Exploring the Complexities of Plastic Composition
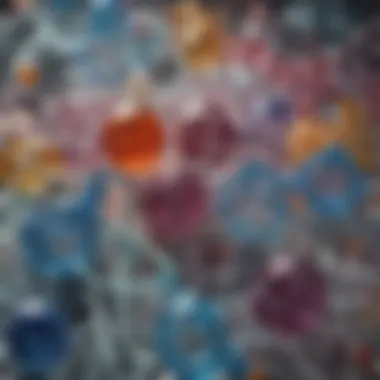
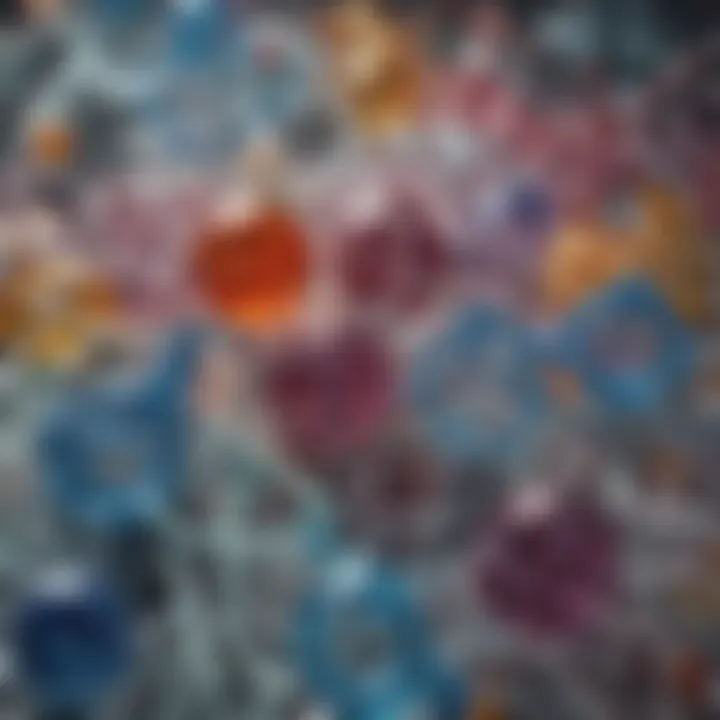
Overview of Research Topic
Brief Background and Context
Plastics permeate nearly every facet of our lives, stemming from their invention in the early 20th century and their subsequent widespread adoption across various industries. From packaging materials that keep our food fresh to the components in modern electronics, understanding the composition of plastics is essential to comprehend their impact on our environment and society. There are myriad types of plastics, each with distinct chemical structures and properties. For instance, polyethylene, often found in shopping bags, is radically different from polystyrene, used in foam containers. This article is a dive into those differences, illuminating the aforementioned aspects of plastics.
Importance in Current Scientific Landscape
In light of increasing concerns regarding environmental sustainability, the study of plastics becomes not only relevant but crucial. With millions of tons of plastic waste entering our oceans each year, a significant question emerges: How can we harness material science to address this growing crisis? Additionally, as researchers develop more biodegradable options and recycling methodologies, there is a harmonious blend of innovation and responsibility that must be grasped. The intricate chemistry of plastics cannot be separated from the larger conversation surrounding pollution, resource management, and sustainable practices. After all, the choices we make today regarding plastic usage will resonate for generations to come.
Methodology
Research Design and Approach
This article employs a comprehensive analysis approach, drawing upon peer-reviewed articles, industry reports, and recent technological advancements pertaining to plastic materials. By triangulating information from various reputable sources, a coherent narrative emerges that encapsulates the multifaceted nature of plastic composition. This means not just discussing the types of plastics but also contextualizing their creation, utility, and eventual disposal.
Data Collection Techniques
Data is collected through a mix of qualitative and quantitative methodologies. Qualitative data pertains to the environmental impact assessments of different types of plastics, whereas quantitative data arises from industry production statistics and market analyses. To further ground this research, case studies on companies innovating in the sustainable plastic space have been included, providing concrete examples of how theoretical concepts translate into real-world applications.
"Understanding the composition of plastics is key to addressing their environmental challenges."
In concluding this overview, the significance of studying plastic composition cannot be overstated. It sets the stage not just for understanding the materials we engage with daily but also for proactively seeking sustainable alternatives as global citizens.
Prolusion to Plastic Composition
The topic of plastic composition occupies a pivotal role in our understanding of materials today. As we weave through the intricate fabric of modern society, we find ourselves surrounded by plastic in various forms — from everyday items in our homes to advanced applications in technology and medicine. This article aims to dissect the topic thoroughly, illustrating its relevance not just in industry but also in environmental dialogues.
