The Essential Role of Semiconductors in Electronics
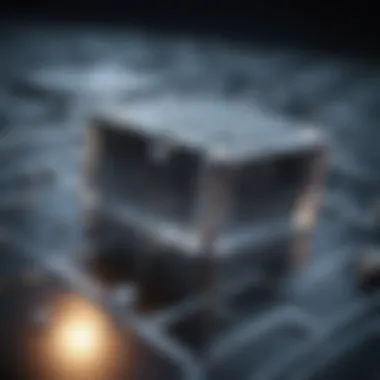
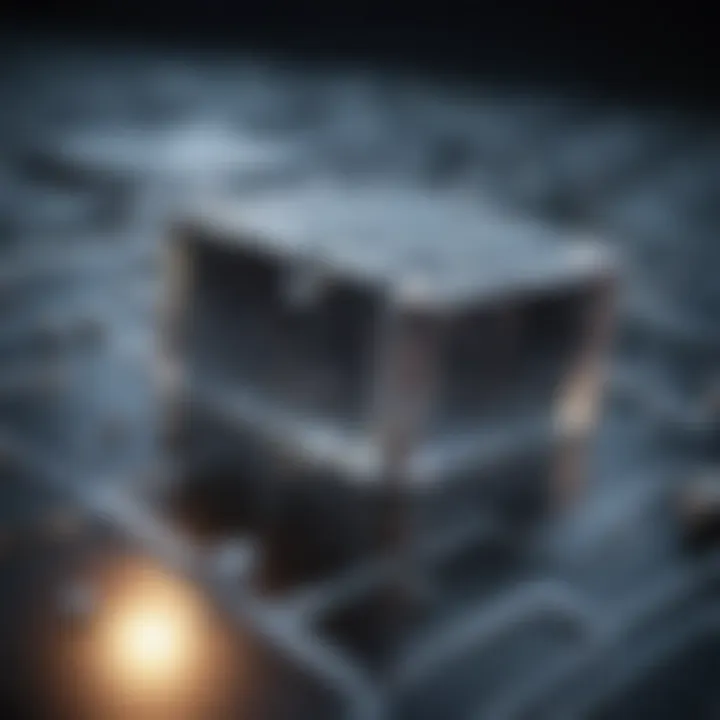
Intro
Semiconductors have become the lifeblood of modern electronic devices, enabling a range of functionalities from basic computations to intricate designs driving our daily lives. With an array of applications in everything from smartphones to electric vehicles, understanding the role and significance of semiconductors is paramount.
Overview of Research Topic
Brief Background and Context
The invention of the transistor in the mid-20th century marked the dawn of semiconductor technology. Historically, semiconductors arose from the necessity for materials that were neither insulators nor perfect conductors. Materials like silicon and germanium emerged as foundational components, having unique electrical characteristics that allowed control over electrical current.
As technology evolved, the ability to manipulate these properties became essential. This gave rise to integrated circuits, which are at the heart of nearly every modern electronic device. As they began to shrink in size while increasing in power, the term "Moore's Law" came into play, observing the exponential growth in the number of transistors that can fit on a chip.
Importance in Current Scientific Landscape
In today’s scientific landscape, semiconductors are not merely reactive components but proactive players in technological advancement. The rise of artificial intelligence, the Internet of Things (IoT), and renewable energy technologies hinges on semiconductor innovations. The synergy between hardware capabilities and software applications has never been more pronounced, showcasing their essential role in driving modern electronics forward.
"At its essence, the semiconductor industry is built on the premise that innovation is relentless, pushing the boundaries of what is possible in electronics."
Methodology
Research Design and Approach
In assessing the impact and future of semiconductors within electronics, a mixed-methods approach provides depth. By combining quantitative analysis of current market trends with qualitative research from industry experts, a more nuanced understanding emerges.
Data Collection Techniques
Data collection involves a dual approach. On one hand, market analysis leverages industry reports and sales data from leading semiconductor manufacturers. On the other hand, expert interviews yield insights on challenges and opportunities within the semiconductor landscape. Engaging with sources such as IEEE and the Semiconductor Industry Association offers both broad and specific perspectives on this dynamic field.
In laying this groundwork, we can delve into the numerous ways that semiconductors underpin modern technology—starting from their types and properties, extending to their production processes, and wrapping up with an analysis of the pressing challenges the sector faces.
