Spectrum Resolution: An In-Depth Exploration
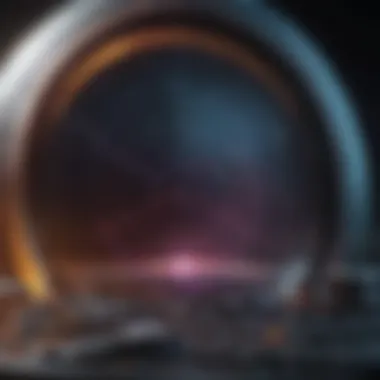
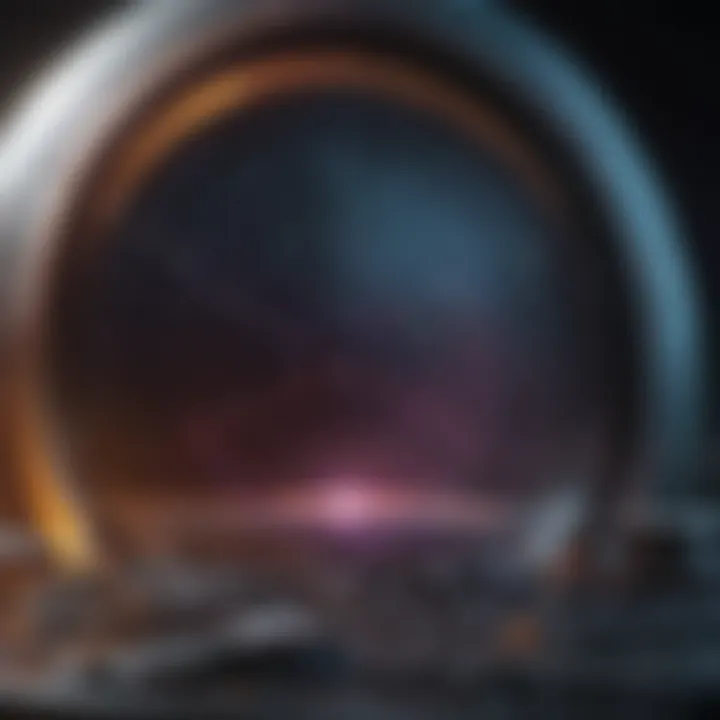
Intro
In the expansive world of scientific inquiry, spectrum resolution stands out as a foundational concept that influences diverse fields such as physics, chemistry, and biology. Understanding spectrum resolution is critical, not just for academic exploration but also in practical applications. With advancements in technology, its relevance in data interpretation has amplified, particularly in analytical techniques that rely on precise measurements to reveal underlying phenomena.
This article serves as a detailed guide through the nuances of spectrum resolution, shedding light on its principles, significance, and methodologies employed to enhance it. Researchers, educators, and science enthusiasts will find a comprehensive exploration that aims to deepen their understanding and appreciation of this essential topic.
Overview of Research Topic
Brief Background and Context
Spectrum resolution refers to the ability to distinguish between different wavelengths or frequencies in a spectrum. It is a vital characteristic of various analytical methods used in laboratories. The historical development of spectrum analysis began in the 17th century and has evolved with technological innovations, such as spectroscopy techniques, that allow for high-quality data acquisition.
Importance in Current Scientific Landscape
With ongoing advancements in science and technology, the importance of spectrum resolution has escalated. High-resolution spectral data enables precise measurements which lead to better interpretation of complex chemical mixtures, identification of molecular structures, and dynamic studies in biological systems. As researchers push the boundaries of knowledge, the demand for more accurate spectral data continues to grow.
"The clarity of the spectrum directly influences our capacity to make informed decisions based on its data."
Methodology
Research Design and Approach
To effectively study spectrum resolution, a two-fold approach can be adopted: theoretical frameworks and practical applications. Theoretical frameworks provide the foundation for understanding the principles behind resolution, while practical applications exemplify how these principles manifest in real-world scenarios. This combination creates a holistic understanding of the topic.
Data Collection Techniques
Data collection is executed through various methods, which can include:
- Spectroscopy: Using light to analyze materials based on their interaction with electromagnetic radiation. Popular spectral techniques include UV-Vis spectroscopy, NMR spectroscopy, and mass spectrometry.
- Sensor Technology: Employing sensors that work on principles of spectrum resolution, such as CCDs or photomultiplier tubes.
- Computational Tools: Analyzing spectral data through software that applies algorithms designed to enhance resolution and interpret the results accurately.
The data gathered through these techniques aids in refining the understanding of spectrum resolution and its implications in scientific research.
This article will proceed to elaborate further on the principles of spectrum resolution. Subsequent sections will explore specific applications, the challenges researchers face, and the future of spectrum resolution in science.
