Gamma Radiation Shield: Importance and Applications
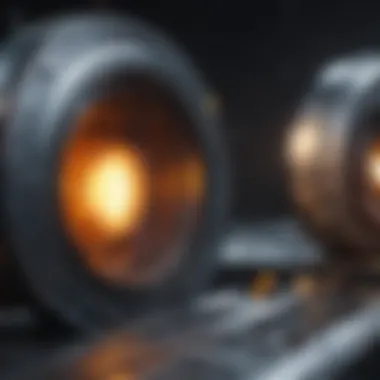
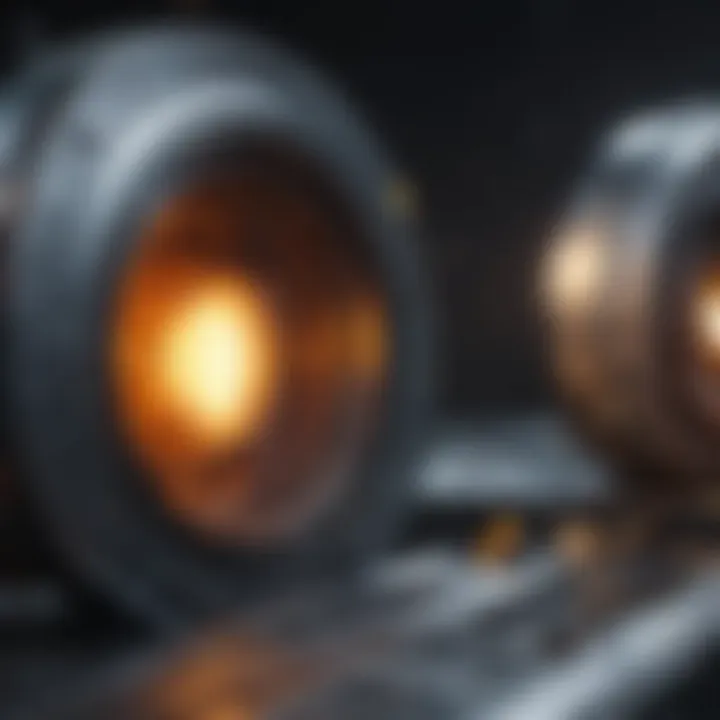
Intro
Gamma radiation, a form of electromagnetic radiation, poses significant risks to human health and the environment. Unlike alpha and beta particles, gamma rays have high penetration ability, making them particularly hazardous. Therefore, the development and implementation of gamma radiation shields have become essential in both scientific research and various industrial applications. This article examines why gamma radiation shielding is not just a technical requirement but a crucial component of safety protocols in environments where exposure to radioactive materials is a concern.
Overview of Research Topic
Brief Background and Context
Gamma radiation shielding has a long historical backdrop, intertwined with the advancement of nuclear science and medicine. The need for effective shielding grew alongside the emergence of nuclear power and radiation therapy used in treating diseases like cancer. Early shielding efforts primarily focused on utilizing dense materials that could absorb or deflect gamma rays, but as technology advanced, more sophisticated materials and methods were developed.
Importance in Current Scientific Landscape
Today, the relevance of gamma radiation shields cannot be overstated. As industries continue to evolve with new discoveries in areas such as nuclear energy, medical imaging, and materials science, the safety concerns persist. Regulatory bodies mandate the use of shields to protect workers, scientists, and the broader public from the detrimental effects of gamma radiation exposure. Additionally, advancements in materials science, such as the introduction of polymer-based shields, open new avenues for both effectiveness and practical usability.
"In an age where radiation is prevalent, understanding shielding technology is not just for scientists — it's a societal necessity."
Methodology
Research Design and Approach
This article employs a comprehensive review methodology to explore gamma radiation shielding. By synthesizing existing research and literature, insights into the primary materials used, the effectiveness of various shielding techniques, and innovations in shielding technologies are examined in detail. Both qualitative and quantitative studies are included to provide a balanced view of the topic.
Data Collection Techniques
Data has been gathered from a variety of reputable sources, including academic journals, industry reports, and case studies related to gamma radiation shielding. Publicly available information and expert interviews also contribute to a rounded understanding of current practices in the field. This multi-faceted approach ensures clarity and accuracy in presenting the subject matter to various audiences, ranging from students to industry professionals.
Preamble to Gamma Radiation
Gamma radiation is a type of high-energy electromagnetic radiation, which plays a pivotal role across various fields, ranging from healthcare to industrial applications. Understanding gamma radiation is not just a technical necessity but also a fundamental aspect of ensuring safety in environments where exposure is possible. This section lays the groundwork by discussing its nature, sources, and potential health risks, thus presenting a clear picture of why gamma radiation warrants diligent study.
