Exploring the Dynamics of ORF Cloning in Modern Science
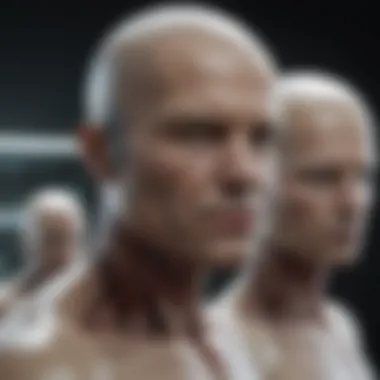
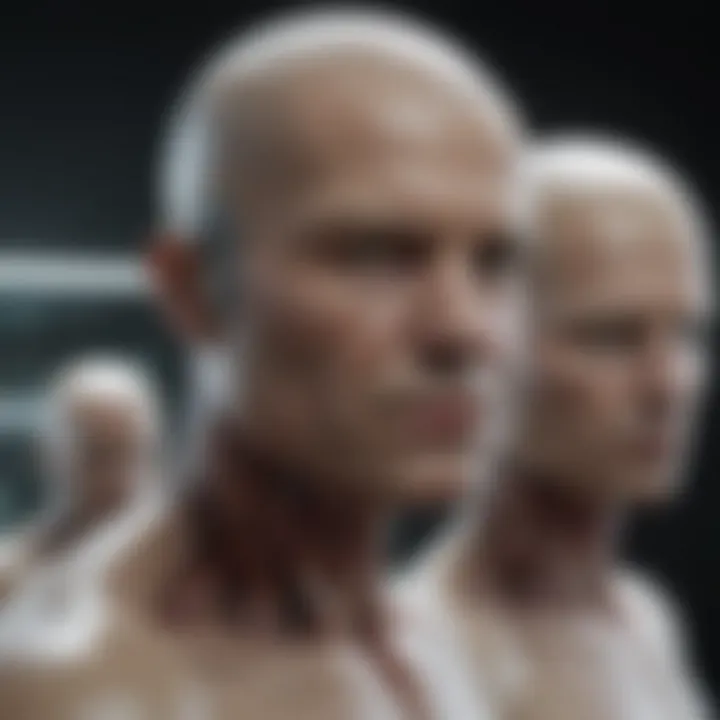
Intro
Open Reading Frame (ORF) cloning stands as a cornerstone in the realm of molecular biology. This technique is integral for studying gene function and understanding protein expression. It plays a significant role in unraveling the complexities of biological systems, allowing researchers to explore the narratives written in our DNA.
As the need for precise genetic manipulation grows, the influence of ORF cloning continues to expand. By enabling the amplification of specific gene sequences, scientists can investigate the underlying mechanisms of various functions in cells. The advancements have opened new dimensions in biotechnology and synthetic biology, thereby enhancing the development of therapeutic solutions.
Overview of Research Topic
Brief Background and Context
ORF cloning originated from the broader field of molecular cloning, which provides techniques for manipulating DNA. The fundamental concept involves isolating a segment of DNA that encodes a protein, providing researchers a way to produce proteins of interest in a host organism. With advancements in technology, the methods for ORF cloning have evolved, allowing for more streamlined processes.
In essence, an Open Reading Frame is a continuous stretch of nucleotides that encodes a protein. Identifying and amplifying these frames is crucial for functional studies. Techniques such as PCR (Polymerase Chain Reaction) and restriction enzyme digestion facilitate the cloning process.
Importance in Current Scientific Landscape
ORF cloning has paved the way for essential breakthroughs in various disciplines, including genetics, biochemistry, and medicine. Its applications are manifold:
- Biotechnology: Developing genetically modified organisms that produce valuable products, such as insulin or enzymes.
- Therapeutics: Creating targeted treatments based on specific proteins involved in disease processes.
- Synthetic Biology: Allowing for the engineering of new biological parts, devices, and systems.
Researchers rely on ORF cloning to provide insights that can lead to innovations in health, agriculture, and environmental sustainability.
"The ability to clone open reading frames has significantly enhanced our understanding of genetic functions, catalyzing advancements in numerous scientific fields."
By situating ORF cloning within the broader context of scientific inquiry, its relevance becomes even clearer. As we delve further into the methodologies and applications of ORF cloning, it becomes evident that its impact on modern science cannot be overstated.