Plastics, with their versatile properties, have transformed the commercial landscape in remarkable ways. The composition determines their physical characteristics like strength, flexibility, and chemical resistance. Knowing these components opens doors to innovative solutions in recycling, developing sustainable alternatives, and enhancing functionality in diverse applications.
Historical Context of Plastic Development
Plastic has a rich history, emerging initially in the mid-19th century. In 1856, Alexander Parkes introduced Parkesine, the first synthetic plastic, at the Great International Exhibition in London. Although it wasn’t commercially successful, it laid the groundwork for further advancements. Fast forward to the 20th century, and polymers were being mass-produced. DuPont, for instance, created nylon in the 1930s — a milestone that revolutionized textiles.
As decades rolled by, more variations sprouted up, such as polyethylene in 1933 and polystyrene shortly thereafter. The introduction of these materials reflected not just technological prowess but also an understanding of the utility and adaptability of plastics. However, while these developments marked industrial progress, they also set the stage for environmental ramifications that we grapple with today.
"The development of plastic was a triumph of science, but as we now know, it also posed a challenge to sustainability."
Definitions and Key Concepts
To fully grasp the realm of plastic composition, it is crucial to clarify some key definitions. At its core, plastic is derived from polymers — long chains of molecules formed through processes called polymerization.
Polymers can be found in two primary forms — natural and synthetic. Natural polymers include substances like rubber and cellulose, while synthetic variants range from polyester to polycarbonate. Understanding the distinction between thermoplastics and thermosetting plastics is also significant. Thermoplastics can be melted and remolded, which makes them suitable for recycling, whereas thermosetting plastics undergo a hardening process, making them rigid and less adaptable.
Additionally, recognizing the role of monomers is essential. Monomers are the building blocks that combine to form polymers. In the realm of plastic, variations in the chemical structure of monomers can lead to significant differences in properties, dictating how and where a plastic can be used.
Finally, additives add another layer of complexity. These substances can enhance or modify plastics in myriad ways, spanning from colorants to plasticizers that make materials more flexible. They play a vital role in defining the environmental impact of plastic as well – a varying consideration in our increasingly eco-conscious society.
Types of Plastics
Understanding the various types of plastics is crucial for grasping the complexities of their applications and impacts. Different plastics have distinct properties, which enable their use across a wide array of industries. Moreover, the classification influences decisions made in design, manufacturing, and recycling processes, aiding in developing sustainable practices.
When discussing types of plastics, we primarily encounter thermoplastics, thermosetting plastics, and elastomers. Each of these categories comes with unique benefits and considerations that inform their operational uses. Learning about them not only helps students and professionals alike understand material properties but also plays a vital role in enhancing environmental considerations tied to plastic production and waste management.
Thermoplastics
Thermoplastics are one of the most versatile plastics available today. By nature, these materials can be repeatedly melted and reshaped, which contrasts with other plastic types. This quality opens numerous doors in terms of manufacturing, making it the go-to choice for many industries. From food packaging to automotive parts, thermoplastics like polyethylene, polypropylene, and PVC are prevalent.
- Durability and Flexibility: Due to their molecular structure, thermoplastics remain tough and flexible under varied temperatures and conditions.
- Ease of Processing: They allow for efficient production techniques which can be key drivers in cost-effective manufacturing.
- Recyclability: Unlike some plastics, thermoplastics can be recycled, which is essential given the growing emphasis on sustainability. This recycling capability is a double-edged sword, however, as improper recycling processes can lead to operational inefficiencies.
"Thermoplastics represent an integral part of sustainable practices in modern manufacturing, offering solutions that adapt to changing demands."
Thermosetting Plastics
Thermosetting plastics, or thermosets, differ significantly from thermoplastics due to their irreversible curing process. Once set, they cannot be remelted or reshaped, which leads to a greater strength and resistance against heat and solvents. Examples include epoxy resins, phenolics, and polyurethanes.