Nature of Gamma Radiation
Gamma radiation is known for its penetrating power, which makes it distinct from other forms of radiation, such as alpha and beta rays. Its high frequency and short wavelength give it the ability to traverse most materials with ease. For instance, while a sheet of paper can halt alpha particles, gamma rays can pass through them like a hot knife through butter. This property of gamma radiation necessitates the implementation of effective shielding to safeguard individuals and equipment from its harmful effects.
Additionally, it’s crucial to grasp that gamma rays are produced during radioactive decay, nuclear reactions, or certain types of particle collisions. Understanding these mechanisms helps in appreciating the various scenarios in which gamma radiation may be encountered—not just in controlled environments but increasingly in everyday life as our technology evolves.
Sources of Gamma Rays
The origins of gamma rays can be quite diverse. They emanate from both natural and artificial sources. Some notable examples include:
- Cosmic sources: Gamma rays can come from outer space, produced by celestial events such as supernovae and neutron stars.
- Radioactive materials: Common isotopes like Cobalt-60 and Cesium-137, typically used in medical and industrial applications, emit gamma radiation during decay.
- Nuclear reactors: Operations in reactors or during the nuclear fuel cycle frequently release gamma rays.
It's essential to recognize that, whether in hospitals for cancer treatment or in nuclear power plants, monitoring these sources plays a vital role in radiation protection.
Health Risks Associated with Gamma Radiation
Exposure to gamma radiation, especially over extended periods, can present significant health hazards. Notably, high doses can lead to radiation sickness, which is marked by symptoms ranging from nausea to severe organ damage, depending on the intensity of exposure. Moreover, prolonged exposure is often linked with an increased risk of cancer.
"Health risks linked with gamma rays underscore the importance of implementing stringent safety measures in workplaces where radiation is present."
To mitigate these risks, it's recommended to adopt a combination of safety protocols which can include:
- Regular monitoring: Utilizing devices that measure radiation levels helps to create a safe working environment.
- Training personnel: Educating workers about safe handling of radiation sources is crucial in avoiding unintended exposure.
- Implementing shielding technologies: Investing in effective shielding materials minimizes risks associated with gamma radiation.
Through solid understanding and awareness of the nature, sources, and health risks posed by gamma radiation, it becomes clear why shielding devices are indispensable in countless settings. The next sections will further explore the principles of radiation shielding, the materials used for construction, their applications, and the evolving threats and solutions regarding radiation safety.
Fundamentals of Radiation Shielding
When discussing gamma radiation, it's crucial to get a grip on the basics of radiation shielding. This section serves as a foundation upon which effective shielding strategies are built. Understanding these fundamentals not only enhances the safety measures taken in various fields but also helps professionals make informed decisions regarding their radiation protection strategies.
Understanding Radiation Interaction with Matter
At the heart of shielding is the way radiation interacts with matter. Gamma rays possess a high energy and a considerable penetration ability, meaning they can travel through various materials. To combat this phenomenon, it’s important to know how gamma rays interact with different substances. The primary mechanisms at play are photoelectric absorption, Rayleigh scattering, and Compton scattering.
- Photoelectric Absorption: This occurs when a gamma photon is completely absorbed by an atom, therefore transferring all its energy to an electron and causing it to be ejected from the atom. This type of interaction is more likely to happen in materials with a high atomic number, making lead a staple in shielding.
- Rayleigh Scattering: In this process, gamma rays bounce off atoms without losing energy. While this interaction isn’t as impactful in terms of shielding, it plays a role in how radiation is distributed in a space.
- Compton Scattering: Here, the gamma photon collides with an electron, resulting in energy transfer. The outcome is a gamma ray of reduced energy and a scattered electron. This interaction is significant in organic materials.
Each of these interactions highlights the complexity involved in designing effective shields, which must account for the energy levels and types of the gamma rays being dealt with. Knowing how different materials perform under radiation exposure allows for more strategic choices in materials used for shielding.
The Role of Absorption and Scattering
The interaction of gamma radiation with materials often boils down to two critical processes: absorption and scattering. Both play vital roles in the efficacy of radiation shields:
- Absorption is the process crucial for diminishing the intensity of radiation. It’s the fundamental principle that allows materials like lead to effectively block gamma rays. The greater the absorption, the less radiation permeates through the shield.
- Scattering, on the other hand, changes the direction of gamma rays without necessarily absorbing them completely. This phenomenon can relay a portion of radiation into neighboring spaces, which can be a significant consideration in specific setups, especially in medical facilities or nuclear plants.
