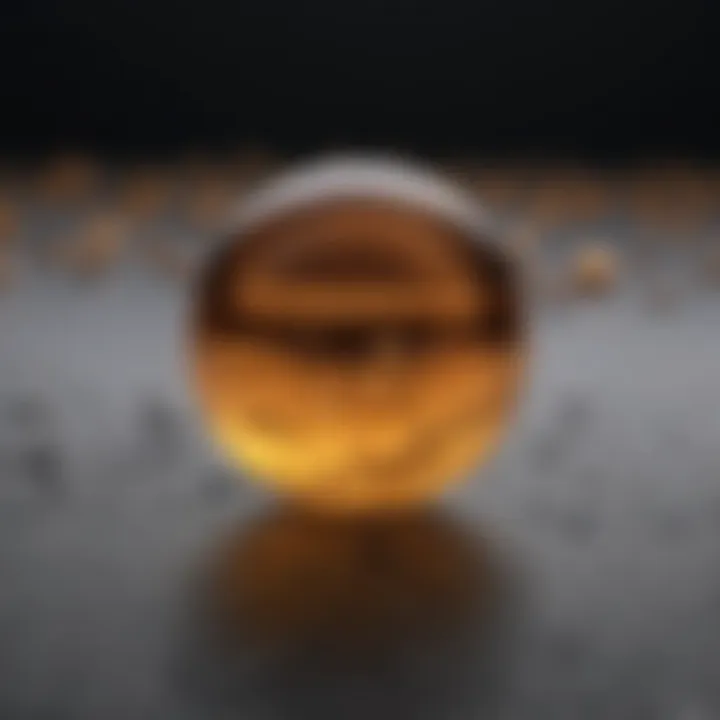
The resonance condition is a key aspect in the behavior of surface plasmons. The phenomenon occurs when the wavelength of light matches the conditions required to excite a surface plasmon. This can be expressed mathematically. In a simplified form, the relation can be articulated as:
k_sp = k_i - k_t
Where:
- k_sp is the wave vector of the surface plasmon
- k_i is the wave vector of the incident light
- k_t is the wave vector of the transmitted light
Once this condition is met, it allows for the maximal energy transfer into the surface plasmon wave, enhancing its effectiveness. Understanding this condition is vital for designing experiments and devices that effectively exploit surface plasmons.
Role of Metal-Dielectric Interfaces
The metal-dielectric interface plays a critical role in supporting surface plasmons. At this boundary, a unique interaction occurs. The electrons from the metal can couple with the electric field of the light within the dielectric medium. This coupling is essential for the propagation of surface plasmons.
Furthermore, the choice of materials influences the properties of the plasmonic waves. Metals such as silver and gold have strong plasmonic responses and are commonly used in applications ranging from biosensing to nanolithography. The dielectric environment, too, can modify the characteristics of the plasmons, such as their wavelength and lifetime.
In summary, comprehending the fundamental principles surrounding surface plasmons allows researchers and professionals to harness their potential effectively. This knowledge serves as the basis for numerous applications across science and technology.
Types of Surface Plasmon Modes
Surface plasmons exhibit diverse modes that are vital for various applications in nanotechnology and material sciences. Understanding these modes allows researchers and professionals to capitalize on the unique properties of plasmons for innovations in sensors, imaging, and photonic devices. The distinction between localized surface plasmons and propagating surface plasmon polaritons is essential because each mode has specific characteristics and potential usages.
Localized Surface Plasmons
Localized surface plasmons occur when light interacts with metallic nanoparticles. These plasmons are confined to the vicinity of the particle’s surface, leading to strong electromagnetic field enhancements. The primary characteristic of localized surface plasmons is their sensitivity to shape, size, and material of the particle.
The applications of localized surface plasmons are numerous and can be found in:
- Biosensing: Utilizing the strong field enhancements for the detection of biomolecules.
- Surface-Enhanced Raman Scattering: Amplifying the Raman signal, enabling detection of low-concentration analytes.
- Imaging Technologies: Enhancing resolution through techniques like near-field scanning microscopy.