Intro to Spectrum Resolution
Understanding spectrum resolution is a cornerstone of many scientific disciplines, including physics, chemistry, and biology. It provides insights into the quality of data collected during experiments and plays a crucial role in the analysis and interpretation of that data. This introduction serves to illuminate the foundational importance of spectrum resolution and how it influences various research outcomes.
Definitely, spectrum resolution emphasizes the ability to differentiate between closely spaced spectral features. Higher resolution allows scientists to detect subtle differences and nuances that could otherwise go unnoticed. This is imperative in fields like analytical chemistry, where precise measurements can lead to significant advancements. For instance, in mass spectrometry, precise resolution can be the difference between identifying a compound correctly or misinterpreting its presence.
In addition, this topic leads to exciting possibilities when researchers leverage spectrum resolution for applications like environmental monitoring and medical diagnostics. A thorough understanding of this concept fosters innovation, enhancing techniques that can yield groundbreaking discoveries.
Defining Spectrum Resolution
Spectrum resolution refers to the clarity with which spectral data can be interpreted. A high resolution means that two closely spaced spectral lines can be distinguished easily from each other. This attribute is quantified by the resolving power of the instrument used for measurement, which is often defined as the ratio of the wavelength to the width of the peak produced by a spectral feature.
It’s important to note that spectrum resolution isn't just a numerical value; it encapsulates a multidimensional understanding of how instruments can be optimized to yield the best data possible. For instance, in spectroscopy, resolution is influenced by a variety of factors, including the physical properties of the spectrometer and the nature of the sample being analyzed. Therefore, resolving spectral features is pivotal in ensuring accurate results, which can guide further research and practical applications.
Historical Context
The history of spectrum resolution is rich, tracing back to the early days of spectroscopy in the 19th century. Initially, the rudimentary instruments limited the capabilities of scientists to analyze light spectra. However, as technology evolved, so did the mechanisms for achieving higher resolution.
Developments in optics and the introduction of new materials have continually pushed the envelope in this area. For example, the invention of diffraction gratings marked a significant turning point. These devices allowed for better separation of spectral lines and fundamentally improved the resolution of spectrometers.
The 20th century saw further advancements, especially with the introduction of digital data analysis methods that provided more accurate and nuanced interpretations of spectral data. As a result, the importance of high spectrum resolution in various fields grew exponentially, paving the way for innovative research methodologies.
In summary, spectrum resolution is more than just a technical detail; it is a vital concept interwoven into the fabric of scientific inquiry, influencing both historical and modern investigative techniques. Understanding its evolution enhances appreciation for the complexities involved in scientific research.
The Physics of Spectrum Resolution
The physics underlying spectrum resolution is critical in understanding how various analytical techniques function. It governs the ability to distinguish between closely spaced spectral lines or signals. This characteristic is vital in fields like spectroscopy, where the separation of light into its constituent parts provides insights into the composition and properties of materials.
The understanding of this concept can lead to enhanced information retrieval from complex data. As we delve deeper into the two primary elements that shape spectrum resolution, namely wave-particle duality and Fourier transform techniques, we also recognize how these principles find applications across different scientific domains.
Wave-Particle Duality
Wave-particle duality is a fundamental principle in quantum mechanics, stating that every particle or quantum entity can be described as either a particle or a wave. This duality plays a significant role in spectrum resolution because it influences how light and matter interact. For instance, when light behaves like a wave, it can produce interference patterns, which can be analyzed for high-resolution measurements.
This behavior is especially important in advanced spectroscopic methods, where precise resolution can be achieved. The wave nature of light enables the differentiation of closely spaced wavelengths through diffraction and interference. Understanding this duality fosters more sophisticated approaches in designing analytical instruments, ultimately improving their spectrum resolution capabilities.