Intro to ORF Cloning
Open Reading Frame (ORF) cloning is a foundational process in molecular biology with widespread implications across genetics and biotechnology. The significance of ORF cloning lies in its ability to facilitate the investigation of gene function and the protein products associated with them. This technique allows researchers to create constructs that express specific proteins, illuminating pathways in cellular biology and aiding in the development of therapeutic strategies. Its understanding is crucial for students, researchers, and professionals who engage with contemporary biological challenges.
Definition and Importance
An Open Reading Frame is a sequence of DNA that has the potential to be translated into a protein. In the context of ORF cloning, the term primarily refers to the methodologies that allow scientists to isolate and manipulate these sequences. The importance of ORF cloning extends beyond basic research; it serves various biotechnological and medical applications. By providing a means to express proteins in various systems, this methodology plays a pivotal role in protein production, functional genomics, and even vaccine development. In essence, ORF cloning enhances the understanding of biological systems, making it an invaluable element of modern biological research.
Historical Context
The journey of ORF cloning began in the late 20th century, coinciding with the advancements in DNA sequencing technologies. Initially, molecular cloning relied heavily on conventional plasmid vectors, which laid the groundwork for more complex cloning strategies. As sequencing capabilities evolved, the identification of ORFs in newly sequenced genomes became increasingly accessible. This era marked a fundamental shift in molecular biology, enabling researchers to explore functional genomics more profoundly. The development of sophisticated tools like restriction enzymes and ligation techniques further advanced ORF cloning methods, propelling them into widespread use in laboratories around the world. Over the years, ORF cloning has adapted to integrate new technologies, making it a dynamic field that continues to evolve.
Fundamental Concepts
Understanding the foundational elements of Open Reading Frame (ORF) cloning is essential for the comprehension of the methodologies and applications that follow. These fundamental concepts lay the groundwork for the advanced techniques and intricate challenges inherent in ORF cloning. A clear grasp of these concepts enhances researchers' capabilities to manipulate genetic material for various applications in biotechnology and therapeutic development.
Understanding Open Reading Frames
Open Reading Frames are sequences of DNA that have the potential to be translated into proteins. They are defined by a start codon, usually AUG, and extend until a stop codon is reached. Recognizing ORFs within a given sequence is crucial as it allows scientists to determine which segments of genetic material can encode for functional proteins. The identification of ORFs is significant for several reasons:
- Protein Synthesis: ORFs provide essential information on how proteins are synthesized. Understanding this process highlights the relationship between genes and the proteins they encode, which is fundamental in molecular biology.
- Gene Annotation: Effectively annotating genes relies heavily on the analysis of ORFs. Accurate annotation helps in mapping genetic functions to traits, aiding in fields ranging from plant breeding to pharmaceutical development.
- Mutation Analysis: Evaluating ORFs helps in pinpointing mutations that may lead to dysfunction in protein production. This aspect is vital for understanding disease mechanisms and developing responsive treatments.
In essence, Open Reading Frames are the blueprints of protein coding genes. Their identification is a primary step in any significant genetic analysis.
Gene Structure and Function
The structure of genes is intricately linked to their function, making the study of gene architecture critical in molecular biology. Genes are more than just sequences of nucleotides; they are comprised of various components that contribute to their overall function:
- Exons and Introns: Exons are coding sections of a gene, while introns are non-coding regions. The balance and interaction between these two elements define how a gene is expressed and processed. The splicing process, where introns are removed and exons are joined, is a critical aspect of gene function.
- Promoters and Regulatory Elements: Promoters are sequences that initiate the transcription of a gene. Their interaction with transcription factors regulates gene expression. This regulation is vital for ensuring that genes are expressed at the right time and place, impacting processes such as development and cellular response to environmental signals.
- Post-Transcriptional Modifications: After transcription, genes are often subject to modifications that influence their activity. These modifications can affect mRNA stability, translation efficiency, and ultimately protein functionality.
The multi-layered structure of genes underscores the complexity of genetic regulation and expression. By understanding these elements, researchers can better design experiments and interpret data related to gene functions and their implications in health and disease.
