Exploring the Complex World of Semiconductor Devices
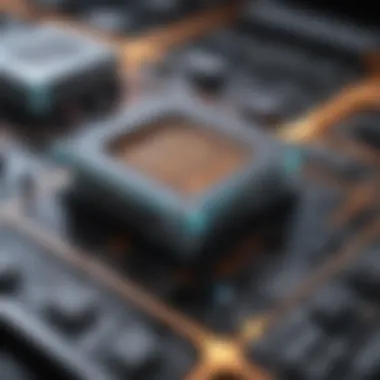
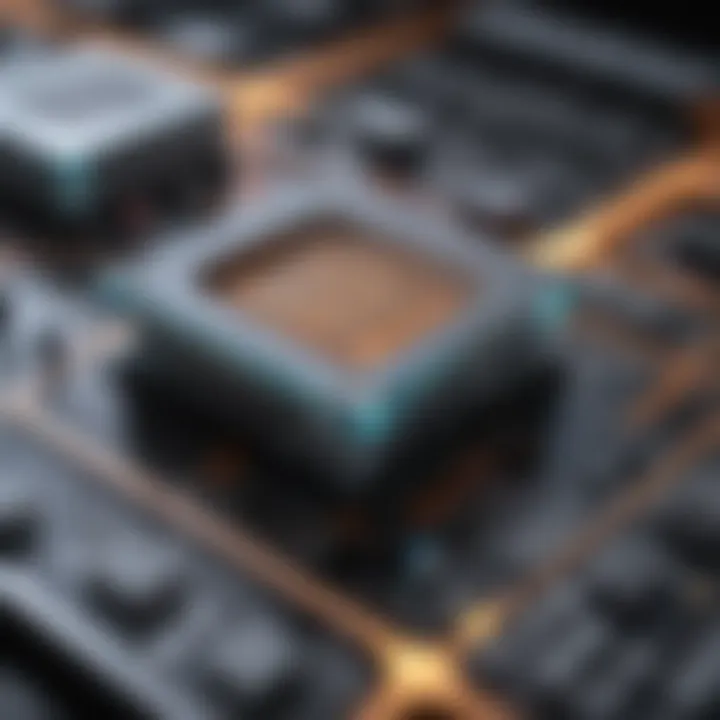
Intro
In the ever-evolving landscape of technology, semiconductor devices stand as a cornerstone of modern electronics. These crucial components facilitate the manipulation of electrical signals in a myriad of applications, from simple household gadgets to complex computing systems. Understanding semiconductor devices is not just an academic endeavor; it is essential for anyone interested in how contemporary technology operates.
As we embark on this journey, we will dissect the very fabric of semiconductor technology, exploring the intriguing features, capabilities, and the mechanics that drive their functionality. Taking a closer look at their diverse applications across various sectors will also shed light on the pivotal role semiconductors play in everyday life.
With innovations surfacing rapidly, the discussion will naturally gravitate toward emerging technologies, like quantum dots and organic semiconductors, that are reshaping the future of electronics. \n Thus, we will delve into the past, examine current trends, and look ahead, providing readers with not only a historical context but also insights into future developments that could revolutionize how we interact with technology.
Preface to Semiconductor Devices
Semiconductor devices stand at the heart of modern electronics. Their significance cannot be overstated, considering they are integral to everything from the smallest mobile devices to the colossal computing servers that power the internet. The discussion on semiconductor devices encompasses various aspectsâhow they function, the materials they are made from, their manufacturing processes, and their applications across different sectors.
In essence, exploring semiconductor devices is akin to peeling back the layers of a complex onion. Each layer reveals critical information that illustrates why these components are the backbone of contemporary technology. They enable the control of electrical current, making it possible for us to put the power of technology right at our fingertips.
Definition and Importance
At its core, a semiconductor is a material whose electrical conductivity falls between that of a conductor and an insulator. This unique characteristic is what allows semiconductors to control electrical current, enabling a host of electronic functions. The ability to manipulate electrical signals with precision forms the basis for all semiconductor devices, whether they are used in transistors, diodes, or integrated circuits.
In practical terms, the importance of semiconductors is highlighted by the fact that they are found in nearly every electronic device. From smartphones and laptops to medical equipment and vehicle control systems, semiconductors help make technology more efficient and versatile. Without them, our gadgets would be much less capable, and various technological advancements we take for granted wouldn't exist.
Historical Context
The story of semiconductors is one of innovation and perseverance. The roots can be traced back to the early 20th century when scientists like Alan Noble and John Bardeen began exploring the properties of materials like silicon and germanium. The invention of the transistor in 1947 by Bardeen and his colleagues at Bell Labs marked a turning point. Prior to this, electronic devices relied on vacuum tubes, which were large, inefficient, and often unreliable.
The transition from these bulky tubes to transistors ignited a revolution in electronics. With transistors being smaller, more reliable, and cheaper to produce, they paved the way for smaller and more efficient devices. The subsequent introduction of integrated circuits in the 1960s further accelerated this trend, leading to the miniaturization of electronic components and kick-starting the age of digital computing.
As the years passed, semiconductor technology continued to evolve, with the development of materials and techniques that enhance performance. The journey from early laboratory experiments to today's sophisticated semiconductor technology is a testament to human ingenuity and has set the stage for future innovations.
"Without semiconductors, the digital age as we know it would not exist."