Understanding Semiconductors
Understanding semiconductors is paramount when exploring modern electronics. These materials form the backbone of countless devices, influencing everything from tiny smartphones to massive data centers. The significance of semiconductors extends beyond just their physical properties; they embody a vital component in technology's push towards efficiency and innovation. Knowing how semiconductors conduct electricity, their types, and their specific characteristics lays the groundwork for comprehending their applications and the technology driving today’s world.
Definition and Characteristics
Semiconductors are materials whose electrical conductivity lies between that of conductors and insulators. This unique trait is primarily due to their bandgap, which is the energy difference between the valence band (where electrons reside) and the conduction band (where they can move freely). When certain conditions are met—like the introduction of energy or impurities—the semiconductor can conduct electricity. This is a defining characteristic that allows them to be engineered for specific tasks. For instance, silicon, the most widely used semiconductor, is particularly valued for its availability and thermal stability, making it a staple in electronic components.
Conductivity and Bandgap Concept
Conductivity in semiconductors is a function of temperature and impurity levels. The bandgap is crucial; a narrow bandgap allows easier electron movement, enhancing conductivity. Think of it as a hill—if the hill is low, it’s simple for an electron to climb over. Conversely, a larger bandgap makes it harder, resulting in less conductivity. The adjustment of temperature affects the number of charge carriers—electrons and holes—which determines a semiconductor’s efficiency in a device. This enabling factor is why semiconductors can be tuned for different applications, from everyday electronics to specialized functions in more complex systems.
Types of Semiconductors
There are generally two main categories of semiconductors: intrinsic and extrinsic.
Intrinsic Semiconductors
Intrinsic semiconductors consist purely of semiconductor material without any significant impurities. Their electrical properties are inherent and are shaped by the material itself, usually silicon or germanium. A key characteristic here is that their conductivity is relatively low at room temperature but increases significantly when temperature rises. This makes intrinsics useful in certain applications, like diodes, where high purity is critical. The unique feature is their natural electron-hole pair generation as heat excites the material. However, the main downside is their limited conductivity under standard conditions, which restricts their usability in some high-power applications.
Extrinsic Semiconductors
In contrast, extrinsic semiconductors are doped with specific impurities to enhance their electrical properties. This modification allows for the creation of n-type (negative-type) and p-type (positive-type) semiconductors, where the introduced impurities either add extra electrons or create holes. Notably, this versatility makes extrinsic semiconductors prevalent in modern electronics, accommodating a wide range of devices like transistors and integrated circuits. The ability to tailor a semiconductor’s properties results in greater control over its conduction performance, marking it as a popular choice in this article’s context. Nonetheless, the introduction of dopants can lead to challenges related to material consistency and stability over time.
Classes of Semiconductor Materials
In the realm of modern electronics, the category of semiconductor materials plays a critical role that cannot be understated. Semiconductors serve as the backbone for countless devices, facilitating their operations through the manipulation of electronic properties. By categorizing these materials, we gain a deeper appreciation of their specific advantages and applications, making it easier to understand their importance in technological advancement.