Fourier Transform Techniques
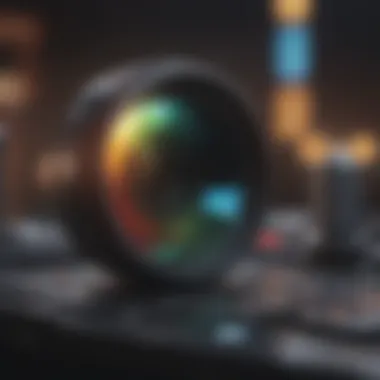
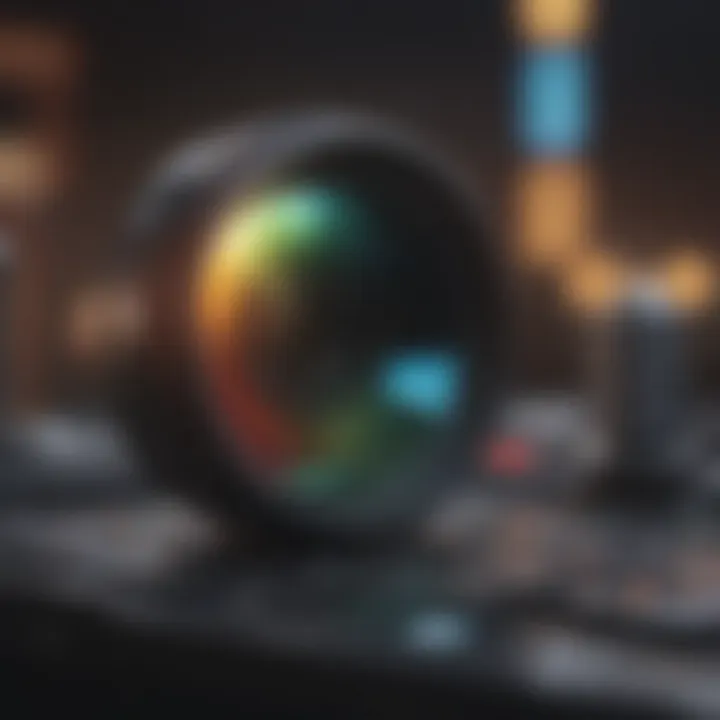
Fourier Transform techniques are instrumental in the analysis of signals. Using these techniques, complex signals can be decomposed into their constituent frequencies. Fourier transforms enable the conversion of time-domain data into frequency-domain data, which enhances the understanding of the spectral characteristics associated with that data. In spectrum resolution, this conversion is vital.
The application of Fourier transforms can be seen in various spectroscopic methods, including nuclear magnetic resonance and mass spectrometry. By manipulating how data is represented, researchers can optimize resolution and extract meaningful information from datasets.
"Fourier Transform techniques are essential for achieving high-resolution analysis, allowing researchers to interpret complex spectral data effectively."
The benefits of employing Fourier Transform techniques are significant:
- Enhanced Resolution: Higher precision in identifying spectral lines allows for better interpretation of results.
- Noise Reduction: Improved algorithms can remove noise in the data, revealing clearer spectra.
- Comprehensive Analysis: It supports the analysis of complex mixtures by separating overlapping signals.
Utilizing these methods effectively can bridge the gap between theoretical understanding and practical application. Therefore, grasping the physics behind these techniques is crucial for advancing research and technology in spectrum resolution.
Importance in Scientific Research
Spectrum resolution plays a vital role in scientific research. It directly influences the clarity and detail of data obtained from various analytical techniques. High spectrum resolution allows researchers to discern finer details in the spectral data, which is crucial for accurate analysis. Consequently, it aids in making precise measurements and conclusions across numerous fields, from chemistry to biology.
The ability to quantify substances accurately hinges on the resolution of the spectrum used. Higher resolution can lead to a better differentiation between closely spaced peaks in a spectrum. In quantitative analysis, this means that substances present in low concentrations can be detected and measured reliably. The implications of accurate quantitative data are immense. For example, in drug development, precise quantification of active ingredients can help ensure efficacy and safety.
In addition to quantitative benefits, spectrum resolution also enhances qualitative analysis. Researchers can identify unknown compounds more effectively when the resolution is high. Each compound in a mixture produces a unique spectral fingerprint, and better resolution allows for clearer differentiation between these fingerprints. This clarity can help in applications ranging from environmental monitoring to forensic science, where specific substance identification is essential.
"High spectrum resolution is an indispensable tool for unveiling subtleties that would otherwise go unnoticed in complex samples."
Moreover, the integration of advanced methods to enhance resolution often intersects with technological advancements. For instance, developing new spectroscopic techniques or improving laser technology can lead to breakthroughs in how we understand chemical properties or biological systems. As science continues to evolve, the importance of spectrum resolution will only increase, fostering new insights into the natural world and informing diverse applications. The interplay of quantitative and qualitative analysis within the sphere of spectrum resolution underlies its significance in a broad range of scientific inquiries.
Quantitative Analysis
Quantitative analysis involves measuring the concentration of components in a sample. In this field, clarity is key. Improved spectrum resolution enables scientists to detect and analyze components present in minimal amounts. This is especially critical in industries like pharmaceuticals, where precise dosing of compounds can significantly affect drug effectiveness.
A good example is the use of High-Performance Liquid Chromatography (HPLC), an analytical technique that relies heavily on spectrum resolution. When analyzing drug compounds, a higher resolution can reveal subtle differences in the spectral data, ensuring that impurities and active ingredients are accurately identified. The better the resolution, the more reliable the data, leading to enhanced reproducibility of results.