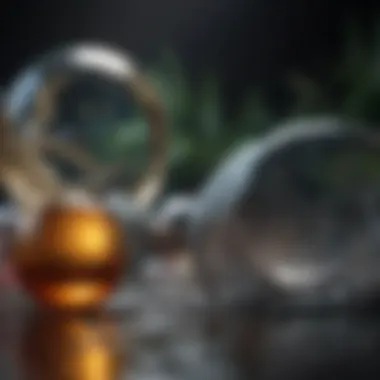
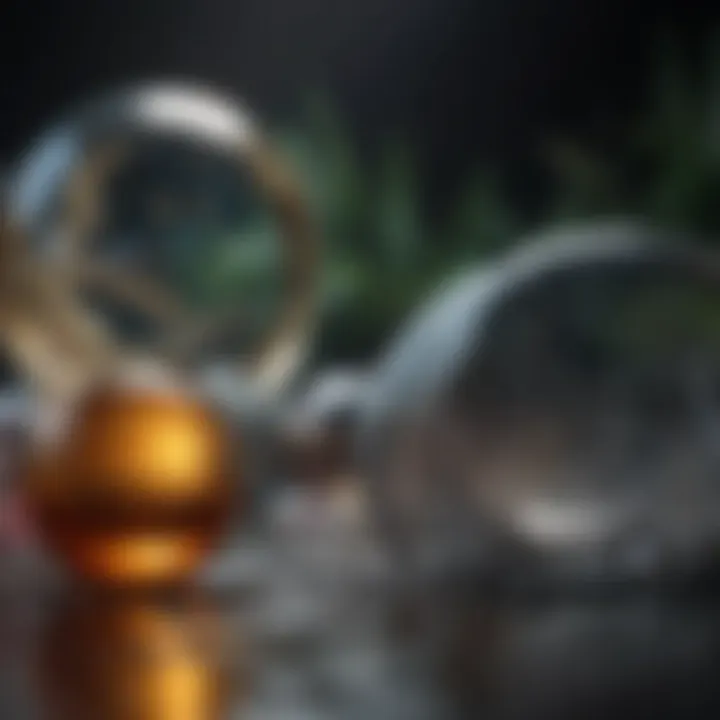
- High Strength and Durability: Their robust structure makes them excellent for high-stress applications, such as in aerospace or electronics.
- Thermal Resistance: Thermosets can withstand significant temperature fluctuations, rendering them ideal for applications requiring thermal stability.
- Limited Recyclability: On the downside, if the aim is to repurpose or recycle thermosetting plastics, it often isn't feasible since they do not remelt. This factor can create challenges concerning waste management and disposal.
Elastomers
Elastomers combine the properties of rubber and plastics. These materials exhibit remarkable elasticity and resilience under stress, which makes them particularly useful in applications where shape recovery is essential. Common examples include natural rubber and synthetic options like silicone and polyurethane elastomers.
- Elasticity and Flexibility: Their ability to stretch and bounce back makes elastomers perfect for applications such as seals, gaskets, and tires.
- Vibration Damping: They provide excellent resistance to vibrations and shocks, making them valuable in automotive and construction sectors.
- Temperature Sensitivity: While generally resilient, some elastomers can degrade under extreme temperature conditions, influencing their lifespan.
Chemical Composition of Plastics
The realm of plastic materials is underpinned by their chemical composition, which serves as the bedrock for understanding their behavior, uses, and impacts on our environment. Understanding this composition is not just about looking at raw ingredients; it’s about comprehending the intricate relationships between these elements and how they coalesce into the versatile materials we rely on daily. This section will break down the foundational components of plastics, emphasizing the unique aspects that shape their properties and applications.
Polymer Structure and Functionality
Plastics primarily consist of polymers—large molecular chains formed by linking repeated units known as monomers. The specific arrangement of these monomer units within the polymer chain determines the physical characteristics of the plastic, such as flexibility, strength, and resistance to chemicals.
For instance, polyethylene, one of the most common plastics, has a linear structure that provides great tensile strength, while polystyrene's branched polymers are more rigid but brittle. Such structural differences can significantly affect functionality—what works perfectly for a food container might not be suitable for durable machinery parts. Understanding polymer structure is crucial because it guides the choice of materials based on the intended application.
"The unique attributes of polymers hinge on their structures, providing insights into their transformative capabilities in various sectors of industry."
Monomers and Polymerization Processes
At the heart of plastic production is the process of polymerization, the chemical reaction that creates polymers from monomers. This process can occur in several ways, but two primary types dominate: addition polymerization and condensation polymerization.
- Addition Polymerization: This occurs when monomers add together without the loss of any small molecules. Most common plastics, like polyethylene and polyvinyl chloride (PVC), use this method. The rapid formation of long chains results in materials that can be tailored for a wide range of applications.
- Condensation Polymerization: This process involves the joining of monomers, with a byproduct like water being released. Common examples include polyesters and certain nylons, which are known for their durability and versatility.
Understanding these processes gives insight into how we can innovate and modify plastic types to meet new challenges, especially as the need for sustainability grows.
Additives and Their Roles
While the base polymer provides the primary characteristics of plastic, additives are often blended in to enhance properties or introduce new functionalities. These additives can make a world of difference, allowing plastics to perform under various conditions or act specific ways.