Factors to consider when using localized surface plasmons include:
- The choice of metallic material, commonly silver and gold, as they exhibit strong plasmonic behavior.
- The fabrication process for maintaining the desired nanoparticle characteristics.
"Localized surface plasmons bridge the gap between molecular recognition and signal amplification, forming the basis of many modern biosensors and imaging techniques."
Propagating Surface Plasmon Polaritons
In contrast, propagating surface plasmon polaritons (SPPs) arise at the interface of a metal and a dielectric. Unlike localized plasmons, SPPs can travel along this interface, making them suitable for applications that require waveguide properties. The efficiency of wave propagation depends on the dielectric properties and the geometry of the system.
These plasmons have significant implications in:
- Optical Communications: Enabling faster data transfer with reduced signal loss.
- Photonic Circuits: Integrating plasmonic components for compact and efficient devices.
- Metamaterials: Creating materials with tailored optical properties that can manipulate light in novel ways.
Considerations for propagating surface plasmon polaritons include:
- The precise tuning of metal and dielectric interfaces to optimize propagation length and propagation speed.
- The design of devices that increase coupling efficiency between external light sources and the plasmonic medium.
The understanding of both localized and propagating surface plasmons is crucial for leveraging their unique characteristics in advanced technologies. Each mode offers a distinctive set of benefits and limitations, making them suitable for different applications in the realm of nanophotonics.
Experimental Techniques for Surface Plasmon Detection
The investigation of surface plasmons relies heavily on experimental techniques. These methods provide not just the means to observe surface plasmon phenomena but also to quantify their properties. Understanding the advantages and limitations of these techniques is crucial for researchers aiming to delve deeper into nanophotonics and materials science. Each method has certain characteristics that suit specific applications, thus expanding the toolkit available to scientists.
Surface Plasmon Resonance (SPR) Spectroscopy
Surface Plasmon Resonance spectroscopy is a widely recognized method for the detection and analysis of biological interactions. SPR exploits the changes in the refractive index near a metal surface that occur when surface plasmons are excited. The primary benefit is its label-free nature, allowing real-time monitoring without the need for fluorescent markers. This makes SPR especially useful in biomolecular studies, where it can identify binding events at very low concentrations.
The setup typically includes a sensor chip coated with a thin layer of metal, often gold. When light hits this layer at a certain angle, it can excite plasmons, leading to a sharp dip in reflectivity at a specific wavelength. Researchers then analyze these changes to provide quantitative data on molecular interactions and kinetics. One of the concerns with SPR is that it is sensitive to environmental fluctuations such as temperature changes, which can affect the accuracy of measurements. However, advances in technology are continually addressing these issues, resulting in more robust systems.
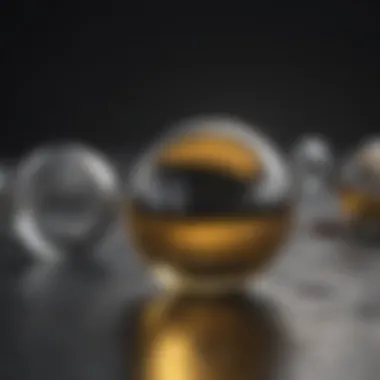
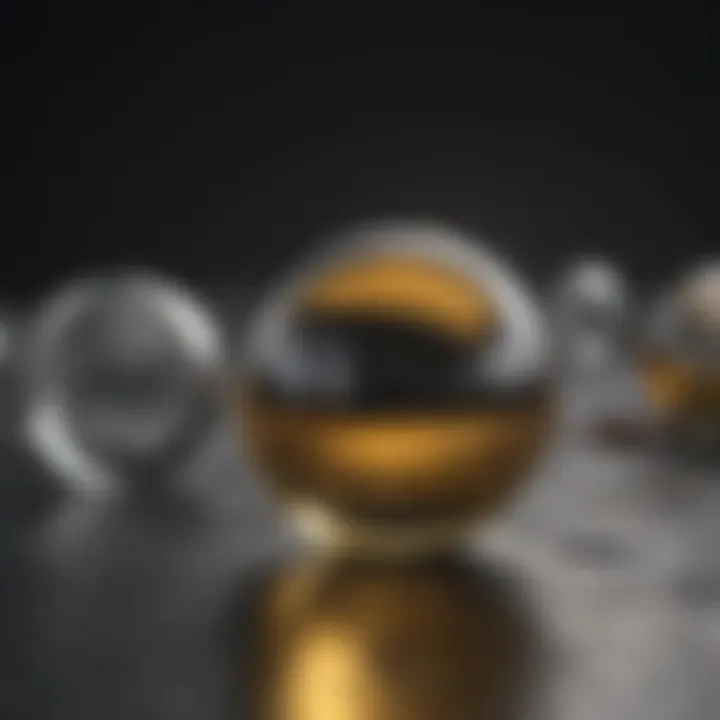
Near-Field Scanning Optical Microscopy (NSOM)
Near-Field Scanning Optical Microscopy offers a distinct approach to visualize surface plasmons at a nanoscale level. Unlike traditional optical microscopy, which is limited by diffraction limits, NSOM can achieve resolutions much finer than the wavelength of light. This capability is particularly advantageous for examining the nanoscale structures that contribute to plasmonic effects.