Qualitative Analysis
In contrast, qualitative analysis focuses on identifying components in a mixture. Spectrum resolution allows researchers to recognize patterns and unique identifiers in spectral data. Each chemical compound exhibited in a spectrum has distinct characteristics and varying levels of complexity. With high resolution, even subtle differences become apparent, enabling scientists to differentiate between similar substances.
For example, in biological research, mass spectrometry is used extensively for analyzing proteins. Accurately identifying protein sequences is vital for understanding biological mechanisms. Higher spectrum resolution allows researchers to pinpoint variations in amino acid sequences, facilitating discoveries related to diseases or biological pathways.
Overall, improving spectrum resolution is critical in scientific research, especially in areas requiring precise quantitative and qualitative analysis. This capability not only enhances the accuracy of results but also propels onward the development of methodologies in diverse research fields.
Methods for Enhancing Spectrum Resolution
Enhancing spectrum resolution is critical for extracting detailed information from complex datasets. It enables researchers to distinguish closely related spectral features that may hold vital clues in their analysis. The methods utilized to improve resolution can significantly impact the accuracy of interpretation in various scientific fields.
This section explores both spectroscopic techniques and computational approaches, each providing different advantages and considerations. Understanding these techniques aids researchers in selecting the best methods for their specific needs, ensuring precision in their results.
Spectroscopic Techniques
Nuclear Magnetic Resonance
Nuclear Magnetic Resonance (NMR) is a powerful spectroscopic technique used primarily for structural determination in chemistry and biology. The key characteristic of NMR is its ability to provide detailed information about the local environment of nuclei in molecules. This unique feature allows researchers to deduce structural and dynamical information with high precision.
One of the main advantages of NMR is its non-destructive nature. It typically requires only small amounts of sample material, making it a valuable tool in studying complex biological systems. However, the technique is often time-consuming and requires significant expertise to interpret the resulting spectra. Still, NMR remains a popular choice among scientists for its unique capabilities and depth of information it provides.
Mass Spectrometry
Mass Spectrometry is another crucial technique for enhancing spectrum resolution, especially in the analysis of molecular compositions. It excels at detecting and characterizing a vast range of biomolecules. The main strength of Mass Spectrometry is its ability to separate ions based on their mass-to-charge ratios. By employing various ionization techniques, Mass Spectrometry can analyze complex samples quickly and with great sensitivity.
A distinctive feature of Mass Spectrometry is its high mass resolution, which allows the differentiation of molecules that differ by even a single atomic mass unit. One downside is the potential complexity of the sample preparation process, which can affect the clarity of the results. Nevertheless, the technique's broad applicability across various disciplines solidifies its position as an essential method.
Computational Approaches
Algorithms for Data Processing
Algorithms for Data Processing are vital in modern research that deals with large datasets. These algorithms optimize the interpretation of spectral data, significantly enhancing resolution through various computational techniques. The central role of these algorithms is to manage noise reduction and improve signal clarity, thereby refining the resolution.
Many researchers prefer to apply sophisticated algorithms because they can automate data analysis, allowing for increased efficiency and reproducibility. However, the choice of algorithm can depend on the specific needs of the research, such as the type of spectral data and the desired outcome. One challenge is the need for extensive validation, to ensure the reliability of the processed data.
Machine Learning Applications
Machine Learning Applications are becoming increasingly important for enhancing spectrum resolution. These applications utilize advanced statistical techniques to analyze complex datasets, making them a favored choice for many researchers. Machine learning can identify patterns in spectral data that may not be visible through traditional analysis techniques.
The primary advantage of employing machine learning is its ability to adapt and learn from large datasets, improving accuracy over time. Additionally, it can handle datasets with missing or varied data values more effectively than conventional methods. On the other hand, the dependence on substantial amounts of data for training can be a limitation. Despite this, the versatility of machine learning in analyzing spectra has significant implications for future research and development.