Some common categories of additives include:
- Plasticizers: This makes plastics more flexible, crucial for materials like vinyl flooring and inflatable products.
- Stabilizers: These prevent degradation from UV light or heat, extending the lifetime of outdoor products such as garden furniture.
- Fillers: Substances like talc or calcium carbonate reduce costs and may also affect strength and weight.
The careful selection and application of these additives are essential for the performance, safety, and sustainability of plastic products. Each tweak at this level can potentially lead to substantial changes in the material's life cycle and its eventual impact on the environment.
Natural vs. Synthetic Plastics
The distinction between natural and synthetic plastics is of paramount importance in understanding the broader implications of plastic usage in modern society. By examining both categories, one uncovers not only the materials' composition but also their environmental impact, sustainability, availability, and the trends driving their development.
Natural plastics, derived from organic materials such as plants and animals, often boast biodegradable properties. A prime example of this includes materials like cellulose, which is found in the cell walls of plants. These biodegradable plastics are noteworthy as they can decompose naturally, given the right environmental conditions. This capability reduces their ecological footprint, making them increasingly relevant in the discussion on sustainable materials and their potential in mitigating plastic pollution.
On the flip side, synthetic plastics are created through extensive chemical processes, typically from fossil fuels. Common examples include polyethylene, polyvinyl chloride, and polystyrene. While they offer remarkable durability and a wide variety of applications, synthetic plastics pose significant environmental challenges. Their decomposition can take hundreds, if not thousands, of years, leading to large accumulations of waste. Furthermore, the chemical processes involved in synthetic production often contribute to carbon emissions and other environmental hazards.
Understanding the implications of both natural and synthetic plastics is vital in guiding policies and practices related to plastic manufacturing, usage, and disposal. By assessing the benefits and drawbacks of each type, stakeholders can make informed decisions that promote sustainability and environmental stewardship.
"In weighing the balance between natural and synthetic plastics, we must consider not only the immediate utility but also long-term consequences entailed by their lifecycle."
Biodegradable Plastics
Biodegradable plastics, a subset of natural plastics, have surged to the forefront of discussions on sustainable materials. These plastics break down more swiftly than their traditional counterparts, resulting in less environmental strain. They are specially designed to decompose through the action of living organisms, thus transforming into compost or biomass. Examples include polylactic acid (PLA), which is derived from corn starch, and polyhydroxyalkanoates (PHAs), produced by microbial fermentation of sugar or lipids.
Key Benefits of Biodegradable Plastics:
- Reduced Waste: They minimize trash accumulation in landfills.
- Less Environmental Impact: Their decomposition process typically releases fewer harmful emissions compared to traditional plastics.
- Sustainable Resources: Many are sourced from renewable materials, decreasing reliance on fossil fuels.
However, challenges remain. The industrial composting infrastructure necessary for their proper disposal is still limited in many areas, which can hinder their effectiveness. Proper public education on biodegradable options is essential so consumers understand that these plastics don’t just break down anywhere; they need the right conditions to be effective.
Traditional Plastics: Environmental Implications
Traditional plastics, while incredibly versatile, present several environmental challenges. Their durability, long viewed as an advantage, becomes a double-edged sword when considering waste management and ecological preservation. Items made from materials like polyethylene can take centuries to decompose, leading to notable pollution.
Environmental Considerations:
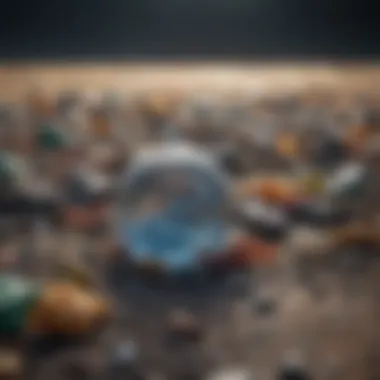
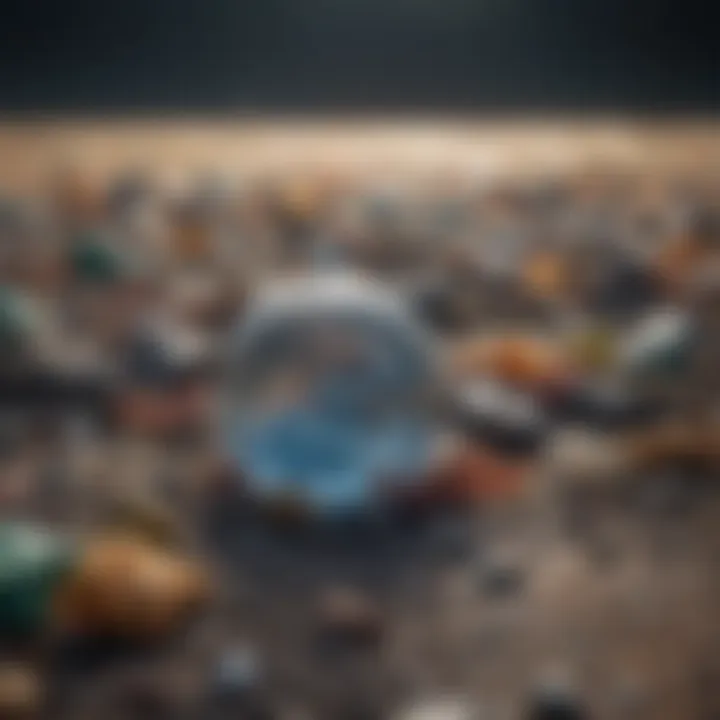
- Ocean Pollution: Millions of tons of plastic end up in oceans every year, harming marine life and polluting ecosystems.
