Quantum Computing: Principles and Future Applications
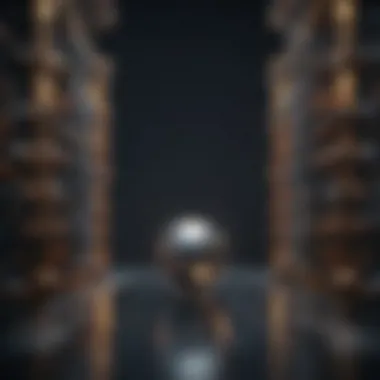
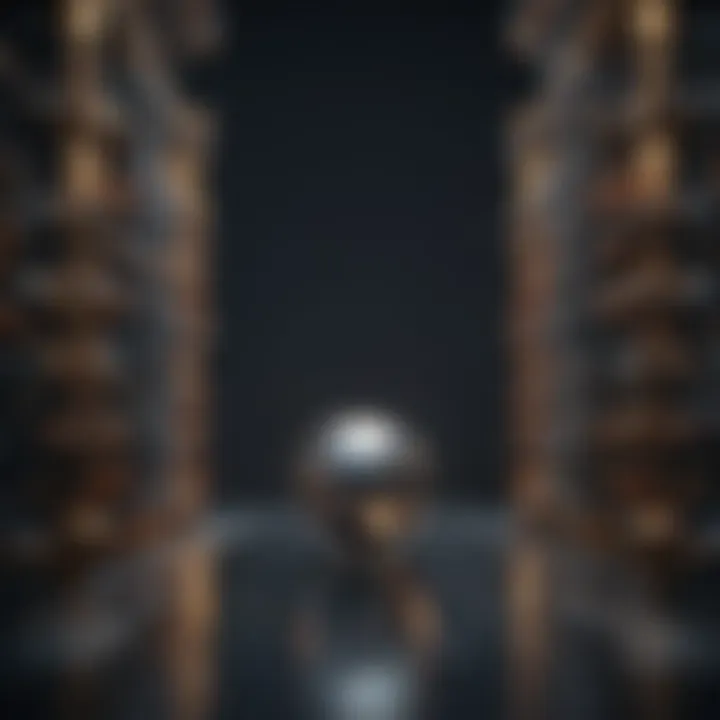
Overview of Research Topic
Brief Background and Context
Quantum computing stands as a revolutionary shift in computation, primarily leveraging the principles of quantum mechanics—those fundamental rules that govern microscopic particles. Unlike classical computers that use bits as the smallest unit of information, quantum computers employ qubits. Each qubit possesses the unique ability to exist in multiple states simultaneously, thanks to quantum phenomena like superposition and entanglement. This allows quantum computers to perform complex calculations at speeds previously deemed unattainable.
Despite being in the experimental stages of researched, ongoing advancements hint at vast implications. From cryptography to complex simulations in quantum chemistry, the horizon stretches far and wide, leaving much ground unexplored.
Importance in Current Scientific Landscape
In today's rapid technological progress, quantum computing isn't merely a niche topic restricted to research laboratories—it's at the forefront of scientific inquiry. The race for quantum supremacy—a term popularized recently—illustrates just how crucial these systems are becoming for various sectors. Enabling solutions for problems that baffle classical computing could unravel new potentials in artificial intelligence, drug discovery, and climate modeling. For instance, a significant milestone was reached when Google announced they had achieved quantum supremacy, a feat showcasing a task completed in a matter of minutes with a quantum computer that would take classical machines thousands of years.
In essence, the stakes are high in the quantum race. Engaging with quantum computing now carries significant weight, both for academia and industry, as researchers and businesses alike embark on a quest toward a quantum-powered future.
Methodology
Research Design and Approach
To fully explore the intricacies of quantum computer systems, a qualitative research design will be adopted. This involves a comprehensive literature review, examining peer-reviewed articles, conference papers, and ongoing research projects. The goal is to integrate varied perspectives, from theoretical foundations to practical applications, establishing a rich tapestry of knowledge.