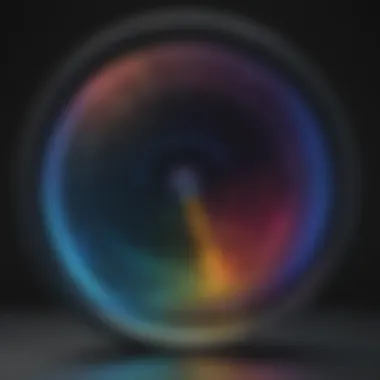
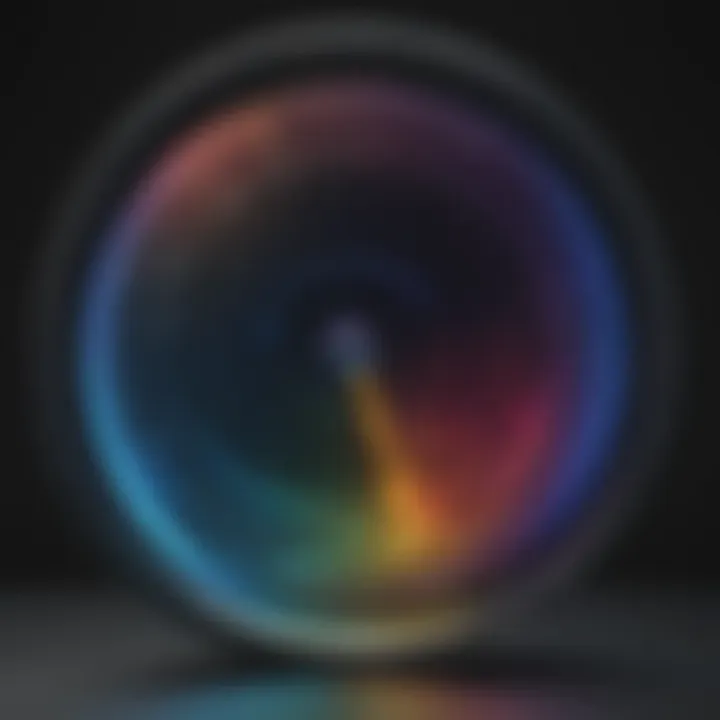
Impact of Resolution on Data Interpretation
Spectrum resolution plays a pivotal role in determining how effectively scientists can interpret data. Essentially, resolution defines the ability to resolve distinct signals within a spectrum. As a result, it directly influences the quality and accuracy of the data collected across various scientific disciplines.
In many cases, higher resolution allows researchers to discern subtle differences between closely spaced spectral lines. This is crucial in fields such as chemistry and biology, where tiny variations in molecular structures may lead to vastly different properties or behaviors. The clarity of the spectrum consequently impacts the conclusions drawn from the data, underscoring the significance of resolution in analytical techniques.
Resolution vs. Sensitivity
While resolution and sensitivity are often mentioned together, they are not the same thing. Resolution refers to the ability to separate two closely spaced signals or peaks in a spectral analysis. Sensitivity, on the other hand, is the ability to detect small quantities of substances or low concentrations in a sample.
A common misconception is that increasing resolution automatically enhances sensitivity. However, this is not universally true. For example, a high-resolution instrument might capture detailed data but may still fail to detect low-abundance species if the overall sensitivity is low. Therefore, it is essential to balance these two aspects for reliable analytical results.
Common Misinterpretations
Misinterpretations concerning spectrum resolution can lead to erroneous conclusions. One prevalent misunderstanding is to regard resolution as an isolated feature of an analytical technique. In reality, multiple factors, including, but not limited to, the instrument's design, the sample's nature, and environmental conditions, influence resolution.
Another frequent misinterpretation occurs in the context of data processing. Some researchers may assume that post-data acquisition processing can completely compensate for low resolution. While advanced algorithms can improve data quality, they cannot substitute for the inherent limitations of the measurement itself.
"Resolution is not solely a characteristic of an instrument; it is an intrinsic feature of the analysis process that necessitates careful consideration."
Ultimately, understanding these distinctions and avoiding common pitfalls is crucial for researchers aiming to interpret their data accurately. By navigating the complexities of resolution versus sensitivity and clarifying common misconceptions, scientists can enhance their analytical capabilities and achieve more reliable results.
Advanced Technologies and Spectrum Resolution
The integration of advanced technologies into spectrum resolution plays a vital role in enhancing the analytical capabilities of various scientific disciplines. Innovations such as thin-film coatings and laser interferometry represent significant developments that contribute to the precision and accuracy of spectral measurements. These advancements not only optimize performance but also enable the exploration of new frontiers in research and applications.
Thin-Film Coatings
Thin-film coatings are one of the most profound advancements in the technology surrounding spectrum resolution. These layers, which consist of a variety of materials applied in controlled thickness, can modify the optical properties of surfaces. The significant benefits provided by thin-film coatings include:
- Improved Sensitivity: Coatings can significantly heighten the sensitivity of instruments. This is essential in applications like spectroscopy, where detecting subtle variations in spectrum is crucial.
- Reduction of Noise: By minimizing unwanted reflections and enhancing desired signals, these coatings ensure that the data obtained is clearer and more interpretable.
- Versatility in Applications: Thin films can be engineered for specific wavelengths, thus making them adaptable across different scientific fields, from material science to biological research.