In NSOM, a sharp tip is scanned over the surface of a material. This tip, when close enough to the sample, can interact with the surface plasmons, allowing mapping of their spatial distribution. The benefit of this method lies in its ability to provide detailed images and information about local plasmonic behavior. Nevertheless, it is essential to note that the method can be affected by tip-sample interactions, which may introduce artifacts in measurements. Continued research seeks to enhance NSOM's reliability and effectiveness in revealing surface plasmon structures.
Electron Energy Loss Spectroscopy (EELS)
Electron Energy Loss Spectroscopy is another potent technique for probing the properties of surface plasmons. EELS is conducted in transmission electron microscopy setups and involves sending a beam of electrons through a thin sample. As these electrons traverse, they may lose energy to excite surface plasmons in the material.
This energy loss is correlated to the excitation of surface plasmon modes, providing valuable insights into the optical properties of materials at a microscopic level. The primary advantage of EELS is its ability to provide elemental and chemical information along with spatial resolution typically in the range of nanometers. However, the method requires ultrahigh vacuum conditions and thin specimens, which can be limiting factors. Its sensitivity makes EELS a powerful tool for researchers studying new materials and their plasmonic properties.
The choice of experimental technique substantially influences the findings in surface plasmon research, shaping everything from experimental designs to interpretations.
Applications of Surface Plasmon
The topic of applications of surface plasmon is pivotal in understanding how this phenomenon enhances various technological fields. The ability to manipulate light at the nanoscale through surface plasmons leads to significant advancements in biosensing technologies, photovoltaic devices, drug delivery systems, and surface-enhanced Raman scattering. These applications capitalize on the unique properties of surface plasmons, such as their sensitivity to environmental changes and their capacity to amplify signals. Understanding these applications can provide insights into the potential advancements stemming from surface plasmon research, pushing scientific and technological boundaries further.
Biosensing Technologies
Biosensing technologies have rapidly evolved with the integration of surface plasmon resonance (SPR). This technology takes advantage of the changes in refractive index that occur when biomolecules bind to a sensor's surface. The detection of these changes is highly sensitive and specific, allowing for real-time monitoring of biological interactions without the need for extensive sample preparation.
Key points include:
- High Sensitivity: SPR can detect low concentrations of biomolecules, making it ideal for medical diagnostics and environmental monitoring.
- Real-Time Analysis: The ability to monitor binding events as they happen allows researchers to gather dynamic information about molecular interactions.
- Versatile Applications: From glucose sensors for diabetics to diagnostic tools in oncology, the potential applications are vast and growing.
Enhancement of Photovoltaic Devices
The integration of surface plasmons into photovoltaic devices has presented new opportunities for improving energy conversion efficiencies. By incorporating metallic nanostructures, these devices can capture light more effectively, enhancing the absorption spectrum. This phenomenon can result in:
- Increased Light Harvesting: Surface plasmons can concentrate light into subwavelength volumes, allowing for the absorption of otherwise missed wavelengths.
- Cost Efficiency: More efficient use of materials can lead to lower production costs for solar panels.