- Carbon Footprint: The extraction and processing of fossil fuels for plastic production result in significant greenhouse gas emissions.
- Microplastics: Traditional plastics often break down into microplastics, which infiltrate food chains and threaten human health.
The pressing challenge is to innovate better ways to manage traditional plastic production and to explore alternatives that could lessen environmental burdens. This continuous exploration will yield insights guiding the way forward for future plastic applications.
Applications of Plastics in Various Industries
Plastics find their way into nearly every corner of modern life. This section sheds light on their remarkable versatility across diverse industries. Beyond being a material with utility, plastics often offer efficiency, durability, and adaptability that other materials might struggle to match. In addition, understanding these applications enables one to appreciate how ingrained plastics have become in our daily endeavors and industrial processes.
Medical Applications
In the medical field, the significance of plastics is hard to overlook. From surgical instruments to packaging of medications, plastics are integral in ensuring safety and hygiene. Consider, for instance, the use of polypropylene in syringes. This plastic is not only lightweight but also resistant to chemicals, making it ideal for disposable uses.
- Biocompatibility: Many plastics are designed to be biocompatible, which means they can safely coexist with living tissue. This is critical in items such as catheter tubes and implants, where performance without adverse reactions is essential.
- Sterilization: Plastics allow for effective sterilization, a vital feature in hospitals. They're easy to clean and can withstand various sterilization methods, such as ethylene oxide gas or steam vacuum.
Moreover, the invention of medical-grade plastics continues to revolutionize treatments and procedures. Particularly striking is the recent increase in the use of 3D printing techniques to create customized medical devices tailored to individual patients. The convergence of technology and material science holds promise for even more advanced medical applications in the future.
Automotive Industry Innovations
The automotive industry has seen remarkable transformations through the use of plastics. Not merely for aesthetic embellishments, plastics are utilized in vital structural components of vehicles. This is a significant boon for safety, fuel economy, and overall performance.
- Lightweight Construction: Reducing vehicle weight is crucial for fuel efficiency. Manufacturers have turned to plastics like acrylonitrile butadiene styrene (ABS) to replace heavier metals.
- Enhanced Safety Features: Bumpers made of high-density polyethylene absorb more impact, offering better protection during collisions.
- Design Flexibility: Plastics enable manufacturers to create complex shapes that would be difficult or impossible to achieve with traditional materials.
The consistent drive toward innovations has led to the development of advanced composites, which merge plastics with fibers to enhance strength without sacrificing weight. The future of automotive design is likely to lean even more on these advanced materials for better energy efficiency and reduction in emissions.
Plastic in Packaging: Benefits and Challenges
Plastics have shifted paradigms in packaging, making them ubiquitous in consumer goods. Their properties allow for lightweight, durable, and moisture-resistant packaging that ensures products remain fresh and protected.
- Benefits:
- Cost-Effective: The lightweight nature leads to lower transportation costs.
- Versatility: Packaging can be customized to a variety of shapes and sizes, accommodating an extensive range of products.
- Barrier Properties: Plastics can be engineered to provide barriers against oxygen, moisture, and light, extending the shelf life of food products.
Nevertheless, the usage of plastics in packaging comes with its own set of challenges, particularly around environmental sustainability. Most conventional plastics are non-biodegradable, and even though recycling efforts have been introduced, they often fall short. Finding solutions to these environmental issues without compromising the practicality of plastics is a significant challenge facing the industry today.
"The evolution of plastics in packaging reflects the delicate balance between utility and ecological responsibility."