Understanding the nuances of gene structure and the relevance of ORFs is crucial for advancing gene manipulation techniques.
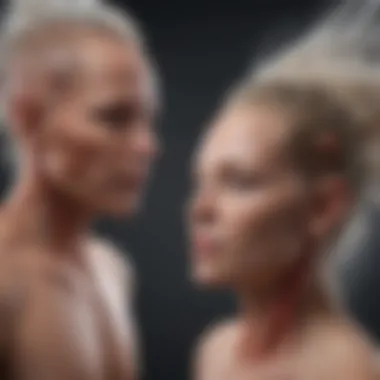
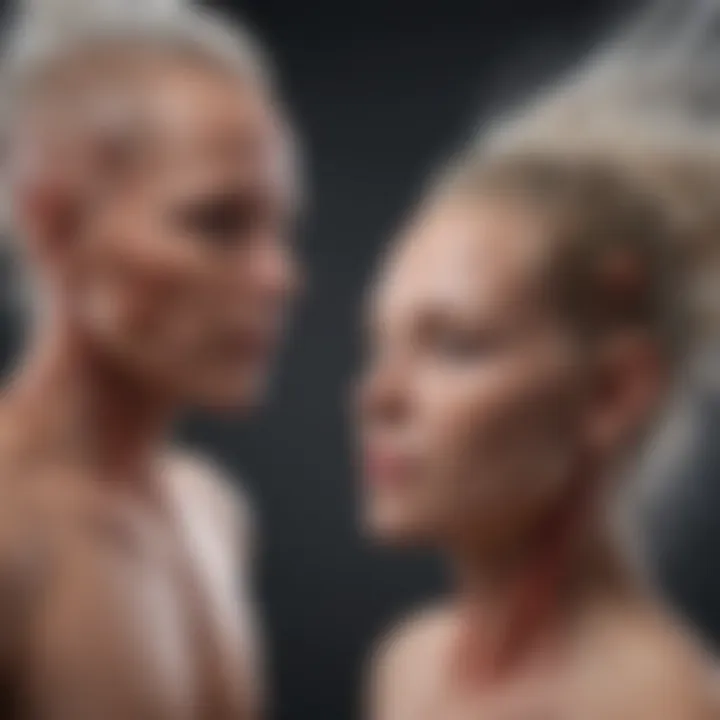
Techniques in ORF Cloning
Understanding the techniques in ORF cloning is fundamental for researchers in molecular biology. These methods allow the efficient manipulation and examination of genes, which aids in understanding their functions and interactions. This section discusses various techniques, emphasizing their individual characteristics and contributions to the broader goals of genetic research and biotechnology. Such knowledge not only enhances experimental design but also impacts the practical applications of ORF cloning in modern science.
Vector Systems
Vector systems are crucial for inserting Open Reading Frames into host cells. Their effectiveness influences the success of cloning experiments and subsequent protein expression.
Plasmid Vectors
Plasmid vectors are circular DNA molecules capable of independent replication within a cell. They serve as a primary tool for gene cloning. Their main characteristic is their ability to easily incorporate small DNA fragments, leading to straightforward transformation procedures. Plasmid vectors are popular due to their ease of use, allowing researchers to manipulate genetic material with relative simplicity. A significant advantage of plasmids is their ability to carry antibiotic resistance genes, enabling the selection of successfully transformed cells. However, they have limitations in accommodating larger inserts, which can restrict their application in some cases.
Viral Vectors
Viral vectors utilize viruses to deliver genetic material into host cells. Their key characteristic lies in their natural ability to infect cells efficiently, which makes them particularly effective for introducing ORFs into mammalian systems. Viral vectors such as lentiviruses and adenoviruses have been extensively employed in gene therapy research. They offer the unique feature of high transduction efficiency and the potential for stable integration into the host genome. Nevertheless, they come with disadvantages, including concerns over immunogenicity and potential safety issues that must be addressed during applications.
Bacterial Artificial Chromosomes (BACs)
Bacterial artificial chromosomes are vectors that can carry large DNA fragments and are derived from the F-plasmid of E. coli. Their main characteristic is the ability to clone large pieces of DNA, up to 300 kb, which is beneficial for mapping genomes and studying large genes. BACs are a popular choice in genomic projects, such as the Human Genome Project, due to their stability and capacity to maintain large inserts. A notable advantage of BACs is their lower copy number, which reduces the likelihood of recombination. However, one disadvantage is the complexity involved in manipulating these larger constructs compared to smaller plasmid vectors.