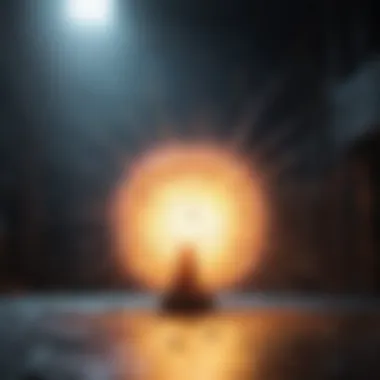
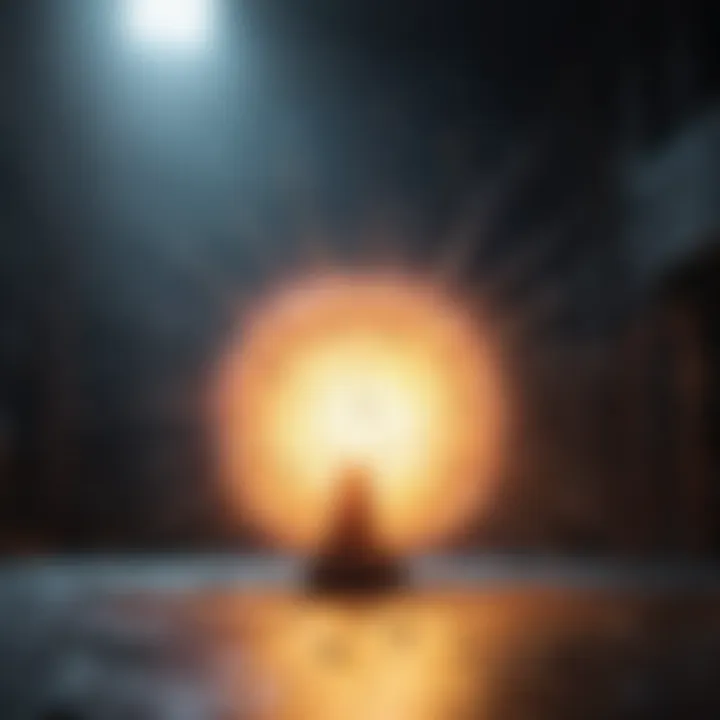
In summary, understanding the complexities of radiation shielding presents a necessary groundwork for further exploration into the materials and types of shields that will be discussed later in the article. Each element, from the atomic structure of materials to the energy of radiation, influences the effectiveness of radiation shields. Adequate knowledge about these fundamentals paves the way for designing safe and effective shielding solutions.
Materials Used in Gamma Radiation Shields
When it comes to shielding against gamma radiation, understanding the materials utilized in such barriers is pivotal. The effectiveness of a shielding solution depends largely on its material properties, including density, atomic number, and overall structure. In radiation protection, materials help to minimize exposure risks, ensuring safety in different environments—be it a hospital, an industrial site, or a research facility.
Lead: The Traditional Shielding Material
Lead has long been the go-to material for radiation shielding. The appeal lies primarily in its high density, which plays a significant role in its effectiveness. Gamma radiation can penetrate materials more easily than other types of radiation like alpha or beta, which means that lead’s density acts as a formidable barrier. It’s been said that just a few millimeters of lead can significantly reduce gamma ray intensity. This makes lead particularly useful in medical applications, like X-ray rooms or radiology departments.
However, there are considerations. First off, lead is heavy, which poses transportation and installation challenges. Furthermore, it can be toxic if not handled correctly. Alternatives do exist, each with their own set of pros and cons, but lead remains a classic choice, especially in settings where space is limited, and efficiency is paramount.
Concrete and Its Applications
Concrete is often viewed as an unassuming choice for radiation shielding, yet it holds its own when it comes to gamma radiation. Its density can be increased by adding materials like barite or iron, which enhances its effectiveness. The versatility of concrete reflects in its varied applications—from protecting nuclear power plants to creating barriers in medical facilities.
One of the standout benefits of concrete is its abundance and cost-effectiveness. Large structures can be built at a relatively low cost and without the concerns associated with some other materials, such as lead. However, it’s worth noting that while concrete is efficient, it requires thickness to be truly effective against gamma rays; thinner walls might not provide adequate protection.
Innovative Shielding Materials
Polymer-based Shields
Polymers have entered the radiation shielding conversation in a big way. With advancements in material sciences, polymer-based shields can be crafted to provide effective protection against gamma rays. One of the key characteristics of these materials is their lightweight nature, making them easier to transport and handle compared to traditional materials like lead.
The unique feature of polymer shields is their ability to be customized. They can be designed to meet specific needs, accommodating various environmental factors. Despite their benefits, polymers may have limitations in extreme radiation environments, and it is crucial to analyze performance based on specific scenarios.
Borated Materials
Borated materials incorporate boron into the shielding matrix, which proves advantageous due to boron's ability to absorb neutrons, alongside offering some protection against gamma rays. This makes borated materials a popular choice in situations where neutron radiation is also a concern, such as in nuclear power facilities.