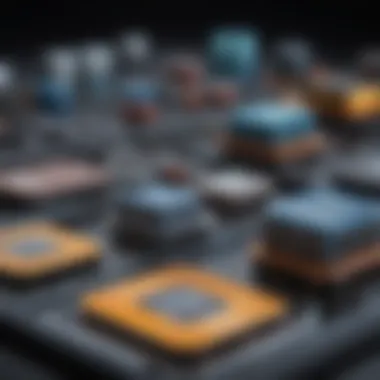
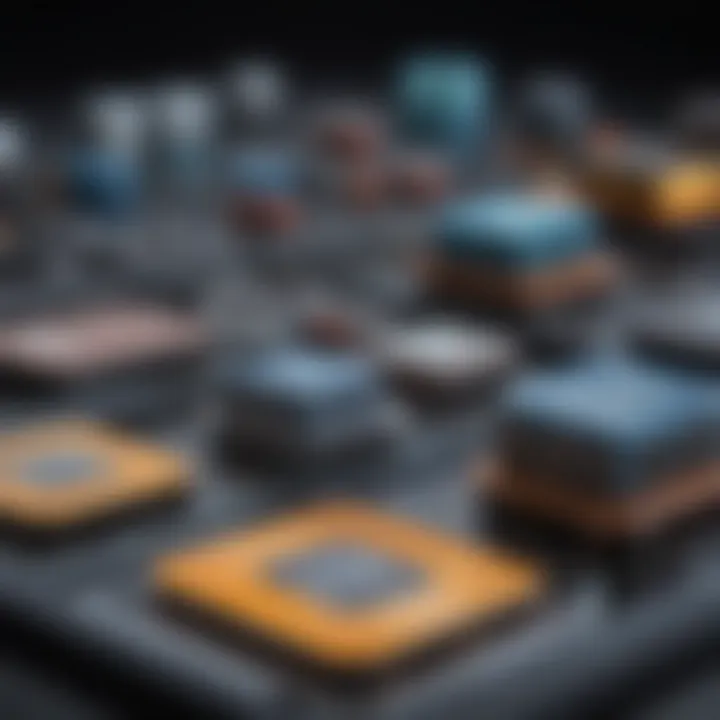
Silicon: The Dominant Material
Silicon is often termed the darling of the semiconductor world, thanks to its widespread availability and favorable electronic properties. It’s like the Swiss Army knife of semiconductors—versatile and reliable. With a bandgap of approximately 1.1 eV, silicon's ability to conduct electricity can be controlled through doping, making it a prime choice for a vast range of applications.
One reason for silicon's dominance is its abundance; it's the second most abundant element in the Earth's crust, which means it’s relatively inexpensive to produce. Moreover, silicon has a well-established manufacturing infrastructure, leading to advancements in processes such as photolithography, which helps create microelectronic devices with incredible precision.
Furthermore, as transistors shrink in size—thanks to Moore's Law—it’s silicon's advantageous properties that allow more functions to be packed into a smaller space without overheating or losing efficiency.
"Silicon's adaptability, from solar cells to computer processors, showcases its pivotal role in shaping modern technology."
Some key applications include:
- Microprocessors: The heart of computers, ensuring rapid computations.
- Solar Cells: Converting sunlight into electricity effectively.
- Sensors: Used in various devices to detect changes in the environment.
Despite its strengths, silicon does exhibit some limitations, particularly in high-frequency applications where the material's speed lags behind competitors. Nonetheless, its long-standing presence in the market cements its status as the go-to material for semiconductors.
Gallium Arsenide and Other Alternatives
While silicon may enjoy the spotlight, alternatives like Gallium Arsenide (GaAs) are making significant waves. This compound semiconductor boasts a higher electron mobility compared to silicon, allowing devices to achieve greater speeds and efficiency. GaAs is particularly favored in applications such as high-frequency ICs, optoelectronics, and solar cells, where efficiency is paramount.
However, GaAs does come with a few caveats: it is pricier and less abundant than silicon, which can hinder its applications outside niche markets. Nonetheless, its unique properties make it indispensable for certain cutting-edge technologies.
In addition to Gallium Arsenide, other materials such as Indium Phosphide and Silicon Carbide are also gaining traction, particularly in fields like wireless communications and semiconductor lasers. The choice of semiconductor material often boils down to the intended application and the physical properties required, such as thermal stability and efficiency.
Some advantages of GaAs and its counterparts include:
- Higher efficiency in solar cells compared to traditional silicon cells.
- Better performance in high-frequency applications, making them suitable for RF amplifiers.
- Optoelectronic devices like lasers which benefit from the direct bandgap of these materials.
Applications of Semiconductors in Electronics
Semiconductors form the backbone of modern electronics, as they are pivotal in facilitating the operation of numerous devices we rely on every day. Without them, the vast world of technology as we know it would be non-existent. From smartphones to laptops, and from medical devices to automotive systems, semiconductors play a crucial role in ensuring efficiency and performance.
This section dives into relevant areas where semiconductors shine brightest, shedding light on their indispensable applications that keep essential devices functioning smoothly. Understanding these applications not only highlights their importance but also underscores the need for advancements in semiconductor technology to meet the growing demands of an increasingly digital world.
Microprocessors and Microcontrollers
In today’s digital ecosystem, microprocessors and microcontrollers serve as the brains behind many electronic devices. Microprocessors act as central processing units (CPUs) in computers, executing a myriad of functions that range from basic calculations to complex, multitasking processes. They help run operating systems and software, making them crucial for functionality.