PCR Amplification
Polymerase Chain Reaction (PCR) plays a pivotal role in amplifying target DNA sequences for cloning. It provides a means to generate sufficient quantities of DNA, ensuring successful cloning outcomes.
Quantitative PCR
Quantitative PCR, or qPCR, is a technique that allows the quantification of DNA. A key characteristic of qPCR is its ability to provide real-time data on the amplification of DNA, offering insights into gene expression levels. This method is beneficial for studying gene regulation and confirming the presence of specific ORFs. The unique feature of qPCR is its high sensitivity and specificity, which allows for the detection of even small amounts of target DNA. The disadvantage can be the requirement for calibration and extensive optimization, which may complicate workflows.
Reverse Transcription PCR
Reverse transcription PCR enables the conversion of RNA into complementary DNA (cDNA) prior to amplification. This technique is essential for studying gene expression from mRNA, providing invaluable data on protein synthesis. A notable characteristic of this method is its capacity to analyze RNA, making it particularly useful in functional genomics. The advantages of reverse transcription PCR include the ability to quantify mRNA levels and gain insights into post-transcriptional regulation. However, it is important to be aware of the disadvantages, such as the potential for contamination and the need for high-quality RNA samples.
Restriction Enzyme Digestion
Restriction enzyme digestion is a critical process for preparing DNA fragments for cloning. These enzymes cut DNA at specific sequences, which allows researchers to create compatible ends on the DNA fragments to be ligated. The importance of this technique cannot be overstated, as it enables precise manipulation of DNA to facilitate cloning activities. The careful selection of enzymes is essential to ensure efficient and successful digestion, as the choice impacts the downstream activities.
Ligation Methods
Ligation is the final step in the cloning process where DNA fragments are joined together. Different ligation methods can influence the efficiency and success of cloning endeavors.
Traditional Ligation
Traditional ligation involves using T4 DNA ligase to join DNA fragments with complementary ends. This method is recognized for its simplicity and effectiveness. The characteristic of traditional ligation is that it relies on the presence of compatible ends created by previous restriction digestion. The advantage of this approach is its historical reliability, as it has been used extensively in various cloning applications. Nonetheless, it can result in lower efficiency with longer DNA fragments, which is a notable disadvantage.
T4 DNA Ligase
T4 DNA ligase derived from the T4 bacteriophage, is a powerful enzyme for ligation. The key characteristic of T4 DNA Ligase is its ability to join DNA strands regardless of whether the ends are sticky or blunt. This flexibility makes it a beneficial choice for diverse cloning applications. Its unique feature is its efficiency in ligating larger DNA fragments, making it suitable for complex cloning strategies. However, one must consider the disadvantage that T4 DNA ligase requires specific buffer conditions, which, if not met, can lead to decreased efficiency in ligation outcomes.
In summary, the techniques in ORF cloning encompass a variety of methods tailored for specific genetic experimentation goals. Understanding these techniques provides researchers with the tools needed to effectively manipulate genetic materials, leading to meaningful scientific advancements.
Challenges in ORF Cloning
Open Reading Frame (ORF) cloning presents multiple challenges that scientists must navigate to ensure successful gene manipulation and characterization. Understanding these challenges is vital to advancing the technique and improving outcomes in research and practical applications. The hurdles faced during ORF cloning can impact the quality and reliability of experimental findings, making them critical considerations for researchers.
Sequence Optimization
Sequence optimization is a requirement in ORF cloning that is essential for enhancing cloning efficiency and expression. Various factors must be addressed during optimization, including codon usage bias, GC content, and secondary structure. Codon optimization helps ensure the ORF translates efficiently within the host organism. This is crucial when transferring sequences between organisms, as different species may prefer certain codons over others.
Poorly optimized sequences can lead to suboptimal protein expression levels, or the protein could misfold, leading to inactive or non-functional products. Researchers often use software tools to analyze and predict ideal codon usage patterns tailored to the specific host. These tools assist in devising constructs that are more amenable to expression systems.