The uniqueness lies in their dual functionality. They serve well in shielding against several types of radiation. However, the cost of borated materials can be higher, and their overall effectiveness can vary, depending on the gamma energy levels involved.
High-density Foam
High-density foam is another innovative material that has started to gain traction in radiation shielding scenarios. This key characteristic is its flexibility combined with a lightweight design, allowing it to fit around complex shapes and spaces unsuitable for heavier materials.
The advantage of high-density foam is its ease of use in various applications, from custom-made shields to even portable devices. However, its limitations come into play when assessing its protective capabilities against high-energy gamma radiation; while it can absorb low-energy radiation effectively, the same cannot always be said for more powerful gamma rays.
"Selecting the appropriate material for gamma radiation shielding can significantly affect the safety and efficacy of radiation protection measures in various settings."
In sum, each of these materials presents its own strengths and potential drawbacks. A thorough understanding of the context in which these materials will be used is crucial for selecting the most appropriate shielding type and ultimately ensuring the safety and protection of personnel in radiation-prone environments.
Types of Gamma Radiation Shields
Understanding the various types of gamma radiation shields is paramount for ensuring safety and effectiveness in environments where exposure to gamma rays is a concern. These shields come in different designs, each with specific advantages tailored to various scenarios. Choosing the right type is crucial, as it can greatly impact both protection and operational flexibility. In this section, we will delve into three main categories of gamma radiation shields: fixed shielding structures, portable shielding solutions, and customizable shielding units.
Fixed Shielding Structures
Fixed shielding structures are often employed in environments where radiation exposure is a constant threat. These installations can be found in hospitals, research laboratories, and nuclear facilities. The main aim of these structures is to create a permanent barrier against gamma rays, ensuring continual protection for both personnel and sensitive equipment.
Key benefits of fixed shielding include:
- Durability: Constructed from heavy materials like lead or thick concrete, these shields are built to last, effectively stopping gamma radiation from penetrating.
- Space Efficiency: Once established, they do not consume additional space, allowing for straightforward integration into existing facility layouts.
- Consistent Protection: They provide uninterrupted coverage, which is crucial in environments with continual radiation exposure.
However, one must consider the potential limitations:
- Cost: Initial construction can be expensive, often requiring significant investment in both materials and labor.
- Inflexibility: Once set in place, these structures cannot be easily modified or relocated to adapt to changing needs.
"The choice of a fixed shielding solution is a balancing act between safety requirements and practical considerations like space and cost."
Portable Shielding Solutions
Portable shielding solutions are a remarkable evolution in gamma radiation protection technology. Designed to be movable, these shields are invaluable in field operations and temporary settings. They cater to situations where radiation exposure is sporadic or variable, providing flexibility and convenience.
These solutions can range from foldable lead-lined curtains to mobile radiation barriers. Their advantages include:
- Versatility: Portable shields can be transported and deployed in various locations as needed, making them useful for both short-term and long-term operations.
- Ease of Use: Quick setup and takedown allow for on-the-spot protection without requiring extensive logistical planning.
- Cost-effective: They can serve as a more budget-friendly option for facilities with limited resources while still maintaining safety standards.
Nevertheless, portability comes at a cost. These shields might not provide the same level of protection as fixed structures due to material limitations and size constraints. They may also require personnel training for proper usage during deployment.
Customizable Shielding Units
Flexibility is the name of the game when discussing customizable shielding units. These are engineered to meet specific operational needs and challenges, allowing for tailored solutions to protect against gamma radiation effectively.
Customization options can include modifying the size, shape, and material of the shields based on the precise nature of radiation exposure and environmental conditions. Benefits include:
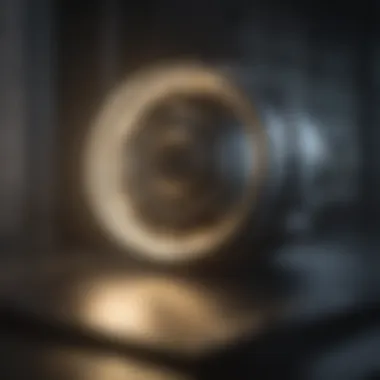
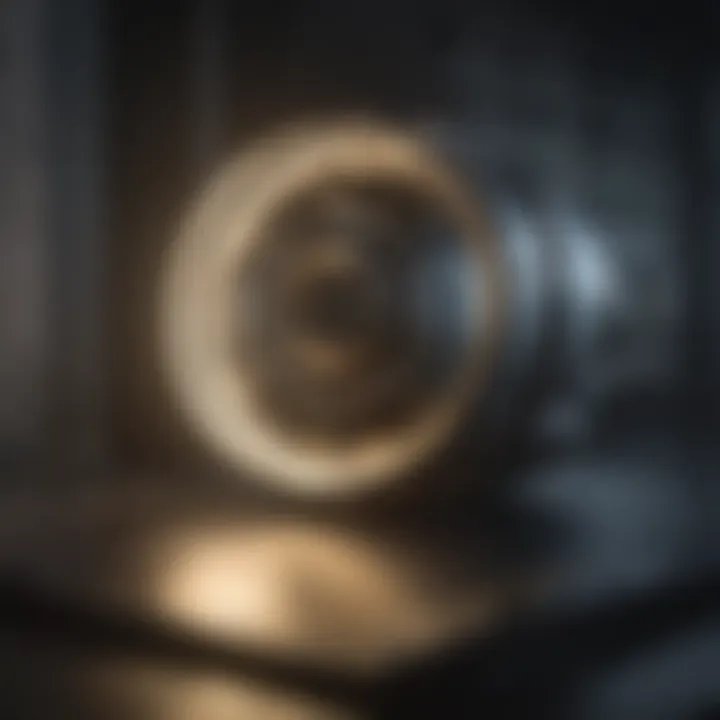
- Tailored Protection: Custom designs ensure that specific vulnerabilities are addressed, enhancing operational safety.