In practical terms, these coatings allow for the fine-tuning of detectors and lenses within spectrometers, resulting in results that provide deeper insights than previously possible. The continual evolution of materials and techniques in thin-film technology suggests that the frontier of spectrum resolution will expand even further.
Laser Interferometry
Laser interferometry represents another significant technological advancement in spectrum resolution. This technique employs the principles of superposition and interference to extract precise measurements of wavelengths and frequencies. The characteristics of laser interferometry that bolster spectrum resolution include:
- High Precision: The coherence of laser light ensures highly accurate measurements, making it instrumental in both fundamental research and practical applications such as metrology.
- Real-Time Analysis: With the ability to measure changes instantaneously, laser interferometry is invaluable in dynamic environments, from industrial processes to analyzing biological reactions.
- Application Across Disciplines: The technology finds utilization in various fields, including engineering, physics, and astronomy, enhancing the resolution of data obtained from numerous experiments and observations.
"Laser interferometry offers a level of precision that traditional methods struggle to achieve, allowing researchers to push the boundaries of what is possible in spectrum analysis."
In summary, the convergence of these advanced technologies—thin-film coatings and laser interferometry—into spectrum resolution emphasizes a direction that seeks enhanced measurement capabilities. As we move forward, these technologies not only assist individual scientific domains but also pave the way for interdisciplinary advancements, enriching our overall understanding of the physical world.
Applications Across Scientific Disciplines
Spectrum resolution is crucial across various scientific fields, influencing how researchers analyze and interpret data. Its applications span biology, environmental science, and more. Understanding the significance of spectrum resolution in these disciplines offers insights into the methodologies and technologies that drive modern scientific inquiry.
Biological Research
Genome Analysis
Genome analysis exemplifies how spectrum resolution plays a vital role in biological research. It is central to understanding the genetic make-up of organisms. The key characteristic of genome analysis is its ability to provide a detailed view of DNA sequences, which is essential for genomic studies. This feature allows scientists to map genes accurately, identify mutations, and understand complex genetic interactions, making it a popular choice in contemporary research.
One unique aspect of genome analysis is its use of high-throughput sequencing technologies. This approach enables researchers to rapidly analyze large volumes of genetic material, greatly enhancing the throughput of genetic studies. However, while high throughput is advantageous, it can lead to challenges in data management. The shear volume of information can overwhelm traditional analysis methods, necessitating the integration of advanced computational techniques.
Protein Structure Characterization
Protein structure characterization is another area where spectrum resolution significantly contributes. It involves determining the three-dimensional structures of proteins, which is key to understanding their function. The ability to resolve fine details in protein structures can help in drug discovery and development, making it a crucial aspect of biomolecular research.
The key characteristic of this method lies in techniques such as X-ray crystallography and nuclear magnetic resonance. These techniques depend heavily on spectrum resolution to provide accurate structural insights. The unique feature of protein characterization is its capacity to reveal conformational changes in proteins. However, these methods also have their limitations. For instance, X-ray crystallography requires the protein to form crystals, which is not always possible for every protein of interest, potentially hindering research.
Environmental Science
Pollutant Detection
In environmental science, pollutant detection serves as a critical application of spectrum resolution. Identifying and measuring contaminants in air, water, or soil is essential for assessing environmental health. The key characteristic of pollutant detection methods is their sensitivity, which allows for the identification of trace levels of harmful substances. This makes it a beneficial choice for monitoring environmental quality and enforcing regulations.
A unique feature of pollutant detection systems is their integration with real-time monitoring technologies. These systems can provide immediate feedback, which is crucial in situations where timely intervention is needed. However, challenges remain regarding the specificity of detection methods. Some techniques may yield false positives, complicating data interpretation.