- Broader Wavelength Utilization: By tuning plasmonic structures, devices can be optimized for various parts of the solar spectrum.
Drug Delivery Systems
The use of surface plasmons in drug delivery represents a thrilling intersection of nanotechnology and medicine. Plasmonic nanoparticles can be designed to release drugs in a controlled manner, responding to external stimuli such as light or temperature. Notable aspects include:
- Targeted Delivery: Applications can be focused on specific cells or tissues, improving efficacy and minimizing side effects.
- Theranostic Applications: By combining therapy and diagnostics, surface plasmons can help monitor the delivery and effectiveness of drugs in real-time.
- Customizable Nanocarriers: The surface properties of nanoparticles can be tailored to enhance attachment and controlled release of therapeutics.
Surface-Enhanced Raman Scattering (SERS)
Surface-enhanced Raman scattering (SERS) is a powerful technique enabled by surface plasmons, utilizing the enhanced electromagnetic fields at metal surfaces to amplify Raman signals from molecules. This has significant implications for chemical analysis and sensing, with benefits including:
- Extremely High Sensitivity: SERS can detect single molecules, making it useful in areas like environmental monitoring and security.
- Universal Applicability: It can be employed in various fields such as biology, chemistry, and materials science, facilitating thorough investigations of complex mixtures.
- Non-Destructive Testing: The technique allows for analysis without damaging samples, which is essential in many research and industrial applications.
In summary, the applications of surface plasmon phenomena are vast and varied, presenting new opportunities in numerous field. Their ability to enhance detection methods, improve energy efficiency, and enable sophisticated delivery systems reflects the ongoing evolution in technology driven by the principles of surface plasmon.
In closing, exploring and understanding each of these applications further emphasizes the importance of surface plasmons within modern science and technology.
Theoretical Models of Surface Plasmon Behavior
Understanding the theoretical models of surface plasmon behavior is crucial to comprehending how these phenomena operate at a fundamental level. The models provide a framework for predicting how surface plasmons will interact under various conditions. They help researchers to simulate and understand complex behaviors in nanostructures, thereby facilitating advancements in applications such as sensors, photovoltaics, and photonic devices. Without such models, interpreting experimental results would be challenging, as they serve as benchmarks against which real-world observations can be compared.
Drude Model
The Drude model serves as a cornerstone in the study of surface plasmons. It was developed in the early 20th century to describe the behavior of electrons in metals. In this model, electrons are treated as a gas of charged particles that can collide with impurities and lattice vibrations. This simple yet effective approach allows researchers to derive important properties of metals that facilitate surface plasmon excitation.
One of the key aspects of the Drude model is its focus on the dielectric response of metal. According to the model, the electrons respond to electromagnetic fields, resulting in a complex dielectric constant. This is integral to understanding the resonance conditions for surface plasmons. The model also lays the foundation for calculating plasmon dispersion relations, which relate frequency and wave vector for plasmon excitations.