On the flip side, microcontrollers are compact integrated circuits designed for dedicated tasks. Found in household appliances like washing machines and coffee makers, they allow devices to perform specific functions reliably. The impact of these components is monumental:
- Efficiency: Devices become smarter and faster, reducing energy consumption.
- Versatility: Applications span from consumer electronics to industrial machinery.
- Customization: They can be programmed for specific tasks, allowing for tailored user experiences.
In essence, both microprocessors and microcontrollers are enabling devices to operate seamlessly, making our lives a little easier and more connected.
Semiconductor Devices: Diodes and Transistors
Diodes and transistors, the fundamental building blocks of semiconductor technology, play a critical role in the shaping of electronic circuits. A diode, which allows current to flow in one direction, is essential in applications such as power supply rectification and signal demodulation. They prevent backflow, ensuring that devices are protected against electrical anomalies.
Transistors, on the other hand, have revolutionized electronics by serving as switches and amplifiers. They have enabled the miniaturization of devices, facilitating the development of everything from radios to computers. The significance of these devices can be illustrated through key points:
- Switching capability: They allow for rapid on/off control in electronic circuits.
- Signal amplification: Transistors can boost weak signals for better transmission.
- Integration: Large numbers of transistors can be integrated into a single chip, leading to compact device design.
Both diodes and transistors allow for much more complex circuitry in devices, thus paving the way for advancements in technology.
Power Electronics and their Applications
Power electronics refer to the technology used to convert electrical power from one form to another. This includes controlling and converting electrical energy with high efficiency, aiding in power management for various applications. Their significance spans a wide array of sectors, such as:
- Renewable Energy: They are instrumental in the functionality of solar inverters and wind turbines, converting generated energy into usable power.
- Electric Vehicles: Power electronics contribute to battery management systems and power distribution, enhancing the performance and efficiency of electric vehicles.
- Consumer Appliances: They manage electrical energy in devices like refrigerators, air conditioners, and smart home systems.
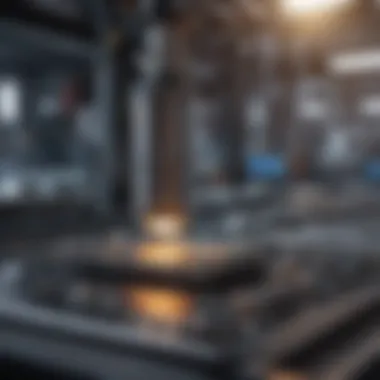
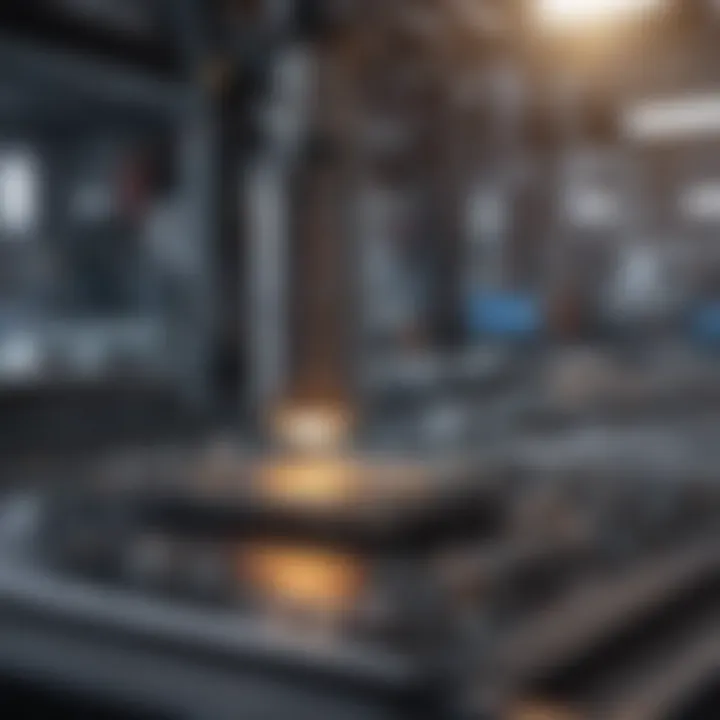
In a world increasingly geared towards sustainability, power electronics is a key player in optimizing energy use and reducing waste.