As industries continue to innovate, understanding the applications of plastics not only informs us about their role in daily life but also emphasizes the ongoing dialogue around sustainability and responsible use.
Environmental Considerations and Sustainability
The significance of environmental considerations and sustainability in regard to plastics cannot be overstated. Plastics have integrated themselves deeply into modern society, contributing to countless conveniences, yet they also come with an array of environmental concerns. As we delve into the impact plastics have on our ecosystems and the potential solutions, it's vital to evaluate both sides—recognizing the advantages of plastics while steadfastly addressing their adverse effects.
Impact of Plastic Waste on Ecosystems
Plastic waste poses a serious threat to ecosystems around the globe. It is not just an eyesore, but a perilous invader of wildlife habitats. Marine environments are particularly vulnerable. Animals often mistake plastic debris for food, leading to dire consequences (e.g., choke or starvation). It's important to note that plastics do not biodegrade like organic materials. Instead, they break down into tiny particles known as microplastics, which can infiltrate even the most remote environments, wreaking havoc on flora and fauna alike.
"Plastic does more than just congest our oceans; it disrupts the delicate balance of life within those waters."
Among the species affected, seabirds, turtles, and fish stand out as critical indicators of plastic pollution's impact. Studies suggest that over 90% of seabirds have ingested plastic at some point in their lives. This is not just an ecological issue; it poses risks to human health as well, as toxins from plastics can enter food chains.
To combat plastic pollution, ecosystem management and restoration efforts must be prioritized. Several organizations and local governments are ramping up campaigns to clean our oceans and educate the public. Yet, the path to recovery demands collaboration and long-term commitments from all sectors of society.
Recycling Processes and Challenges
Recycling plastics is regarded as one of the most viable ways to mitigate waste. However, the current systems have their own set of challenges. Each type of plastic requires specific methods for recycling, leading to complications in sorting and processing. In many facilities, plastic waste often ends up in landfills due to contamination or inadequate separation technologies.
The recycling process generally involves the following stages:
- Collection: Gathering used plastic materials from consumers and businesses.
- Sorting: Identifying and categorizing plastic types—this is where contamination often complicates the process.
- Cleaning: Rinsing off any residues or contaminants.
- Shredding: Breaking down the plastic into smaller pieces.
- Reprocessing: Melting and remolding the plastic into new products.
Despite these efforts, many recycled plastics do not achieve the quality necessary for high-end uses. Additionally, the economic viability of recycling varies greatly depending on market demands and technological advancements.
Advancing recycling technologies and embracing a circular economy model is key to improving these processes. Circularity means designing products that can be easily recycled or repurposed, thus minimizing waste and environmental strain. Stakeholders across the board—from manufacturers to consumers—must work together to establish efficient recycling systems, which underscore sustainability in the plastic production sphere.
In understanding the implications of plastic composition, we must not shy away from examining how these materials interact with our environments. The balance between utilization and sustainability remains a pressing issue that requires immediate attention and informed action.
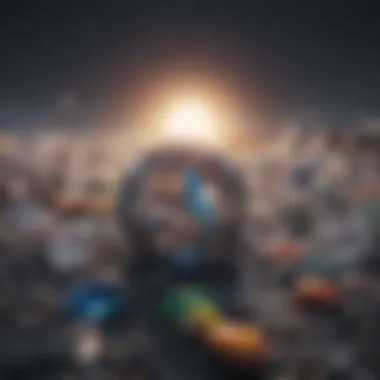
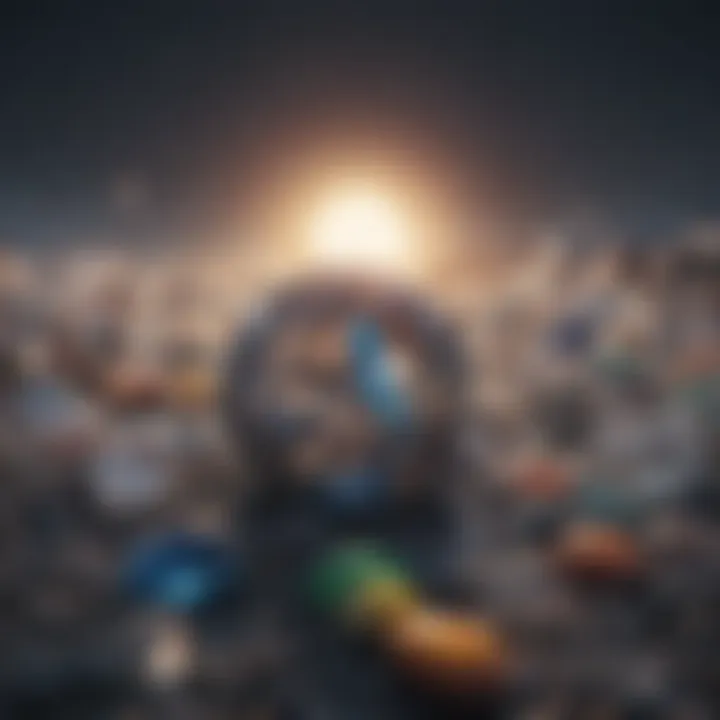
Innovations in Plastic Technology
The realm of plastic technology is dynamically evolving, driven by the urgent need for sustainable solutions. As societies grapple with the consequences of plastic waste, innovations in this sector are not just welcome but essential. This section seeks to illuminate the strides being made in plastic technologies that promise both ecological and practical benefits.