Quantum Mechanical Approaches
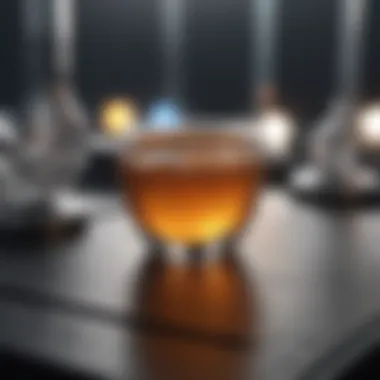
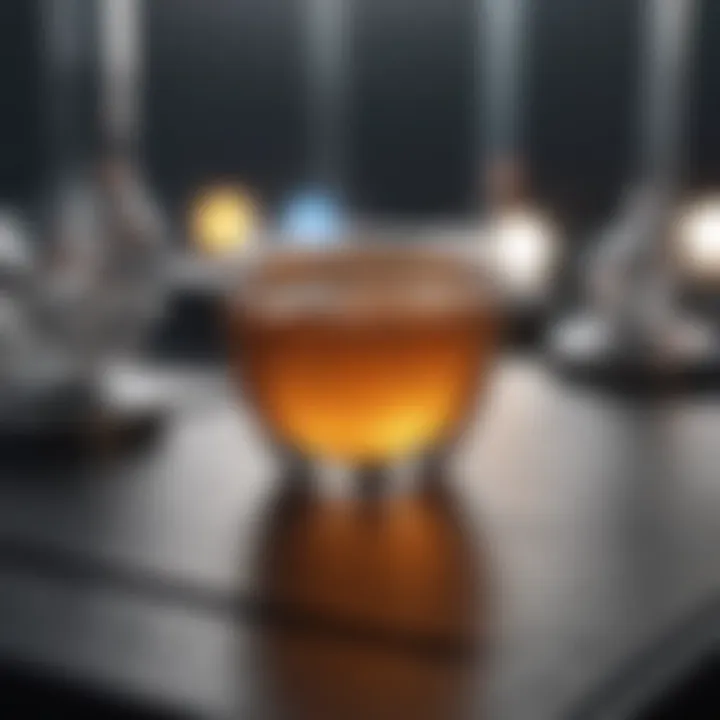
In contrast to the Drude model, quantum mechanical approaches provide a more nuanced perspective on surface plasmon behavior. These models account for quantum effects that become significant at smaller scales, especially in nanostructured materials. Quantum mechanics considers the wave-particle duality of electrons, allowing for a better understanding of phenomena such as electron tunneling and localization.
Using quantum mechanical frameworks, one can derive the energy levels and modes associated with surface plasmons under various configurations. More advanced models incorporate aspects such as electron-electron interactions, which influence the collective behavior of electrons on the metal surface. This results in a more accurate depiction of plasmon dynamics and their interaction with light.
Overall, both the Drude model and quantum mechanical approaches are essential in the analysis and prediction of surface plasmon behavior. They enable researchers to explore and design advanced materials and devices that utilize the unique properties of surface plasmons. Understanding these theoretical constructs is vital for future innovations in nanoparticles, and nanophotonics.
Limitations and Challenges in Surface Plasmon Research
Understanding the limitations and challenges in surface plasmon research is crucial for advancing the science and technology in this field. Despite its many advantages, research on surface plasmons encounters obstacles that can hinder progress and practical applications. Recognizing these constraints allows researchers to strategize potential solutions and innovative approaches. This section delves into two major aspects: material constraints and environmental sensitivity, both of which have significant implications for the future of surface plasmon technologies.
Material Constraints
Material selection plays a vital role in the behavior and efficiency of surface plasmons. Surface plasmons are typically supported by noble metals such as gold and silver. While these materials have excellent plasmonic properties, there are several limitations associated with them:
- Cost and Accessibility: Noble metals are expensive and can limit the scalability of plasmonic devices in practical applications. This can be a barrier for large-scale production and deployment.
- Material Stability: Gold and silver can oxidize or degrade under certain environmental conditions. This degradation can lead to a loss of plasmonic efficiency over time, making long-term studies more complex.
- Limited Wavelength Range: The plasmonic response is often confined to certain wavelengths, reducing the versatility of these materials in various applications. Researchers are exploring alternatives such as titanium dioxide and other conductive materials to improve versatility but these are not without their own challenges.
The shift to newer materials demands in-depth analysis to understand their plasmonic capabilities and how they compare to traditional noble metals.
Environmental Sensitivity
Surface plasmons can be influenced by their surrounding environment. This sensitivity presents challenges in experimental conditions and applications. Key considerations include:
- Refractive Index Variations: Changes in the refractive index of nearby materials can affect the resonance conditions for surface plasmons. This requires precise control in experimental setups and can lead to variability in results.
- Temperature Fluctuations: Temperature changes can alter the properties of metal-dielectric interfaces, impacting surface plasmon behavior. Therefore, maintaining a controlled environment is critical for precise measurements.
- Contaminants and Interference: The presence of contaminants, such as dust or other particles, can affect the interactions between light and the surface plasmons. This can lead to unreliable data and complications in sensor applications.
Understanding these environmental factors is essential for enhancing the reliability of plasmonic devices, especially in fields like biosensing where external conditions can fluctuate significantly.