Semiconductors in Communication Technologies
The field of communications has dramatically evolved, and semiconductors have been at the forefront of this progress. They facilitate critical aspects of telecommunications and networking, providing the necessary infrastructure for seamless data transmission. Various applications illustrate this:
- Signal processing: Semiconductors are essential for modulating and demodulating signals in mobile and broadband communications.
- Networking hardware: Devices such as routers and switches depend on semiconductor components to manage and direct data traffic efficiently.
- Wireless Communication: From Bluetooth to Wi-Fi, semiconductors enable robust connections that define modern connectivity.
As communication technology continues to advance at a breakneck pace, the role of semiconductors has never been more critical. Their capability to drive innovation in this arena is undoubtedly significant, influencing how we interact in an ever-connected world.
"In a tech-driven society, semiconductors represent not just components, but the very foundation of innovation and connectivity."
The Manufacturing Process of Semiconductors
The manufacturing process of semiconductors is a fundamental pillar in the realm of modern electronics. It is the complex journey from raw materials to functional microchips that shapes the capabilities of electronic devices we use every day. Understanding this process not only reveals the intricacies involved but also highlights the importance of each step in achieving high-performance output suitable for various applications. Given the rapid advancements in technology, knowing how semiconductors are fabricated provides context for the innovations we are witnessing today.
From Raw Silicon to Wafer Production
The journey of semiconductor creation begins with silicon, the cornerstone of the industry. Silicon is an abundant element and serves as an ideal base material due to its favorable electrical properties. The first step involves purifying silicon to high purity levels—generally 99.9999% or more—through processes such as the Czochralski method or the Siemens process.
Once purified, the silicon is melted and formed into cylindrical ingots. These ingots are then sliced into thin discs, known as wafers.
- Wafers act as the substrates for the subsequent processing steps.
- They must be polished to achieve a mirror-like finish, allowing for optimal photolithographic results in later stages.
This phase is critical because any imperfections can compromise the integrity of the finished electronic components. Each wafer must undergo rigorous cleaning to eliminate contaminants, ensuring that they are ready for doping and etching processes.
Doping Techniques Explained
Doping is the technique used to modify the electrical properties of silicon. By introducing small amounts of impurities, or dopants, such as phosphorus or boron, manufacturers can create n-type or p-type semiconductors.
- N-type semiconductors are infused with elements that provide extra electrons, enhancing conductivity.
- P-type semiconductors have elements that create holes or deficiencies in electrons, which also aid in conducting electricity.
The process of doping can be performed using several methods:
- Diffusion: involves placing the wafers in a chamber with gaseous dopants, allowing them to penetrate the silicon surface.
- Ion Implantation: involves accelerating ions of dopants into the substrate using high-energy beams, providing precise control over the depth and concentration of dopants.
Both methods require fine-tuning to ensure that the electrical properties of the resulting semiconductors meet specific performance criteria.
Photolithography and Its Role in Fabrication
Photolithography is a crucial step in semiconductor manufacturing, enabling the creation of intricate circuit patterns on the silicon wafers. The process begins by applying a light-sensitive material called photoresist to the wafer's surface.
- After the photoresist is spun onto the wafer, it is exposed to ultraviolet light through a mask containing the circuit design.
- The exposed areas undergo chemical changes, allowing for selective removal of the resist when processed.
Following this, etching techniques are employed to carve out the desired patterns into the silicon. Here are a few techniques commonly used:
- Wet etching: involves chemical solutions that remove material from the wafer.
- Dry etching: uses plasma to create more precise and detailed patterns.
These patterns are critical for defining the structure of transistors, capacitors, and interconnections that comprise semiconductor devices. Moreover, photolithography continues to evolve, with the advent of extreme ultraviolet (EUV) lithography pushing the limits of miniaturization in semiconductor design.
"The precision of photolithography directly influences the performance and efficiency of modern electronic devices."
Challenges Facing the Semiconductor Industry
The semiconductor industry stands as a backbone of modern electronics, driving technological advancements across various sectors. However, this sector is currently navigating through a myriad of challenges that could significantly impact its future development. Addressing these difficulties is crucial not only for the sustainability of semiconductor production but also for the overall progress of electronics.