Data Collection Techniques
The information will be gathered through a variety of techniques:
- Literature Review: Scrutinizing published papers in quantum physics and information technology journals.
- Interviews: Engaging with experts in the field to gain insights into practical implementations and current challenges.
- Case Studies: Analyzing existing quantum computer systems to discern their applied uses and observed limitations.
This tripartite approach ensures a well-rounded understanding, bridging the gaps between theoretical knowledge and practical applications, preparing the stage for a deeper exploration into the principles, applications, and future prospects of quantum computing.
Preamble to Quantum Computing
Understanding quantum computing is like stepping through a looking glass into a realm where the conventional rules of computation bend and fold. At its core, quantum computing harnesses the principles of quantum mechanics to process information in fundamentally novel ways. Recognizing the significance of this technology is paramount, as it stands to alter our conception of problem-solving and data processing across countless domains, from cryptography to drug discovery.
Unlike traditional computers that rely on bits as the smallest unit of information, quantum computers utilize qubits. These qubits can exist in multiple states simultaneously, which leads to an exponential increase in processing power. Delving into quantum computing is not merely an academic endeavor; it's an exploration of a potential future, ripe with advantages and challenges.
Defining Quantum Computers
In simple terms, quantum computers are devices that use quantum-mechanical phenomena to perform operations on data. The standout feature is the qubit, which, unlike a classical bit that can only be in a state of 0 or 1, can be in a state of both at the same time due to superposition. This property allows quantum computers to process a vast amount of possibilities concurrently.
"A quantum computer doesn't just solve problems; it fundamentally changes the way we understand computation."
A clear example of a qubit could be a spinning coin. When a coin is tossed, while it’s in the air, it doesn’t just land on heads or tails; it has the potential to be in a state of both until observed. This analogy illustrates the power of superposition in quantum mechanics, shaping how quantum computers operate.
In practical aspects, quantum computers promise to fast-track tasks that are currently daunting for even the most advanced classical systems. They may provide efficient solutions to complex problems like prime factorization, which is critical to cryptographic systems, thus redefining the landscape of digital security.
Historical Context
The journey toward quantum computing began with theoretical musings by physicists and computer scientists in the late 20th century. Pioneering work in the late 1980s by figures like Richard Feynman and David Deutsch articulated how quantum mechanics could compute information differently than classical systems. They sparked a revolution in thought about computation.
As interest burgeoned, the late 1990s witnessed practical demonstrations of quantum algorithms, such as Peter Shor's algorithm for integer factorization, showcasing that quantum computers could outperform classical equivalents in certain scenarios. This breakthrough laid the groundwork for the first rudimentary quantum algorithms, igniting further investment and research into quantum hardware.
Fast forward to today, many elite universities and organizations, such as IBM and Google, are in a fierce race to develop quantum computers that could one day be widely accessible. Yet, this excitement comes tethered to challenges and skepticism about the technology’s maturity and practicality.
In sum, a grasp of quantum computing's roots helps us appreciate its evolution and the transformative impact it may have in the years to come. Not just a field of science fiction, quantum computing is bound to redefine, well, how we compute.