One significant area of focus is the development of advanced recycling methods. It’s no secret that traditional recycling processes have their limitations, often leading to a situation where a large percentage of plastics go unrecycled or are downcycled into lower-quality materials. Recent advancements aim to improve the efficiency of recycling by incorporating new technologies. For instance, chemical recycling methods have gained traction. Unlike mechanical recycling, which can compromise the integrity of the polymer, chemical recycling breaks down plastics into their original monomers, allowing for the production of virgin-quality plastics. This method can potentially keep plastics in circulation longer, reducing the need for virgin resin and subsequently lowering the carbon footprint associated with plastic production.
Moreover, these advancements are increasingly becoming accessible. More facilities are adopting these high-tech processes, leading to broader adoption and ultimately creating a more robust recycling infrastructure. With consumers growing aware of their choices, the demand for high-recycled-content materials continues to rise, and so does the motivation for brands to invest in these technologies.
Advancements in Recycling Methods
In recent years, recycling methods have transformed significantly, responding to the complex challenge of managing plastic waste:
- Chemical Recycling: This method decomposes plastics back into their original raw materials. This allows the creation of higher quality, usable products. For instance, organizations are now using solvents to dissolve plastics efficiently, thus promoting a circular economy.
- Biological Recycling: Emerging approaches utilize microbes to digest plastics, breaking them down into less harmful substances or even into usable products. Although still in initial stages, these innovations show promise for reducing waste.
- Advanced Sorting Technologies: Systems that employ artificial intelligence and machine learning are becoming game changers in sorting plastic waste. These technologies enhance the accuracy of separating different types of plastics, contributing to better recycling outcomes.
It’s essential to note that these advancements don't come without challenges. Evolving legislation needs to keep pace with technological innovations. Moreover, there are substantial costs involved in modernizing existing recycling facilities to accommodate new methods.
Bioplastics: The Future of Sustainability
Bioplastics have emerged as a beacon of hope in the quest for sustainable materials. Composed from renewable sources like corn starch, sugarcane, or even food waste, they pose an attractive alternative to conventional petroleum-based plastics. Some notable aspects of bioplastics include:
- Reduced Carbon Footprint: Since they're derived from renewable resources, production often results in lower greenhouse gas emissions.
- Potential for Compostability: Certain bioplastics can break down in composting environments, reducing the need for landfill disposal.
- Versatility: Bioplastics can be engineered to mimic the properties of traditional plastics, making them suitable for a vast range of applications, from packaging to automotive parts.
Nevertheless, not all bioplastics are created equal, and misconceptions abound. Some varieties require specific conditions to degrade, which may not align with typical waste management systems. It’s vital for industries, consumers, and policymakers alike to educate themselves on the capabilities and limitations of bioplastics.
"The future increasingly seems inclined to favor materials that are both functional and sustainable, where bioplastics could play a significant role in reducing our reliance on petroleum-based materials."
The evolution of plastic technology is marked by these promising innovations. As these advancements solidify and expand, the path toward a more sustainable future becomes clearer. Reconciling the efficiency of plastic with environmental responsibility hinges on continued research, innovation, and most importantly, a commitment by all stakeholders to embrace these transformative changes.
Future Directions for Plastic Research
Plastic composition and technology are rapidly evolving fields facing both challenges and opportunities. Research into innovative materials and technologies may hold the key to numerous pressing issues, from reducing waste to discovering more sustainable options. As the world grapples with the ongoing plastic crisis, understanding future directions for plastic research is paramount. It not only lays the groundwork for better practices but also equips industries and consumers alike to make informed choices.
Emerging Materials and Technologies
One promising area in plastic research is the exploration of alternative materials, which seeks to reduce reliance on conventional fossil-fuel-based plastics. Interesting developments are ongoing:
- Bio-based plastics are gaining traction, which are derived from renewable sources such as corn or sugarcane. These materials often boast biodegradability or recyclability, addressing some environmental concerns associated with traditional plastics.