The challenges are multifaceted, encompassing supply chain disruptions that hinder production efficiency and pose risks to the timely delivery of critical components. At the same time, the industry grapples with environmental concerns, as the manufacturing processes can produce substantial waste and emissions, compelling companies to adopt more sustainable practices.
Understanding these challenges allows for a deeper insight into how the semiconductor industry can innovate and adapt to continue supporting the evolving landscape of technology.
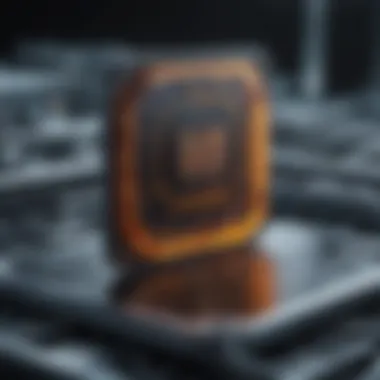
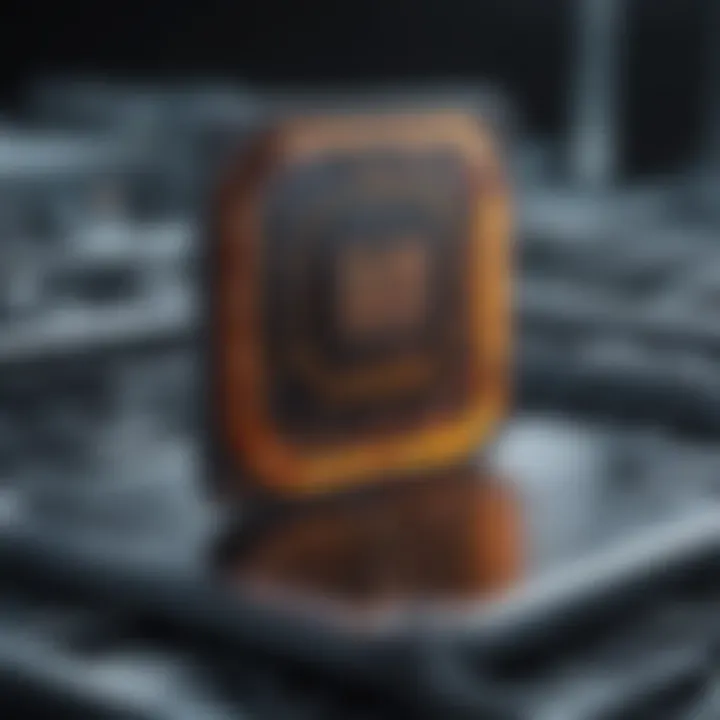
Supply Chain Disruptions
In recent years, the semiconductor industry has been no stranger to supply chain complexities. A unique combination of factors has resulted in significant hiccups in the supply chain. These factors range from global events like health crises, such as the COVID-19 pandemic, to political tensions and trade restrictions.
"The semiconductor shortage we faced globally highlighted not just the fragility of our supply chains but also our dependence on a few manufacturing hubs."
The consequences of these interruptions can be dire. For instance:
- Production Halts: Manufacturers may find themselves unable to produce essential electronics, leading to lost revenue.
- Increased Prices: With supply outstripping demand, costs of semiconductors have soared, affecting the pricing of finished products.
- Innovation Stifling: Start-ups and smaller companies might struggle to keep up rather than innovate due to uncertainty in obtaining the necessary components.
Finding solutions to these disruptions requires collaboration across the industry. Companies are now re-evaluating their supply strategies, exploring options for diversification, and investing in local manufacturing to mitigate future risks.
Environmental Concerns and Sustainable Practices
As the semiconductor industry grows, so does its environmental footprint. The production of semiconductors can be resource-intensive, consuming significant amounts of water and energy while generating toxic waste. Consequently, the industry faces increasing pressure to adopt sustainable practices.
In response to these environmental concerns, some key considerations include:
- Efficient Waste Management: Efforts to reduce waste and recycle materials are vital. For instance, techniques like wafer recycling minimize resource consumption.
- Green Manufacturing: Companies are starting to implement greener production methods. This includes utilizing renewable energy sources and seeking eco-friendly materials in manufacturing processes.
- Lifecycle Assessment: Understanding the environmental impact throughout the product lifecycle can guide better design and manufacturing choices.