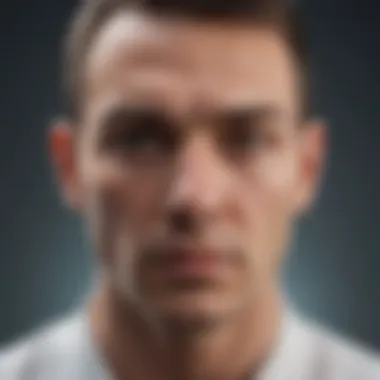
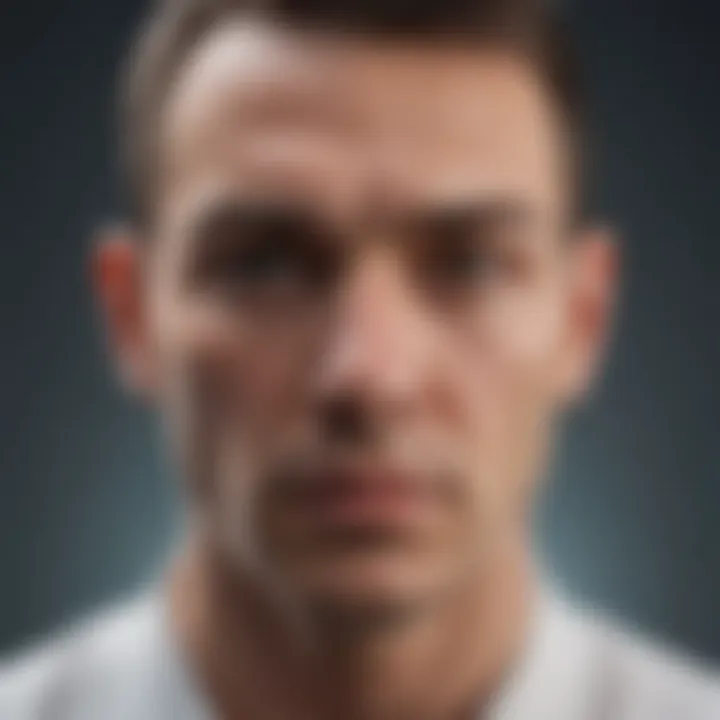
Contamination Issues
Contamination remains a persistent challenge in laboratory settings, significantly affecting the purity and quality of cloned DNA. Contaminants can arise from various sources, including cross-contamination from other samples or microbial contamination. Such issues can lead to false results, increased variability, and misunderstandings in gene function studies.
To mitigate contamination risks, researchers must maintain stringent laboratory practices. This includes using sterile tools, regularly cleaning workspaces, and applying proper disposal measures for biological materials. Additionally, the implementation of controls in experiments can help identify potential contamination, allowing for corrective actions to be taken before drawing conclusions from experimental data.
Proper contamination monitoring is critical for producing valid and reliable results in ORF cloning projects.
Mismatches and Errors
Mismatches and errors during the cloning process can lead to significant setbacks. These errors may arise from various stages of experimentation, including PCR amplification and enzymatic reactions. Mismatches can affect the fidelity of the cloned sequence, resulting in incorrect protein products.
To address these concerns, researchers can use high-fidelity DNA polymerases during PCR to minimize errors in amplification. Regular sequencing of cloned products post-ligation is also necessary to identify and rectify any discrepancies. A rigorous checking process enhances the overall reliability of ORF cloning outcomes, ensuring that subsequent experiments are built on accurate representations of target sequences.
Applications of ORF Cloning
The applications of Open Reading Frame (ORF) cloning in modern science are extensive and significant. This technique serves as a foundational element in various research areas, including molecular biology, biotechnology, and therapeutic developments. Its ability to facilitate the analysis of gene function and protein expression makes it an indispensable tool.
Functional Genomics
Functional genomics involves the study of the relationship between gene expression and biological function. ORF cloning allows researchers to construct specific expression libraries, helping to identify gene functions in an organism's genome. By using cloned ORFs, scientists can express proteins in different systems to determine their roles in biochemical pathways. This can highlight molecular mechanisms that underpin various biological processes, aiding in the advancement of knowledge in fields such as developmental biology and cancer research.