Fundamental Principles of Quantum Computing
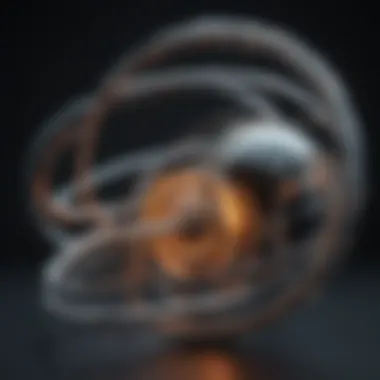
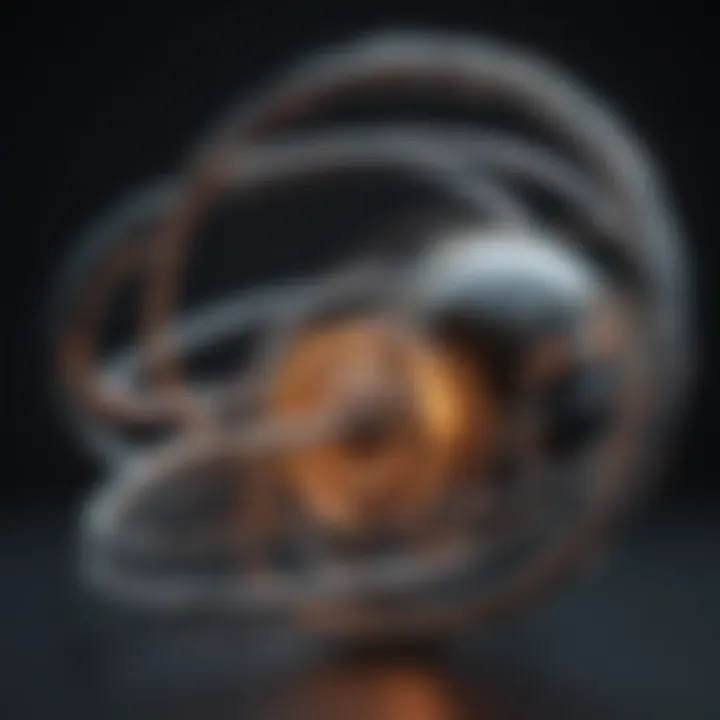
The fabric of quantum computing is woven from intricate principles that set it apart from classical computing paradigms. Understanding these principles lays the groundwork for appreciating the revolutionary potential that quantum systems hold. They form the backbone for innovations that can address complex problems in ways traditional systems cannot. By exploring qubits, quantum superpositions, and entanglement, we shed light on the fundamental mechanics that enable quantum computers to thrive.
Qubits and their Significance
At the heart of quantum computing lies the qubit, or quantum bit. Unlike conventional bits, which exist solely as either a zero or a one, qubits can inhabit a state of both zero and one simultaneously. This characteristic is not just a quirk of physics; it's a game-changer. Qubits can be realized using various technologies, such as superconducting circuits, trapped ions, and even photons. Each of these technologies has its strengths and weaknesses.
The significance of qubits extends beyond their dual-state nature. For instance, a system of just a few qubits can represent an astonishing number of states. To illuminate this, consider:
- Exponential Growth: A classical computer with n bits can process 2^n states, while a quantum computer with n qubits can manipulate 2^n states simultaneously. This means that even a modest quantum system can outperform classical counterparts in specific tasks.
- Parallelism: With multiple qubits working in concert, quantum computers can tackle problems through parallel processing, leading to significant reductions in computation time.
In essence, the qubit opens the door to a realm of possibilities that classical bits simply can’t access.
Quantum Superposition Explained
Quantum superposition is another cornerstone of quantum computing. It refers to a qubit's ability to exist in multiple states at once. Imagine flipping a coin. While it spins in the air, it isn't just heads or tails; it exists in a superposition of both until it lands. In quantum computing, this means a qubit can explore a multitude of solutions all at once, rather than sequentially as in classical systems.
This principle enables quantum computers to potentially solve complex problems that would take classical computers eons to compute. For instance:
- Search Algorithms: Through the advantage of superposition, quantum computers can search unsorted databases in considerably less time than classical methods. Grover's algorithm highlights just such an advantage - it reduces the number of required operations significantly.
- Optimization Problems: Many problems, like those found in logistics or financial modeling, require evaluating countless combinations of options. Quantum superposition allows for exploring all these combinations simultaneously, which can lead to optimal solutions much faster.
Understanding Quantum Entanglement
Quantum entanglement takes the principles of quantum mechanics to another level and stands as a testament to the non-intuitive nature of the quantum realm. When qubits become entangled, the state of one qubit becomes intrinsically linked to the state of another, regardless of the distance separating them. If one qubit is measured, its state instantly influences the state of its entangled partner - a phenomenon that perplexes even the most seasoned physicists.