- Adaptive Use: As demands change, these units can be redesigned or adjusted to accommodate new risks or facility configurations.
- Integration with Other Safety Measures: Often, these units can be combined with other safety technologies, such as imaging systems or monitoring devices, to create a comprehensive radiation safety strategy.
On the flip side, the process may require additional lead time for design and production. This could delay deployment, and the complexity of customization can elevate overall costs. Not every facility might find it necessary to invest in such adaptable solutions, making this approach more suited for specialized fields.
Design Considerations for Effective Shielding
Designing effective gamma radiation shielding involves multiple crucial factors that must be meticulously taken into account. By understanding these elements, we can ensure both the safety of individuals and the effective mitigation of harmful radiation exposure. This section takes a comprehensive look at important aspects like shield thickness calculation, geometry and shield configuration, and conducting a cost-benefit analysis of shielding solutions. Each of these considerations intersects to create a robust framework for effective shielding practices.
Shield Thickness Calculation
One fundamental aspect of designing gamma radiation shields is calculating the appropriate thickness. The effectiveness of a shield is primarily determined by its ability to absorb radiation. The inverse square law suggests that radiation intensity diminishes with distance, but when it comes to materials, sheer thickness often does the trick. The more material, the greater the potential for absorbing gamma rays.
This involves employing the half-value layer (HVL) concept, which indicates the thickness of a material required to reduce gamma ray intensity by half. Different materials serve various purposes in shielding, dictated by their distinct HVLs. For example, lead boasts a much lower HVL compared to concrete, making it a popular choice in many settings.
To determine the exact shield thickness for a specific application, one may rely on calculations that factor in gamma radiation energy, material density, and desired level of protection. These calculations can become quite complex, sometimes requiring advanced software tools. However, the principle remains straightforward: thicker material equates to an increased ability to block radiation. Proper calculations ensure that the shield provides adequate protection without excessive use of resources.
Geometry and Shield Configuration
Equally critical is the geometric arrangement of shielding materials. The shape and layout can significantly affect their effectiveness against gamma rays. For instance, a shield that is too thin in one area while excessively thick in another can create weak points, allowing radiation to penetrate more easily. Careful design can enhance shielding efficiency, thus providing better overall safety.
Designers often propose using multi-layered configurations where different materials with varying density levels are combined. Such configurations capitalize on the distinct absorption properties of each layer. The outer layer might consist of a dense material, like lead, followed by an intermediate layer of a lighter shield, such as concrete, and finally an absorbent foam. This systematic layering can enhance the absorption and scattering effects, effectively breaking down hazardous gamma rays before they reach their intended target.
Notably, spatial considerations should also come into play. The placement of the shielding against the source of gamma radiation affects its efficiency. For example, in a medical imaging environment, often the placement of a shield between the source and a patient or technician can minimize exposure to radiation.
Cost-Benefit Analysis of Shielding Solutions
Finally, undertaking a thorough cost-benefit analysis is essential in determining the most effective shielding solutions. Various materials and designs come with different price tags, and affording the best option may not always be feasible. Analyzing the return on investment (ROI) involves understanding initial costs versus long-term savings from potential health risk reductions and regulatory compliance.
Key considerations in this analysis often include:
- Initial Material Costs: How much do the chosen materials cost?
- Installation Expenses: What are the labor costs associated with implementing the shielding?
- Maintenance and Upkeep: How often will the shielding need to be replaced or maintained?
- Regulatory Compliance Costs: Are there penalties for non-compliance, and how might these influence your choice?
By factoring in these elements, one can arrive at a practical decision that maximizes safety without breaking the budget. It’s all about finding that sweet spot between performance and cost-efficiency, ensuring that the shielding solutions serve their intended purpose without draining resources.