Protein Production and Purification
The production and purification of proteins is another critical application of ORF cloning. Cloned ORFs can be used to express proteins in host systems, such as Escherichia coli or yeast, which often leads to high yields of the desired protein. This is crucial for various scientific and industrial applications, including enzyme production, vaccine development, and therapeutic drug creation. Moreover, successful purification methods facilitate the isolation of proteins from complex mixtures, ensuring that researchers can analyze them accurately and effectively.
Therapeutic Applications
Vaccine Development
Vaccine development significantly benefits from ORF cloning. By using ORF cloning, researchers can efficiently express antigens from pathogens, which can then be used in the development of vaccines. This method has led to improved vaccine formulations by allowing for quicker strain adaptation and antigen selection. The precision of ORF cloning helps in designing vaccines that can elicit potent immune responses with reduced side effects. Studies have shown that this method can streamline the production process, ultimately benefiting public health.
Gene Therapy
Gene therapy is another application that demonstrates the impact of ORF cloning. By creating ORF clones that encode therapeutic proteins, researchers can address genetic disorders more effectively. These clones can be inserted into patients' cells to replace or augment defective genes. The key characteristic of gene therapy is its potential for long-term efficacy and precision in treating diseases at the genetic level. However, the ethical considerations and challenges surrounding delivery methods and immune responses to the introduced proteins can complicate this approach.
Technological Advancements in ORF Cloning
Technological advancements have revolutionized the field of Open Reading Frame (ORF) cloning. These innovations not only streamline existing methods but also enable new applications in molecular biology and genetics. Modern techniques have improved precision, efficiency, and scalability, allowing researchers to tackle complex biological questions with greater ease. The impact on functional genomics, protein synthesis, and therapeutic advancements cannot be overstated.
In this section, we will delve into two significant advancements: Next-Generation Sequencing and CRISPR technology. Each of these innovations brings unique benefits and considerations to the field of ORF cloning.
Next-Generation Sequencing
Next-Generation Sequencing (NGS) has fundamentally altered the approach to DNA analysis. By offering unprecedented speed and accuracy, NGS allows researchers to sequence entire genomes quickly and cost-effectively. In the context of ORF cloning, NGS can identify and validate the desired open reading frames efficiently. This technology reduces the time required for analyzing genetic material, enabling scientists to focus on downstream experiments more rapidly.
Key benefits of NGS in ORF cloning include:
- Precision: Improved accuracy in determining the exact sequence of nucleotides.
- High-throughput capability: Ability to analyze multiple samples simultaneously, enhancing research productivity.
- Comprehensive analysis: Capability to detect variants, enabling a deeper understanding of genetic diversity and function.
Despite its advantages, NGS also comes with challenges. The need for bioinformatics expertise to analyze large datasets can be a barrier for some laboratories. Additionally, the initial costs of sequencing equipment may be high, though the long-term benefits often outweigh these expenses.
"Next-Generation Sequencing is not just about obtaining sequence data; it is about transforming how we understand genomic function and variation."
CRISPR and ORF Cloning
CRISPR technology has emerged as a powerful tool for genome editing, and its integration with ORF cloning is noteworthy. The ability to precisely edit genes allows researchers to create targeted modifications in specific open reading frames. This precision opens possibilities for understanding gene function and protein interaction in ways that were not feasible with earlier technologies.