The significance of quantum entanglement in computing cannot be overstated. Here’s how it adds value:
- Faster Communication: Quantum entanglement can lead to faster data transfer rates between qubits, improving the processing speed of quantum algorithms.
- Secure Communication: This property is also the backbone of many quantum encryption protocols, enhancing security through principles like quantum key distribution, ensuring that any attempt to eavesdrop disturbs the system and alerts the communicating parties.
In summary, the fundamental principles of quantum computing—qubits, superposition, and entanglement—represent not just technical concepts but foundational elements driving the next frontier of computational capabilities. The potential implications are vast, both in theoretical development and practical applications. As we turn our focus to the architectures that utilize these principles, it's clear that we are merely scratching the surface of what quantum computing can achieve.
Architectures of Quantum Computer Systems
The architecture of quantum computer systems serves as the backbone for how they operate and harness the unique principles of quantum mechanics. Understanding these architectures is crucial, as they influence not only performance but also the practical applications of quantum computing. Each architecture path holds unique strengths and is suitable for different types of computational tasks. The choice of a particular quantum computing architecture can dictate efficiency levels, scalability, and even feasibility for particular problems. In this section, we will examine three major architectures: Gate-Based Quantum Computing, Quantum Annealing, and Topological Quantum Computing.
Gate-Based Quantum Computing
Gate-based quantum computing, often compared to classical computing's logic gates, is at the forefront of quantum computer architecture. In this setup, operations are performed via quantum gates, which manipulate qubits in a controlled manner. The beauty of this architecture lies in its flexibility: it can simulate any quantum algorithm by constructing a quantum circuit.
Some notable advantages include:
- Universality: This architecture can theoretically perform any computation that can be expressed in quantum terms.
- Error Correction: Specific gate-based systems allow for intricate error-correcting codes, which are critical for maintaining the integrity of computations over time.
- Mature Technology: Many existing technologies, being focused on quantum gates, are feeding directly into potential future applications such as quantum supremacy.
However, it does have its drawbacks. Significant overhead in the form of control electronics and the complexity of compiling high-level algorithms into qubit operations can limit system performance.
Quantum Annealing
Quantum annealing focuses on solving optimization problems more efficiently than classical counterparts by leveraging quantum fluctuations. This architecture employs a process analogous to the classical annealing in metallurgy, gradually cooling a material to reach a state of minimum energy. In quantum terms, this translates to navigating the energy landscape of a problem to find its optimal solution.
Key features include:
- Specialization: Well-suited for specific optimization problems, such as those found in logistics and operations research.
- Low Energy Consistency: The system can potentially deliver consistent solutions while consuming less energy compared to classical methods.
- Robust to Noise: While classical systems can be heavily impacted by noise, quantum annealing can exploit quantum effects to navigate solutions even in chaotic environments.
On the flip side, it’s not a one-size-fits-all solution. Its limitations arise particularly when dealing with more generalized computational problems, where it lacks flexibility compared to gate-based systems.
Topological Quantum Computing
Topological quantum computing is a novel and somewhat experimental approach that utilizes topological states of matter. It functions on the principle that certain types of particles, known as anyons, exhibit unique non-abelian statistics which can be harnessed for quantum computation. The fundamental advantage of this architecture is its inherent resistance to decoherence.