In summary, while surface plasmon research holds great promise, it is essential to address the limitations related to material constraints and environmental sensitivity. These challenges require innovative solutions and interdisciplinary approaches to fully harness the capabilities of surface plasmons in practical applications.
Future Directions in Surface Plasmon Research
The study of surface plasmon phenomena is not only a reflection of the past and present scientific achievements but also a door to future technological advancements. This domain, particularly in nanophotonics, is ripe with potential. Understanding the future directions of surface plasmon research illuminates the areas where innovation can occur, leading to significant implications for various fields. The integration with nanotechnology and the exploration of new materials are two critical elements that will shape future research.
Integration with Nanotechnology
Nanotechnology plays a vital role in advancing surface plasmon research. The unique properties of materials at the nanoscale allow for enhanced interactions between light and matter. This can improve the performance of devices, such as sensors and solar cells. Recent developments show that by integrating plasmonic materials with nanostructures, researchers can achieve higher sensitivity and efficiency.
Key areas of exploration include:
- Nano-Optical Devices: Utilizing surface plasmons in photonic circuits can lead to the development of compact and efficient devices.
- Biosensors: Enhanced sensitivity from plasmonic constructs allows for more accurate detection of biological agents.
- Energy Applications: The coupling of surface plasmons with nanostructured materials enhances light absorption in photovoltaic devices, improving their efficiency.
Ongoing research also focuses on designing plasmonic nanostructures with precise control over their geometry, which can tailor their optical properties for specific applications.
Exploration of New Materials
Exploring new materials for surface plasmon research is equally crucial. Traditional metals like gold and silver provide good plasmonic properties, but they also have limitations, such as high costs and susceptibility to oxidation. Researchers are pursuing a variety of alternative materials that show promise in enhancing plasmonic effects. These materials include:
- Graphene and Other Two-Dimensional Materials: Exhibiting unique optical properties, they can support surface plasmons at optical frequencies.
- Metalloids and Alloys: New metal combinations can lead to improved performance while reducing costs and enhancing durability.
- Dielectric Materials: Incorporating dielectrics into plasmonic systems has shown improvements in light management and influence of electromagnetic phenomena.
"The ongoing developments in surface plasmon technologies could redefine your interaction with light and materials, paving the way for significant breakthroughs in science and engineering."
By focusing on these areas, researchers can contribute to developing next-generation technologies with superior capabilities.
Culmination
The conclusion section serves as a vital closing to the extensive examination of surface plasmon phenomena. It encapsulates the essential insights gleaned from the research, highlighting how surface plasmons play a pivotal role in modern nanophotonics and materials science. This concept has profound implications not only for scientific understanding but also for practical applications that affect various fields including biosensing, energy, and advanced imaging techniques. Each aspect of the topic we discussed contributes to the broader narrative of innovation and technological advancement.
Summary of Key Findings
A. Surface plasmon resonances significantly enhance the interaction of light with matter, leading to improved sensitivity in detection methods.
B. Surface plasmon modes can vary, including localized surface plasmons and propagating surface plasmon polaritons, each with distinct characteristics and applications.
C. Experimental techniques like Surface Plasmon Resonance (SPR) and Near-Field Scanning Optical Microscopy (NSOM) are crucial in detecting and studying these phenomena.
D. Applications in biosensing and photovoltaics demonstrate the versatility of surface plasmons in enhancing technology that meets societal needs.
E. Understanding material limitations and environmental sensitivity is key to addressing challenges in surface plasmon research.
Implications for Future Research
Future research in surface plasmon phenomena holds exciting potential. New materials and technologies can lead to enhanced performance in fields such as sensing and energy conversion.
- Integration with nanotechnology encourages the development of novel devices that utilize plasmonic properties for improved functionality.
- Exploration of new materials, like graphenes or transition metal dichalcogenides, may reveal unique plasmonic behaviors that could revolutionize applications.
- The influence of environmental factors on plasmon behavior indicates a need for further studies on how surface plasmon interactions can be optimized in real-world conditions.
As surface plasmon research continues to evolve, the exploration of these dimensions will likely uncover transformative technologies with significant impact on science and industry.