Climate Modeling
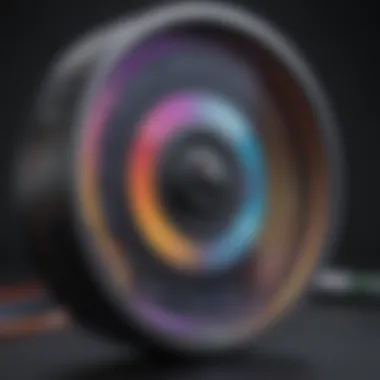
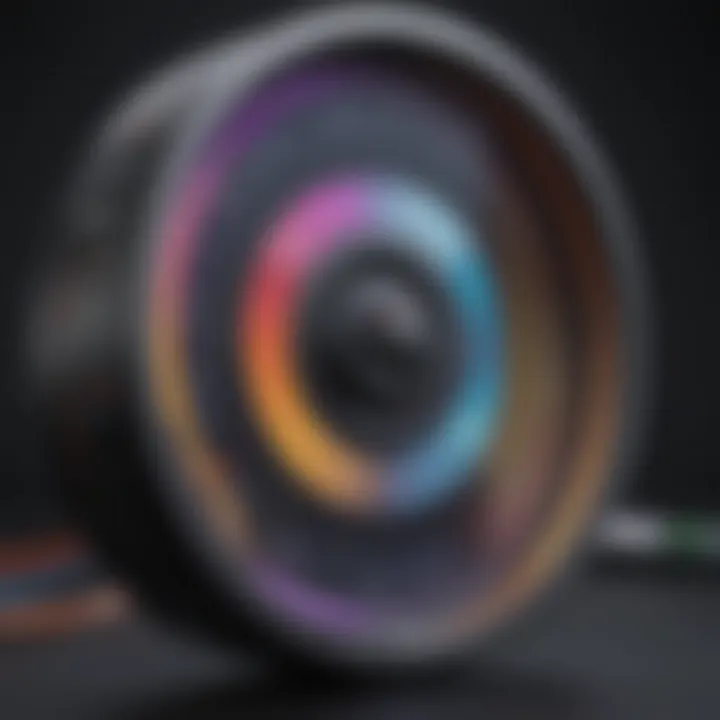
Climate modeling represents another significant application of spectrum resolution within environmental science. It helps scientists predict future climate changes based on current and historical data. The key characteristic of climate models is their ability to simulate complex interactions within the Earth's atmosphere, oceans, and land surfaces. This allows researchers to forecast climate scenarios, making it a popular tool in climate science.
A unique feature of climate modeling is its reliance on large-scale data integration from diverse sources. This interdisciplinary approach enhances the model's accuracy. However, one disadvantage is the inherent uncertainty in predicting climate behavior due to the complexity of environmental systems. Despite these challenges, the importance of spectrum resolution in climate modeling cannot be overstated; it is fundamental for optimizing models and improving predictions.
Challenges in Achieving Optimal Spectrum Resolution
Achieving optimal spectrum resolution is an essential aspect of analytical chemistry and related fields. It has numerous implications for data accuracy and interpretation. Despite various advancements in technology, certain challenges persist that can hinder the effective resolution of spectra.
These challenges stem primarily from both instrumental limitations and environmental factors. Each challenge brings to light the complexities involved in conducting precise analytical measurements and interpreting data successfully. It is crucial to understand these limitations if researchers and scientists aim to improve their methods and outcomes in spectrum analysis.
Instrument Limitations
Instrument limitations pose significant barriers to achieving optimal spectrum resolution. Instruments used for spectrum analysis often come with predefined specifications that can impact the quality of the data collected. For instance, the resolution of a spectrometer is defined as its ability to distinguish between two closely spaced wavelengths. If the hardware lacks the necessary sensitivity or has a limited range, the spectra obtained may be inherently flawed.
The following factors below influence instrumental limitations:
- Spectral Range: Instruments may not cover the entirety of the spectral range required for a given analysis.
- Detector Efficiency: The type and quality of the detector play a vital role in capturing data accurately. Low-efficiency detectors may not be able to pick up weak signals effectively.
- Calibration: Inaccurate calibration can lead to distorted results, significantly impairing the quality of the data.
Each of these aspects highlight the technical challenges involved. As technology develops, addressing these limitations will be imperative to improve spectrum resolution across various fields.
Environmental Factors
Environmental factors also contribute to challenges in achieving optimal spectrum resolution. These factors can introduce noise and inconsistencies in the readings, affecting the reliability of data interpretation.
Several examples illustrate how environmental variables interfere with spectrum resolution:
- Temperature Fluctuations: Variations in temperature can impact instrument performance. For example, certain spectrometers may require stable environmental conditions for accurate results.
- Vibration: Mechanical vibrations in the surroundings can lead to disruptions in readings, particularly in sensitive instruments like interferometers.
- Light Pollution: Unwanted ambient light can interfere with the detection process in spectrometry, influencing signal clarity.
Overall, navigating these environmental challenges is essential for obtaining accurate spectrum data. Researchers must develop protocols to mitigate the influence of these factors in their experiments, ensuring the highest possible spectrum resolution.
"Understanding both instrumental and environmental factors is key to mastering spectrum resolution techniques and improving data reliability in scientific research."
Addressing these challenges requires a multi-faceted approach combining improved technologies with a careful evaluation of the experimental environment.
Future Directions in Spectrum Resolution Research
The future of spectrum resolution research holds immense potential for advancing various fields of science. As technologies evolve, so too do the tools and methodologies for enhancing spectrum resolution. This area of inquiry is vital, not only for improving data accuracy but also for facilitating new discoveries in disciplines ranging from physics to biology. Researchers today must consider the implications of emerging techniques and interdisciplinary approaches which can reshape our understanding of spectral data.