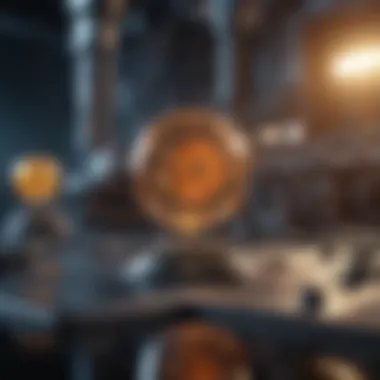
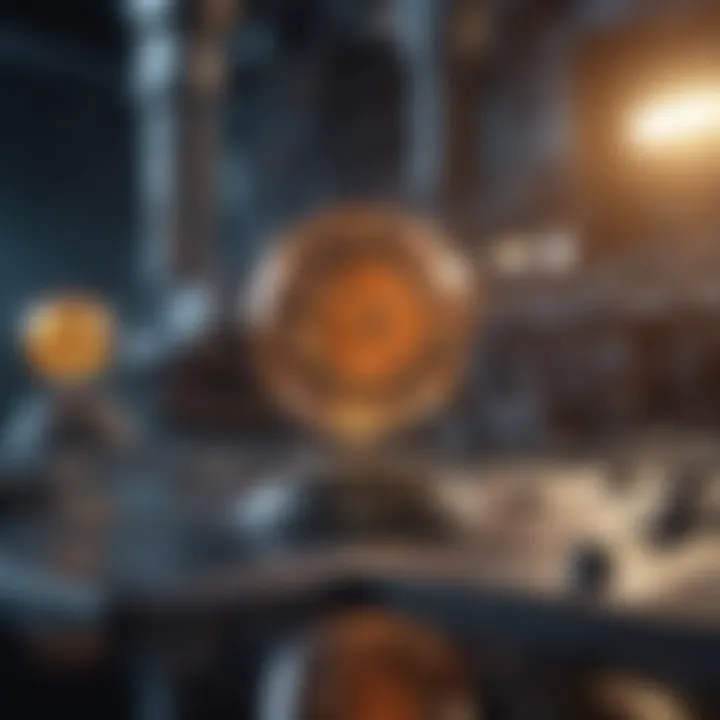
Advantages include:
- Stability: It boasts high fault tolerance due to the braiding of anyons—meaning quantum information can be preserved even in the face of dimensional noise.
- Scalability: In theory, this approach might scale more effectively than traditional qubit systems, as it requires fewer physically distinct components for computations.
- Universal Computation: Through the right braiding patterns, all quantum gates can be represented, implying maximum theoretical capability.
Challenges remain, notably the complexity of creating and manipulating anyons in practice; research into materials and implementations continues to pave the way forward.
Understanding these architectures allows researchers and enthusiasts alike to appreciate the diverse landscape of quantum computing. By tapping into varied strategies, the field can harness the sophisticated quantum mechanics principles and translate them into computational prowess.
Applications of Quantum Computing
The applications of quantum computing are as vast as they are transformative. This section shines a light on how quantum technologies have the potential to revolutionize different sectors, sparking a change in how we approach complex problems. From enhancing cybersecurity to tackling intricate optimization challenges, quantum computing stands at the cusp of a technological renaissance.
Cryptography and Security
One of the standout applications of quantum computing is in the realm of cryptography. Traditional encryption methods, such as RSA (Rivest-Shamir-Adleman), rely on the difficulty of factoring large numbers—a task that's monumental for classical computers. However, quantum computers leverage Shor's algorithm, allowing for the efficient factoring of these numbers. This could render conventional encryption methods obsolete, sparking a necessary shift towards quantum-resistant algorithms.
"With one hand tied behind its back, classical computers face a formidable enemy in quantum systems when it comes to encryption."
- Quantum Key Distribution (QKD) offers a potential solution. Through techniques like BB84, it allows for the creation of encryption keys that are theoretically impervious to interception. Security is ensured by the laws of quantum mechanics. If someone tries to observe the key, it changes, alerting the parties to potential eavesdropping. This paves the way for enhanced security protocols that can withstand not just current eavesdropping techniques, but future ones as well.
Optimization Problems
When companies faced with juggernaut challenges in logistics, manufacturing, and finance, they often find themselves hitting brick walls. Classical algorithms can take eons to provide the optimal solution, which is where quantum computing shines. It introduces new methodologies for solving optimization problems, such as through the use of quantum annealers.
For instance, the Travelling Salesman Problem (TSP), where one has to find the shortest route that visits a set of locations, transforms from a Herculean task into something more achievable. Quantum algorithms can scan myriad possibilities much faster than their classical counterparts, allowing solutions to emerge in a fraction of the time.