Safety Guidelines and Regulations
Safety guidelines and regulations in the context of gamma radiation shielding are paramount, ensuring not only the well-being of individuals but also the efficacy of radiation protection measures. These protocols safeguard both workers in environments like healthcare and nuclear industries, as well as the general public, from the potentially harmful effects of gamma rays. The effectiveness of shielding is heavily dependent on adhering to these guidelines, which provide a framework for best practices and usage of shielding materials.
International Standards for Radiation Protection
International standards play a crucial role in establishing the benchmarks for radiation protection. Institutions such as the International Atomic Energy Agency (IAEA) and the World Health Organization (WHO) outline comprehensive measures designed to minimize radiation exposure. These standards involve the following key elements:
- Recommended Exposure Limits: International standards set limits on the amount of radiation that a person may be exposed to over a defined period.
- Classification of Areas: Environments are categorized based on radiation levels—controlled areas, supervised areas, and public areas. This classification helps in implementing specific safety measures tailored to the risk levels.
- Quality Assurance: Regular monitoring and evaluation of shielding effectiveness are required to ensure that protective measures stay relevant and effective over time.
Following these internationally recognized protocols not only fosters a safe environment but also encourages best practices in gamma radiation awareness on a global scale.
Implementation of Safety Protocols
The implementation of safety protocols is as important as establishing them in the first place. Safety protocols encompass a series of systematic steps to ensure that personnel are adequately protected from gamma radiation. Some core components of these protocols include:
- Training Programs: Regular training ensures that all personnel understand the risks associated with gamma radiation and know how to use shielding effectively.
- Personal Protective Equipment (PPE): Alongside shielding materials, the use of PPE such as lead aprons in clinical settings significantly reduces exposure.
- Emergency Procedures: Detailed emergency procedures must be in place to respond effectively in case of radiological incidents. Regular drills can keep everyone prepared.
- Signage and Alerts: Clearly marked signs indicating radiation zones help maintain awareness and mindfulness regarding safety.
Incorporating these protocols into daily operations ensures that both individuals and organizations comply with regulations and protects against unintentional exposure. Ultimately, the commitment to safety guidelines and regulations establishes a culture of vigilance and respect for gamma radiation, one which is critical for ongoing protection in all relevant fields.
Applications of Gamma Radiation Shields
Gamma radiation shields play a crucial role in various sectors, protecting individuals and environments from the potentially harmful effects of gamma radiation. As we examine the importance of these shields, it becomes evident that their applications span healthcare, industry, and research, each with specific benefits and considerations relevant to the field. Understanding these applications helps to emphasize why rigorous radiation shielding is essential in many modern technologies and practices.
Healthcare and Medical Imaging
In the realm of healthcare, gamma radiation shields are indispensable, particularly in medical imaging. Techniques like Positron Emission Tomography (PET) and single-photon emission computed tomography (SPECT) utilize gamma rays to create detailed images of the body. Here, shielding is not just an option; it is a necessity for both patients and healthcare professionals.
- Patient Safety: The use of shielding significantly minimizes radiation exposure for patients undergoing diagnostic tests. This fosters a safer environment while maintaining diagnostic accuracy.
- Staff Protection: Medical staff, including radiologists and technicians, often work with radioactive materials. Proper shielding ensures they are protected from excessive exposure, thereby adhering to safety regulations.
In addition, facilities are designed with specific shielding materials like lead-lined walls and protective barriers. This not only prevents radiation leakage but also promotes compliance with health regulations.
Industrial Use Cases
The industrial sphere also leverages gamma radiation shields extensively, particularly in sectors involving nuclear energy, manufacturing, and construction. In these sectors, gamma radiation can emanate from various processes or materials, making robust shielding critical.
- Nuclear Power Plants: Shields are fundamental in control rooms and areas surrounding reactors to protect workers from radiation. High-density concrete or lead barriers are often employed in these settings.
- Radiography in Manufacturing: Industrial radiography uses gamma rays to inspect welds and materials for quality control. Shielding prevents radiation from escaping these areas, ensuring worker safety while allowing for thorough inspections.
Employing effective shielding not only protects employees but also helps industries avoid hefty fines related to safety violations.