Emerging Techniques
In recent years, several innovative techniques have come to the fore, promising to revolutionize spectrum resolution. These methods leverage advancements in technology to improve measurement accuracy, speed, and precision.
- Quantum Dots: These are semiconductor nanocrystals that emit light. They can enhance resolution in fluorescence-based methods, making them ideal for biological imaging.
- Super-resolution Microscopy: Techniques such as STED (Stimulated Emission Depletion) microscopy allow scientists to visualize structures at the nanometer scale. This level of detail was previously unattainable with conventional microscopy methods.
- Integrated Photonic Devices: These devices combine optics with electronic circuits to process spectral data with unprecedented efficiency. Such integration enhances signal processing and reduces noise, resulting in clearer data capture.
Adapting and perfecting these emergent techniques is key for future research initiatives. Scientists must understand their implications and appropriate applications. Each new method comes with unique challenges and considerations that must be addressed to ensure efficacy.
Interdisciplinary Approaches
Interdisciplinarity is another pivotal aspect of future spectrum resolution research. Collaboration between different scientific fields can yield a more holistic understanding of spectrum resolution and its applications. This convergence encourages the sharing of techniques and insights across disciplines.
For instance, researchers in environmental science might work closely with physicists to develop better sensors for pollutant detection. Likewise, biologists can benefit from physicochemical principles to enhance protein analysis. Some relevant elements of interdisciplinary approaches include:
- Shared Methodologies: Many fields use similar analytical techniques adapted for their specific needs. This exchange can be beneficial for refining methods applicable across various domains.
- Innovative Problem Solving: Combining perspectives from diverse fields often leads to creative solutions for complex problems that one discipline alone may not be able to tackle.
- Improved Funding Opportunities: Many funding organizations favor projects that demonstrate an interdisciplinary approach, which can lead to more resources for spectrum resolution innovations.
"Interdisciplinary research enriches our understanding and opens new avenues for collaboration, crucial for advancing spectrum resolution capabilities."
Ending and Summary
In the realm of spectrum resolution, understanding its nuances is paramount for researchers across various scientific fields. The dynamic nature of this topic influences a plethora of analytical techniques and applications. As this article has illustrated, the significance of spectrum resolution cannot be understated. Its role in enhancing data accuracy directly impacts research outcomes, making it a crucial consideration for scientists.
Moreover, spectrum resolution serves as a bridge between theory and application. It allows researchers to interpret data more effectively, thus deciphering complex information embedded in spectral data. Through the different methodologies discussed, such as spectroscopic techniques and computational methods, it becomes evident that improving spectrum resolution is an ongoing endeavor.
One must recognize that challenges exist in this pursuit. Instrument limitations and environmental factors can lead to significant variability in results. Addressing these issues is essential for the advancement of spectrum resolution research. \n Overall, the insights provided in this article contribute to a deeper understanding of spectrum resolution and its relevance in both present and future scientific explorations. Now let’s delve into the key takeaways from this exploration.
Key Takeaways
- Spectrum resolution is vital in ensuring the quality of data analysis, affecting both quantitative and qualitative research outcomes.
- Various techniques, such as nuclear magnetic resonance and mass spectrometry, are instrumental in enhancing resolution.
- Computational approaches, including machine learning and algorithms for data processing, have emerged as valuable tools in this field.
- Misinterpretation of data can occur without adequate resolution, leading to flawed conclusions.
- Future advancements in technology will likely foster better spectrum resolution, enhancing research capabilities.
Implications for Future Research
The future of spectrum resolution is promising, with ample opportunities for innovation. As new technologies emerge, researchers can expect more refined spectral analysis methods. Collaboration between disciplines such as physics, biology, and environmental science can yield valuable intersections in knowledge and technique.
Furthermore, with the rise of big data and machine learning, researchers can harness vast amounts of data for improved pattern recognition and predictive analytics in spectral analysis. This opens doors for interdisciplinary research, which has the potential to address complex challenges in spectrum resolution.
In addition, ongoing research into the limitations faced by current instruments and techniques will help refine the tools available. By incorporating feedback from practical applications, researchers can push the boundaries of what is achievable in spectrum resolution. Identifying these limitations is essential for fostering advancements that better serve diverse scientific applications.
In summary, the exploration of spectrum resolution highlighted its critical role in scientific inquiry. Understanding both the current practices and future directions provides a framework for enhancing research methodologies. It is essential that scientists across all disciplines remain aware of these developments to leverage the full potential of spectroscopic analysis.