- Applications include:
- Supply chain management
- Investment portfolio optimization
- Traffic flow optimization
Quantum Simulations
The capacity for quantum simulations to model complex systems is another promising application of quantum computing. Quantum mechanics governs the behavior of subatomic particles, which makes classical simulations often infeasible for specific types of problems. For example, studying molecular interactions in chemistry or the quantum states of materials becomes significantly more efficient.
- In the pharmaceutical industry, quantum simulations can expedite the discovery of new drugs by providing insight into molecular structures and interactions that classical computers simply cannot handle effectively. This accelerates research processes, leading to faster innovations that can save lives.
- In the field of materials science, simulations can help in developing superconductors or new alloys, revealing emergent properties before these materials are physically created—this can drastically reduce the time and resources needed for experimentation.
The versatility and power of quantum computing applications make it not just a promise for the future but a reality waiting to be harnessed. Many sectors will benefit, fundamentally changing perceptions and methods of tackling problems. As research continues, it will be thrilling to see how these applications evolve and further influence technology, business practices, and daily life.
Challenges in Quantum Computing
As quantum computing continues to burgeon, the journey is hardly a walk in the park. Understanding the inherent challenges that are part and parcel of this technology is crucial, as it frames the issues that need navigating for realizing its true potential. Quantum computing promises to radically change the landscape of computation, but not without facing considerable hurdles. These challenges aren’t just technical; they have profound implications for the advancement and acceptance of quantum technologies.
Technical Challenges and Hardware Limitations
Building a functional quantum computer is akin to assembling a puzzle with pieces that keep shifting. The very nature of qubits, the fundamental unit of quantum information, makes them delicate and prone to errors. Their sensitivity to environmental disturbances poses a major roadblock. Currently, quantum computers require extremely low temperatures to operate, which demands sophisticated cooling technologies and materials.
Some of the key technical challenges include:
- Scalability: As more qubits are added, maintaining coherence becomes increasingly complex. The interactions among qubits can lead to complications like cross-talk, which hinders performance.
- Error Rates: High error rates in quantum operations mean that even the best-designed quantum circuits can yield unreliable results. Error correction methods exist but they require overhead resources that limit practicality.
- Materials and Fabrication: Developing reliable and efficient materials for qubits, such as superconducting circuits or trapped ions, is crucial. Each material brings its own set of challenges, from fabrication difficulties to integration issues.
Quantum Decoherence
Quantum decoherence is like the thief that steals the magic of quantum superposition. It occurs when qubits lose their quantum state due to interaction with the environment. This phenomenon fundamentally limits the time during which quantum operations can be performed, presenting a significant barrier to effective computation. The coherence time—the period a qubit can stably retain its state—must be maximized, but achieving this is no easy task.
Factors contributing to decoherence include:
- Electromagnetic Interference: External electromagnetic fields can disrupt qubit states, necessitating stringent isolation and shielding measures.
- Thermal Fluctuations: Too much heat can introduce noise, making it vital to maintain a chilled environment for qubit stability.
- Material Imperfections: Defects in the materials used for qubits can induce additional decoherence, therefore emphasizing the need for high-quality, defect-free materials.