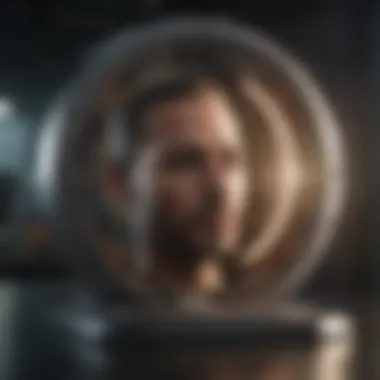
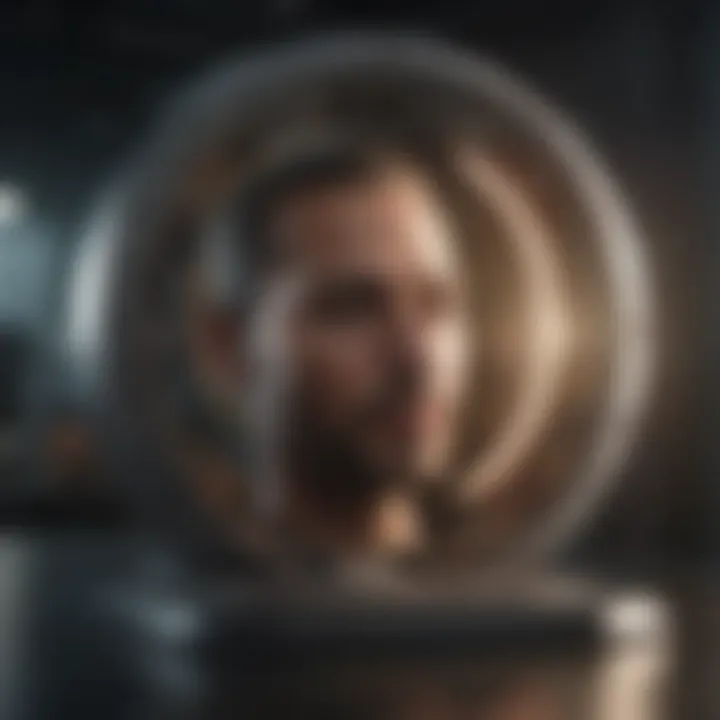
Research and Development Environments
In research and development settings, such as physics or medical research laboratories, gamma radiation shields are pivotal for safe experimentation. Research involving radioactive isotopes or radiation-producing equipment often requires careful shielding considerations to foster a safe working environment.
- Laboratory Design: Effective lab design incorporates shielding into its layout. Typically, research facilities utilize materials that provide adequate protection, enhancing the safety of researchers.
- Innovation Testing: When developing new technologies that employ radiation, adequate shielding must be tested alongside the technology to ensure compatibility and safety for future applications.
Research environments thrive on safety. Hence, the correct application of gamma radiation shields enhances research integrity and promotes innovation.
"Without proper shielding, both worker safety and research integrity might be at risk, hindering scientific advancement and posing severe health threats."
Through these applications, it is clear that gamma radiation shielding is not merely a technical requirement but a fundamental aspect of protecting health and promoting advancements across various fields. As technologies evolve, the importance of investing in better shielding solutions will only become more apparent.
Emerging Technologies in Radiation Shielding
The field of radiation shielding is undergoing a transformation with the advent of various emerging technologies. These new approaches are reshaping how we protect ourselves from harmful gamma radiation, making shielding not only more effective but also more efficient. Understanding these technologies is critical for researchers and professionals in industries where radiation is a concern. The balance of safety, cost, and material performance is paramount in this field, which is where these recent advancements come into play.
Advanced Materials Research
Nanotechnology
Nanotechnology plays a pivotal role in advancing radiation shielding due to its ability to manipulate materials at the molecular level. This aspect of research helps create materials that can efficiently absorb or scatter gamma rays more effectively than traditional materials. One of the key characteristics of nanotechnology is its capacity to enhance the properties of existing materials. For instance, when nanoparticles are integrated into polymers, the resulting composite can exhibit significant improvements in density and stability.
Moreover, nanotechnology provides unique features, such as increased surface area and customized interactions at the atomic level, which are advantageous in reducing radiation exposure. However, there are challenges to consider as well. The manufacturing processes for these nanomaterials can be complex and costly, which may limit their widespread application.
Smart Shielding Techniques
Smart shielding techniques represent another innovative facet of radiation protection. These methods leverage technology to create dynamic shielding solutions that can adapt to varying radiation levels. The key characteristic of smart shielding is its responsive nature—some materials can change properties when exposed to radiation, providing enhanced protection only when needed. This adaptability makes them a popular choice in environments where radiation levels can fluctuate.
An important unique feature of smart shielding is the integration of sensors that monitor radiation exposure in real-time. This allows for immediate adjustments in shielding effectiveness, maximizing safety while minimizing unnecessary materials and costs. On the downside, these systems can be quite complicated and may require regular maintenance to ensure functionality.
Simulation and Modeling in Shield Design
Simulation and modeling technology is essential in the design of effective radiation shields. By employing sophisticated software tools, engineers can predict how different materials will interact with gamma radiation before physical prototypes are built. This not only accelerates the design process but also reduces costs related to materials and testing.