Utilizing CRISPR in ORF cloning has several implications:
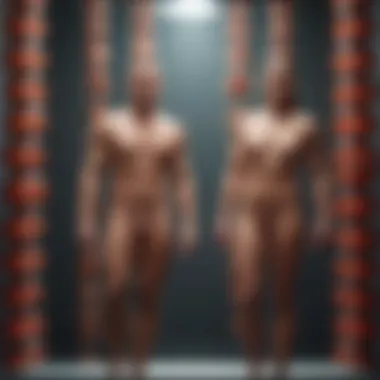
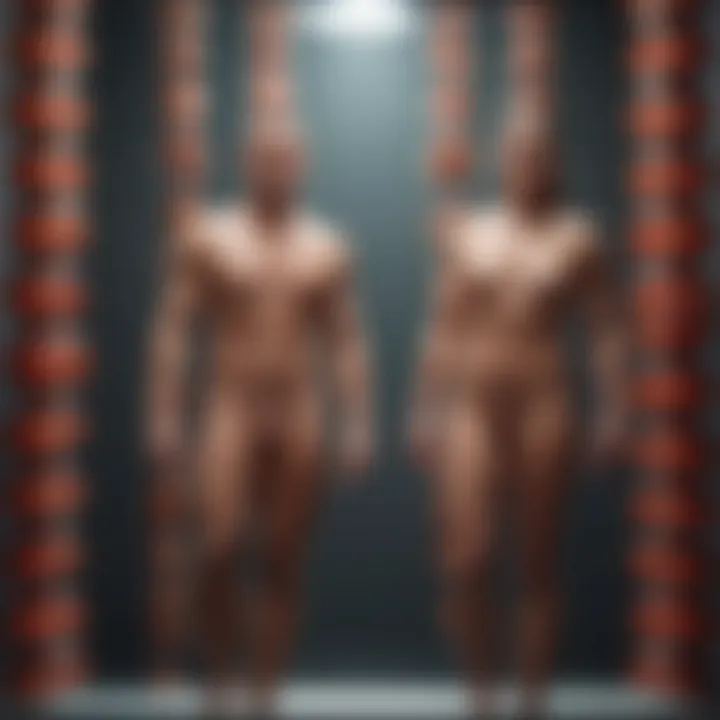
- Targeted gene editing: Modifications can be made at specific loci, allowing for precise alterations in gene function.
- Simplified workflows: The combination of ORF cloning with CRISPR can simplify the process of gene knockout or knock-in experiments, reducing time and labor.
- Enhanced functional studies: Researchers can investigate the effects of these gene modifications on cellular processes and phenotypes in real-time.
However, several considerations must be addressed when using CRISPR with ORF cloning. Off-target effects, where unintended genetic changes occur, could complicate results. Therefore, thorough validation of CRISPR constructs is crucial to ensure accuracy in research findings. Additionally, ethical considerations regarding gene editing continue to pose significant discussions in the scientific community.
Ethical Considerations
As the field of molecular biology progresses, the ethical considerations surrounding techniques like Open Reading Frame (ORF) cloning become increasingly significant. The implications of genetic modification and the responsibilities of scientists cannot be overstated. The decision to manipulate genetic information carries with it a variety of ethical dilemmas that demand careful scrutiny and respectful deliberation.
Genetic Modification Concerns
Genetic modifications through ORF cloning raise substantial ethical questions. Sometimes, the potential for unintended consequences leads to concern among scientists and ethicists alike. For instance, when a gene from one organism is introduced into another, there may be unpredictable effects not only on the organism but also on the ecosystem. The introduction of genetically modified organisms (GMOs) can alter existing biological balances in nature, which might lead to invasive species or biodiversity loss.
Moreover, the moral implications surrounding human genetic modifications fuel heated debates. As scientists explore the potential of gene therapy or enhancements, questions arise regarding consent, access, and societal equity. For example, is it ethical to modify the genes of embryos to prevent genetic disorders? What guidelines should govern such practices?
"Ethical considerations in genetic modification are not just about what we can do, but what we should do," said a prominent bioethicist at a recent symposium.
Regulatory Framework
The regulatory framework surrounding ORF cloning is designed to address these ethical concerns while promoting innovation. It is imperative to establish robust guidelines that govern research practices. Various countries have different regulations concerning genetic modification, and many institutions have their own internal review boards to evaluate proposed studies. These structures aim to ensure that scientific practices adhere to accepted ethical standards and that research outcomes do not pose harm.
Additionally, accountability mechanisms should be in place to oversee how genetically modified organisms are used. There are international treaties and conventions, such as the Convention on Biological Diversity, that address biodiversity concerns and outline governance strategies for genetically modified organisms.
Furthermore, public dialogue plays a vital role in shaping these regulatory frameworks. Engaging with stakeholders, including the public, policymakers, and scientists, fosters transparency and encourages informed decision-making. Ultimately, the goals are to balance the need for scientific advancement with public safety and ethical norms.
In summary, ethical considerations are paramount in ORF cloning. As the field continues to grow, it is crucial for scientists and the regulatory bodies that govern them to work collaboratively. By addressing genetic modification concerns and establishing comprehensive oversight through regulatory frameworks, the scientific community can uphold responsible practices that benefit society.