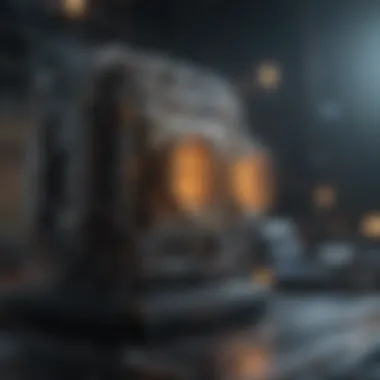
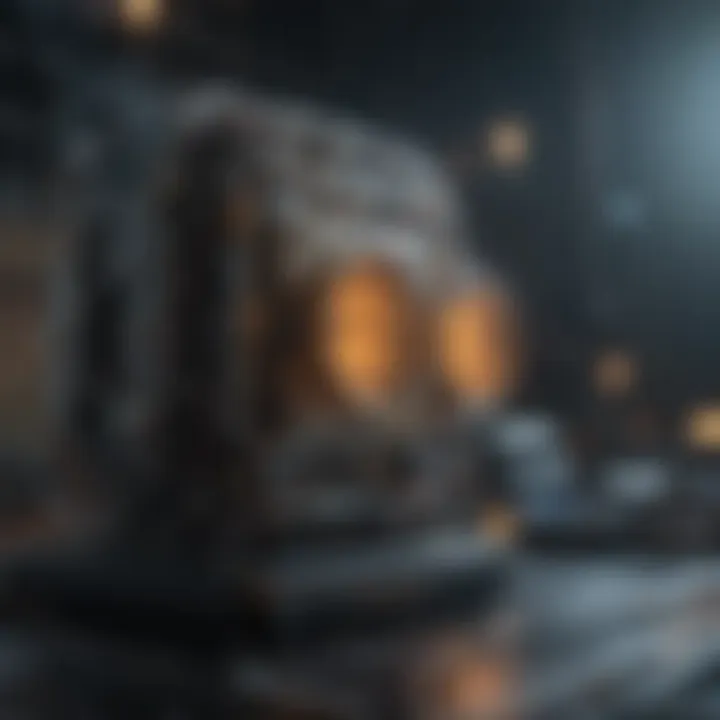
"Decoherence is the dark side of the quantum world, overshadowing our goal to harness its potential."
Algorithmic Limitations
Despite the theoretical superiority of quantum algorithms, in practice, they are often hampered by their own limitations. The speedup that quantum computing may offer is not universal across all types of problems. Many problems still lean heavily on classical algorithms for efficiency, posing a dilemma for researchers.
Some notable algorithmic challenges include:
- Problem Suitability: Not all problems are suited for quantum computing. For instance, tasks that rely heavily on linear programming may not see substantial benefits from quantum algorithms.
- Optimal Qubit Utilization: Many quantum algorithms require a well-structured approach to leverage the maximum power of qubits. Poor designs may lead to suboptimal outcomes.
- Research and Development: The current understanding of quantum algorithms is still maturing. Many potential algorithms remain theoretical, with little practical implementation.
In summary, the challenges presented in quantum computing are vast and multifaceted. Addressing these complexities is essential not just at a technical level but also impacts the way we perceive and integrate quantum technology into society. The promise of revolutionizing computation is real, but must be pursued with an understanding of the hurdles involved.
Future Prospects of Quantum Computing
The future of quantum computing stands at a fascinating crossroads, where innovation meets uncertainty. As technological advancements accelerate, it is crucial to assess how quantum systems will evolve and create ripples through multiple sectors. With the profound changes quantum computers promise, understanding their trajectory becomes essential for academia, industry, and society as a whole. This section dives deep into three key areas shaping the future of quantum computing: ongoing research trends, societal impacts, and the ethical considerations that accompany these monumental changes.
Trends in Research and Development
In the realm of research, quantum computing enjoys a vibrant landscape, filled with promising explorations. Institutions, universities, and tech companies worldwide are pooling resources, focusing on areas such as error correction, algorithm development, and scaling qubit systems. One prominent trend is the exploration of hybrid quantum-classical approaches. These allow researchers to integrate the strengths of both paradigms, thus flicking the switch toward practical applications without waiting for full quantum supremacy.
Moreover, the emergence of quantum hardware startups, such as Rigetti Computing and IonQ, illustrates a healthy environment for competition and innovation. These companies vie to develop qubit technologies that are both robust and scalable. There's a growing interest in building quantum networks—think of them as the internet for quantum information. The idea is to enable quantum entanglement over long distances, providing a whole new avenue for applications in secure communication and distributed computation.
A further trend includes the development of accessible quantum software platforms, like IBM’s Qiskit, allowing more individuals to experiment with quantum algorithms. These programs lower the barrier of entry and foster a budding community of quantum developers and enthusiasts.