Simulation encompasses various strategies, including Monte Carlo simulations, which model the random interactions of particles with matter to obtain statistical data on shielding effectiveness. This technique allows designers to visualize the impact of different shielding configurations and materials under specific conditions.
Through advanced computational models, the design process for radiation shields becomes more insightful and data-driven, leading to enhanced designs that meet safety standards while also considering economic factors. The combination of these technologies marks a significant leap forward in ensuring radiation protection, making every advancement a critical piece in the ongoing effort to safeguard public health and safety.
Future Directions and Challenges
The field of radiation shielding, particularly in regards to gamma radiation, is continuously evolving. As technology advances, it becomes paramount to address various challenges and opportunities that play a critical role in enhancing safety and effectiveness. Understanding these future directions can shape how we approach radiation shielding and instigate a proactive stance towards environmental and health concerns.
Addressing Environmental and Health Concerns
One of the most pressing issues we face in radiation shielding is ensuring that materials used do not inadvertently harm our environment or public health. With the world focusing increasingly on sustainability, the pressure mounts to adopt materials that are both effective against gamma radiation and environmentally safe.
For instance, lead has been the staple material for radiation shielding, famous for its high density and effectiveness. However, its heavy metal nature raises concerns regarding its disposal and potential environmental impact. Researchers are exploring alternatives such as borated materials or polymer-based shields, which could offer comparable protection without the hazardous footprint.
Furthermore, it's crucial to consider how these materials behave over time in real-world conditions. Efforts are underway to develop biodegradable shielding options or materials that break down safely. This shift not only carries promise for the environment but also encourages the adoption of circular economy principles in the field of radiation safety.
"The quest for environmentally sound shielding solutions stands at the intersection of technology and responsibility."
Improving Public Understanding of Radiation Safety
Another vital challenge is the public's perception and understanding of radiation safety. Misinformation often circulates regarding the dangers of gamma rays, leading to unnecessary fear. Clear, comprehensive information regarding the risks and benefits of gamma radiation and shielding is essential for informed decision-making.
Educational campaigns targeted at different demographics can foster a more nuanced understanding. For instance:
- Schools and Universities should integrate radiation safety topics into their curriculums, teaching students not only about gamma radiation but also about the practical applications of shielding in medicine and industry.
- Public Forums and Workshops can engage community members, demystifying radiation and showcasing how shielding helps in various fields.
- Online Resources and Social Media platforms can be harnessed to spread awareness, leveraging the digital age to communicate effectively with a far-reaching audience.
End
In summarizing the insights around gamma radiation shielding, it's imperative to grasp how indispensable these protective measures are in safeguarding both human health and the environment. As we have delved throughout the various sections of this article, the need for effective gamma radiation shields is multifaceted. From their role in healthcare settings, where medical imaging necessitates safety from exposure, to industrial applications in nuclear facilities or research labs, the ability to shield against harmful rays is crucial.
A stratified understanding was put forth—beginning with the nature and sources of gamma radiation, progressing through the specifics of materials used in shielding, and finally examining the diverse applications. Implications stretch beyond mere compliance with safety regulations; they touch on ethical considerations about ensuring a safe working environment for all involved.
Moreover, the design factors, safety protocols, and advancement in technologies like nanotechnology and smart shielding introduced throughout provide a holistic view of how we can improve our approaches to radiation protection.
"Understanding the importance of gamma radiation shielding is not just about protection, but about pushing the envelope in safety and innovation in our scientific pursuits."
Recap of Key Points
- Nature of Gamma Radiation: We explored gamma rays' intrinsic properties and the roles they play as both a tool and a hazard in our society.
- Shielding Materials: The comparative study of traditional materials like lead versus more innovative options highlighted the evolution of shielding technology.
- Applications: Emphasis on diverse applications in healthcare, industry, and research cemented an understanding of the shields' critical importance across different settings.
- Safety Measures and Regulations: Knowledge of international standards is fundamental to ensuring protection measures are in place and adhered to.
The Importance of Continued Research
The landscape of radiation protection is dynamic, reflecting technological advancements and a deeper understanding of the implications of radiation exposure. Continued research in gamma radiation shielding remains vital to address emerging challenges, such as:
- Environmental Concerns: As industries evolve, the impact of shielding materials on the environment must be a point of investigation. Research can lead to the development of more sustainable shielding options, without compromising protection.
- Health and Safety: The relationship between exposure levels and long-term health effects continues to warrant scrutiny. Ongoing studies are necessary to refine guidelines and enhance safety protocols.
- Innovative Technologies: Cutting-edge research into smart materials and real-time monitoring solutions holds promise for revolutionizing how we approach radiation shielding.
In summary, as the fields of science and technology push boundaries, maintaining a robust focus on research in gamma radiation shielding will substantially benefit future safety practices and innovations. The journey towards better protection and understanding of gamma radiation is far from over.