Moreover, customers today are becoming more aware of the ecological implications of their purchases. Companies that prioritize sustainability may find themselves at a competitive advantage, as consumers increasingly favor environmentally responsible brands.
By tackling these environmental challenges, the semiconductor industry won’t just aid the planet but could also inspire innovation that leads to more efficient, sustainable technologies.
The Future of Semiconductor Technology
The future of semiconductor technology is a hotbed of innovation and critical for driving progress across all electronic sectors. As technology advances, semiconductors become even more intricate and essential in our daily lives. The convergence of various fields—like computing, communication, and energy—demands a continuous evolution in semiconductor capabilities. This urgency is not only about increasing processing speeds or reducing power consumption, it's also about developing systems that can handle unprecedented amounts of data efficiently and securely.
Emerging Technologies: Quantum Computing
Quantum computing represents one of the most promising facets of semiconductor innovation. Unlike classical computers which process data in binary (1s and 0s), quantum computers utilize qubits that can exist in multiple states simultaneously. This capability potentially enables quantum machines to execute complex calculations far faster than their classical counterparts. However, achieving stable qubits is a significant challenge. They're fragile, requiring conditions usually found only in highly controlled environments.
This is where semiconductors play their role. Materials such as superconducting circuits or semiconducting nanostructures are critical in developing viable qubits. Researchers are exploring innovative approaches, including topological qubits, which may offer greater stability and error resistance, enhancing the feasibility of quantum systems.
Additionally, industries are investing heavily in integrating quantum computing capabilities with existing technologies. Companies are forming partnerships to share knowledge and resources, aiming to push quantum computing from experimental labs into real-world applications. It's a fascinating horizon for the semiconductor sector as it navigates the nascent but promising terrain of quantum technology.
Advancements in Materials Science
The field of materials science is undergoing a renaissance, particularly in semiconductor technology. While silicon remains the cornerstone, new materials are being explored to overcome silicon's limitations. For instance, graphene and two-dimensional materials offer unique electronic properties that could revolutionize device design.
Advantages of new materials include:
- Higher electron mobility, allowing for faster electronics.
- Flexibility, opening the door to wearable tech and flexible displays.
- Lower energy consumption, a key consideration as we face growing energy demands.
Moreover, compound semiconductors such as gallium nitride (GaN) and silicon carbide (SiC) have gained traction, particularly in power electronics. They're ideally suited for high-voltage applications, making them indispensable in electric vehicles and renewable energy solutions. The ongoing research into enhancing and integrating these materials wills foster more efficient semiconductor components, pushing the boundaries of what's conceivable in technology design.
"The advancements in materials science are not merely incremental improvements; they represent a paradigm shift in how we build and think about electronics."
Returning our focus to the broader implications—these advancements not only hold promise for improved performance but also inherently address broader concerns, such as resource efficiency and sustainability. As the semiconductor industry straddles the line between performance and environmental implications, the future appears ripe with potential.
Culmination
Significance of Semiconductors in Technology
Semiconductors stand at the heart of contemporary electronics and play a pivotal role in various technological advancements. Their unique properties, such as the ability to conduct electricity under certain conditions, make them essential for building everything from basic circuits to sophisticated microchips that power modern devices. Without them, the technological landscape would look much different; our smartphones, computers, and even appliances would not function as we are accustomed to them.
The expansive field of electronics heavily relies on semiconductors due to their versatility and efficiency. Their integration in integrated circuits allows for miniaturization, which is crucial in today’s fast-paced tech world. In addition, semiconductors enable processing speeds and energy efficiencies that are vital for powering AI systems, improving the performance of renewable energy sources, and facilitating communication technologies.
Reflection on Future Developments
Looking ahead, the trajectory of semiconductor technology is poised for remarkable changes. The possibility of quantum computing represents a leap forward, as it holds the potential to solve problems at speeds unattainable by classical computers. Similarly, advancements in materials science could lead to the development of more efficient and sustainable semiconductors, ultimately reducing the environmental impact of manufacturing processes.
Moreover, innovations like flexible electronics and bio-compatible semiconductors open new paths in healthcare and wearable technology. As we venture into an era of increasing connectivity and smart devices, the significance of semiconductors will only intensify, making their role even more critical for the infrastructure of future advancements.
"The future of semiconductors is not just about improving existing technologies but entirely reshaping how we interact with the world."