Future Directions in ORF Cloning
The field of Open Reading Frame (ORF) cloning is poised for significant advancements. Future directions in this area are shaping the scope and impact of molecular biology. As new technologies and methodologies evolve, they bring fresh insights and possibilities. Understanding these developments is crucial because they can lead to enhanced accuracy and efficiency in gene manipulation and analysis.
This section will explore some emerging trends and potential breakthroughs, shedding light on their implications for research and practical applications.
Emerging Trends
One of the most notable trends in ORF cloning is the integration of artificial intelligence (AI) and machine learning. Researchers are beginning to harness these technologies to predict gene behavior and optimize cloning strategies. By analyzing large datasets, AI can identify patterns and outcomes that are not easily discernible through traditional methods. This leads to smarter, quicker decision-making in the cloning process.
Another prominent trend is the shift toward more precision-focused techniques, such as base editing and prime editing. These technologies offer greater specificity and efficiency compared to traditional CRISPR methods. Researchers can now make precise changes to DNA sequences with minimal off-target effects. This promotes better understanding of gene functions and minimizes unwanted mutations.
Crowdsourcing and collaboration in scientific research is also on the rise. Platforms that facilitate collaborative efforts allow researchers from different disciplines to share insights and resources more effectively. This trend enhances the pooling of knowledge and expertise, which can lead to innovative solutions in ORF cloning challenges.
Potential Breakthroughs
As we look to the future, several potential breakthroughs loom on the horizon. One promising area is the advancement of high-throughput screening methods for ORF libraries. Improved techniques will enable the screening of thousands of ORFs simultaneously, accelerating the identification of desired traits or functions in organisms. This gain in speed and efficiency can significantly impact areas like drug discovery and functional genomic research.
Additionally, the application of synthetic biology in ORF cloning stands to revolutionize the field. By designing and constructing new biological parts, functions, or systems, synthetic biology can create organisms with exceptional properties. This has implications for biotechnology, pharmaceuticals, and environmental science. Such tailored organisms could lead to the development of new therapies or sustainable solutions to pressing challenges.
Finally, the merging of genomic technologies with ORF cloning is another exciting frontier. The integration of genomic data and gene editing techniques may lead to more potent genetic modifications. This can improve gene therapy approaches, offering hope for addressing genetic disorders more effectively.
In sum, the future of ORF cloning holds great promise. As the scientific community embraces these trends and breakthroughs, the potential for impactful discoveries will expand significantly. The ongoing evolution of ORF cloning techniques will continue to enhance our understanding of genetics, paving the way for advancements in numerous fields.
Closure
The conclusion serves as a crucial recapitulation of the extensive discussions surrounding Open Reading Frame (ORF) cloning. This section emphasizes the significance of understanding not just the techniques but also the implications of ORF cloning in modern scientific research. The insights gained from prior sections can have substantial benefits for students, researchers, educators, and industry professionals alike, enhancing their approach to molecular biology.
Summary of Insights
The exploration of ORF cloning has uncovered multiple dimensions of gene function and associated technological advancements. Key takeaways include:
- Execution of techniques: Various methodologies, such as plasmid vectors and PCR amplification, provide researchers with tools that are adaptable to different experimental setups.
- Applications in the field: From functional genomics to therapeutic developments, the applications directly impact various branches of biology. Researchers can leverage these techniques for vaccine development and gene therapy projects.
- Navigating challenges: The section on challenges discusses issues such as sequence optimization and contamination, which can hinder research outcomes. Addressing these matters is crucial for successful ORF cloning.
Final Thoughts
The journey through this article elucidates that ORF cloning is not merely a molecular technique but a gateway to deeper understanding in genetics. As researchers continue to innovate, the relevance of ORF cloning will likely amplify, especially in an era of rapid technological advancement.
Ultimately, awareness of ethical considerations and regulatory frameworks is important for shaping the future direction of gene manipulation and other related practices in the life sciences. As contemporary issues arise, the insights from ORF cloning will guide development and application in a responsible manner.
The study of ORF cloning stands as a testament to how science evolves through innovation, collaboration, and ethical responsibility, shaping a future where biological research can significantly improve human health and environmental sustainability.