- Smart plastics are in the pipeline, meant to have adaptive functionalities. For example, these materials may alter their properties in response to environmental triggers, enhancing their usability in various settings.
- Nanotechnology plays a pivotal role in enhancing plastic properties. By utilizing nanoscale additives, plastics can be made stronger, lighter, and more resistant to degradation over time.
These advancements suggest that the focus on developing materials can lead to solutions that not only perform comparably to, but potentially outperform, current plastic options. This can shift perceptions in both consumer markets and industry spaces, driving changes towards more responsible usage of materials.
"Innovation in material science is not just about creating alternatives; it’s about redefining our relationship with consumption and waste."
The Role of Policy in Plastic Usage
Policy plays a crucial part in steering plastic research and usage, especially as recent international dialogues focus on achieving sustainability. Here are a few key considerations:
- Regulatory Frameworks: Governments are beginning to impose regulations that could influence how plastics are produced, used, and disposed of. This includes incentives for adopting greener alternatives and penalties for excessive plastic waste generation.
- Public Awareness Campaigns: Effective policies often go hand-in-hand with public education. By informing citizens about responsible plastic use, recycling methods, and environmental impacts, policymakers can motivate behavioral shifts.
- Funding for Research: Investment in research initiatives, often spearheaded by governmental bodies, can catapult innovative ideas into real-world applications. Increased funding can expedite the development of biodegradable plastics and recycling technologies, creating more sustainable options.
- International Collaboration: Engaging in cross-border cooperative efforts can amplify the impact of plastic research. Global challenges require international solutions, making partnerships essential.
Understanding the interplay between policy and technological innovation equips stakeholders with the knowledge to advocate for change. As new materials emerge, they must be supported by robust policies that foster responsible consumption and steer industries toward sustainability.
Ending
As we draw the curtain on our exploration of plastic composition, we find ourselves anchored in a sea of insights that underscore both the complexities and the significance of this material. Understanding plastic composition is not merely an academic exercise; it is a vital consideration for innovation, environmental stewardship, and technological advancement. The composition of plastics, from their polymer structures to the additives that enhance their properties, plays a critical role in determining their functionality and sustainability.
One of the pivotal insights gleaned from our discussion is the distinction between natural and synthetic plastics, which brings forth a layered understanding of their environmental implications. The increasing reliance on synthetic plastics raises questions around their biodegradability and the long-term impact on ecosystems. Meanwhile, advancements in biodegradable alternatives shine a light on innovative solutions that blend utility with responsibility.
Moreover, the various applications of plastics across different industries—from medical uses to packaging—highlight their indispensable role in modern society. However, the issues surrounding plastic waste management and recycling challenges remind us of the pressing need for sustainable practices.
"The road ahead requires us to rethink our relationship with plastics, urging scientists, consumers, and policymakers alike to engage in proactive dialogue and action."
In sum, the conclusion serves as a clarion call, urging us to not just understand but to also engage with the implications of plastic in our lives. By emphasizing the importance of material science in crafting sustainable solutions, we can contribute to a healthier relationship with the materials we use every day.
Summarizing Key Insights
In synthesizing the key insights from our exploration into plastic composition, several points emerge as fundamental:
- Diverse Types of Plastics: Understanding the different categories—thermoplastics, thermosetting plastics, and elastomers—reveals their distinct properties and applications.
- Chemical Composition: Recognizing how polymers are structured and the role of monomers in their formation enriches our grasp of their functionalities.
- Environmental Considerations: The interplay between synthetic and biodegradable plastics has critical implications for ecosystem health and waste management strategies.
- Innovative Applications: The variety of applications underscores the versatility of plastics and the pressures for innovation to mitigate their environmental footprint.
- Future Directions: Ongoing research into emerging materials and supportive policies will shape the landscape of plastic use moving forward.
Call to Action for Further Research
With the landscape of plastic composition laying out complex challenges and significant opportunities, it is essential to champion further research in this domain. Here are several avenues ripe for investigation:
- Innovative Recycling Technologies: Developing novel methods to recycle plastics more effectively can drastically reduce waste and enhance sustainability.
- Lifecycle Assessments: More comprehensive studies assessing the full impact of plastics from production to disposal are critical in understanding their environmental footprint.
- Development of Bioplastics: Studying the potential of bioplastics offers a forward-thinking approach to reducing reliance on traditional polymers, paving the way for more sustainable materials.
- Policy Integration: Research on the effect of legislation and consumer behavior on plastic usage will drive informed policy-making.