Potential Impact on Society
The societal impacts of quantum computing are poised to be groundbreaking. With its unmatched computational prowess, quantum technology can potentially solve problems currently deemed intractable. Fields such as pharmaceuticals could witness a revolution; simulating molecular interactions to discover new drugs much faster than traditional methods. Similarly, industries dependent on complex optimization—like logistics and finance—might harness quantum algorithms to streamline processes that take classical computers eons to compute.
Healthcare is another critical domain. Quantum imaging techniques could push the boundaries of diagnostics, enabling earlier detection of diseases and tailored treatment options. At its core, the efficiency gains from quantum computers could translate into savings and better resource allocation across many sectors, providing a considerable uplift in productivity.
However, this impact isn't just silver linings; it beckons a cause for caution. The very capabilities that quantum computers lend to industries can also be a double-edged sword. For instance, vulnerabilities in current cryptographic systems could be exposed, prompting a swift need for upgraded security protocols.
Ethical Considerations
As quantum computing progresses, ethical considerations come to the forefront. With great power comes great responsibility, and understanding the consequences of deploying such transformative technology becomes crucial. One area of concern lies in data privacy and security. Quantum computers could potentially render traditional encryption methods ineffective, raising questions about data protection and surveillance.
Additionally, there’s a substantial risk of exacerbating societal inequalities. The race for quantum supremacy could see wealthier nations and corporations monopolize the technology, leaving less developed regions behind in the technological curve. Addressing this inequity requires proactive measures and international cooperation.
Moreover, ethical implications extend to AI enhancement through quantum computing. As quantum systems process vast datasets more efficiently, the potential for bias in decision-making could intensify, necessitating vigilant oversight on algorithmic fairness.
"The real challenge is not just about building better quantum computers, but ensuring that their benefits are distributed equitably across society."
Ending
In the grand tapestry of technological advancement, quantum computing holds a unique thread, woven tightly with promises and challenges alike. This article sought to shed light on the intricate workings and significance of quantum computer systems, exploring the multifaceted layers that define this revolutionary technology. Understanding these systems is not just an academic exercise; it's critical for students, researchers, and professionals who aim to leverage quantum computing's vast potential in various fields.
Recapitulation of Insights
As we reflect on the earlier sections, several key insights stand out. The concept of qubits, the very basic units of quantum information, is pivotal. Unlike classical bits, qubits can exist in multiple states at once due to superposition, allowing quantum computers to process a vast amount of information simultaneously. The phenomenon of quantum entanglement further complicates matters, enabling qubits that are entangled to be correlated in ways that classical bits cannot achieve.
The various architectures discussed, such as gate-based systems, quantum annealing, and topological quantum computing, illustrate the different pathways researchers are taking to build functional quantum systems. Each architecture comes with its unique strengths and challenges—ranging from scalability to error rates—highlighting that the field is still very much a work in progress.
Moreover, the applications of quantum computing are both diverse and impactful. Its potential in reshaping cryptography, solving complex optimization problems, and conducting advanced quantum simulations could lead to breakthroughs that traditional computing methods might struggle to achieve. However, the hurdles we face today, particularly in terms of hardware limitations and maintaining coherence, remind us that this journey is fraught with challenges.
Final Thoughts on Quantum Computing
Looking ahead, the future of quantum computing is not merely about the technology itself but also about its broader implications for society. As research continues to evolve, trends indicate that quantum computing might revolutionize industries—a change that could fundamentally alter how we think about privacy, security, and scientific inquiry. Ethical considerations, particularly around these changes, cannot be overlooked; we must consider how society will adapt to a landscape increasingly influenced by quantum advancements.
The promise of quantum computing beckons us forward, urging stakeholders across fields to engage in discussions that ping pong between optimism and caution. With each breakthrough, there is a call for responsibility, ensuring that the benefits extend to all and that the challenges are met with thoughtful solutions.
"Quantum computing isn’t just about better algorithms; it’s about rethinking the very nature of computation itself."
In summary, to fully harness quantum computing's potentials, an interdisciplinary approach—integrating insights from computer science, ethics, and social sciences—is essential. The journey is complex, yes, but the rewards could be monumental.