Fundamental Principles of Semiconductor Physics
Understanding the fundamental principles of semiconductor physics is critical for delving into the inner workings of semiconductor devices. This segment lays the groundwork for comprehending how these devices function, influencing everything from consumer electronics to advanced computational technologies. Moreover, grasping these principles paves the way for innovations in the field, making it pertinent for students, researchers, and industry professionals alike.
Intrinsic vs. Extrinsic Semiconductors
When discussing semiconductors, one must first consider the distinction between intrinsic and extrinsic types.
- Intrinsic Semiconductors: These are pure materials that exhibit semiconductor properties without any added impurities. Silicon and germanium are classic examples. Their properties are dictated solely by the atomic structure and behavior of electrons within the crystal lattice. In an intrinsic semiconductor, the conduction of electricity arises from the thermal energy that excites electrons, allowing them to jump across the band gap. This creates holes which can also contribute to current flow.
- Extrinsic Semiconductors: In contrast, extrinsic semiconductors have been doped with impurities to enhance their conductive properties. By introducing elements like phosphorus or boron into the silicon lattice, the electronic properties can be finely tuned.
- N-Type Semiconductor: When the dopant has more valence electrons (like phosphorus), it provides extra electrons for conduction, making the material negatively charged.
- P-Type Semiconductor: Conversely, if the dopant has fewer valence electrons (like boron), it creates holes, resulting in a positively charged material.
Understanding these types is pivotal as they come into play in various devices including diodes and transistors, which are foundational in electronics. Each type brings unique characteristics to the table, allowing for tailored electrical behaviors based on specific application requirements.
Band Theory and Energy Bands
Band theory offers a framework to understand the electronic properties of solids, particularly how semiconductors conduct electricity. This concept revolves around the notion of energy bands â the ranges of energy that electrons may occupy in a solid.
- Valence Band and Conduction Band: In semiconductors, electrons are situated in the valence band at lower energy levels. When sufficient energy is provided, they can leap into the conduction band, where they can move freely and contribute to conductivity.
- Band Gap: The band gap is a crucial factor in this context. It is the energy difference between the conduction band and the valence band. In intrinsic semiconductors, the band gap is small enough that thermal energy can promote electrons across it. However, in extrinsic semiconductors, doping modifies the band structure, enhancing conductivity.
Utilizing the band theory enables engineers to design materials with specific electronic properties, optimizing them for various applications. The manipulation of the band gaps through doping techniques is what fuels the advancement of modern electronics, allowing for the development of highly efficient devices.
Understanding the distinctions between intrinsic and extrinsic semiconductors and the implications of band theory underpins much of modern semiconductor technology.
In summary, the principles of semiconductor physics are not only foundational but essential for navigating the evolving landscape of technology. As advancements continue, comprehensively grasping these principles will determine the efficacy and innovation of future developments in semiconductor devices.
Classification of Semiconductor Devices
Understanding the classification of semiconductor devices is crucial because it sets the stage for grasping how these devices function and where they are applied in real-world scenarios. Each type of semiconductor device has unique characteristics, operational mechanisms, and applications that cater to different technological needs. This classification not only helps students and professionals navigate through the semiconductor landscape but also highlights the diverse innovations that stem from these devices.
Diodes
Diodes are among the simplest types of semiconductor devices. They essentially act as one-way streets for electric current, allowing it to flow in only one direction. Their fundamental operation relies on the P-N junction, a boundary created between P-type and N-type semiconductors. This interface is where charge carriersâholes and electronsâcombine to produce a depletion region, inhibiting current flow under reverse bias and permitting it under forward bias. As a result, diodes are widely utilized in rectification purposes where alternating current must be converted to direct current.
The importance of diodes extends beyond traditional power supply applications. They play a pivotal role in signal demodulation, voltage regulation, and even in the functioning of essential circuits like Zener and Schottky diodes. Given their varietyâeach serving unique purposesâdiodes are indispensable in modern electronics.
"Without diodes, the modern electronic world we know today would not exist; they are the unsung heroes in every circuit."
Transistors
Transistors, originally developed in the mid-20th century, ushered in a new era in electronics. They function as switches or amplifiers, enabling the control of electrical signals. With three terminalsâemitter, base, and collectorâa transistor can manipulate the flow of current based on the input signal at the base. The ability to switch states rapidly makes transistors integral components in digital circuits, especially in computers and smartphones.
Transistors can be further divided into two main categories: bipolar junction transistors (BJTs) and field-effect transistors (FETs). BJTs utilize both electron and hole charge carriers, while FETs rely primarily on electron movement for switching, offering higher input impedance and better efficiency in many applications.
The concept of integrating multiple transistors on a single chip has given rise to integrated circuits, pushing the envelope of what semiconductor technology can achieve. This integration amplifies the computing speed and reduces power consumption, making transistors not just pivotal but revolutionary in the world of technology.
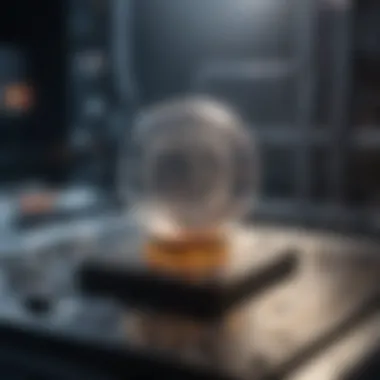
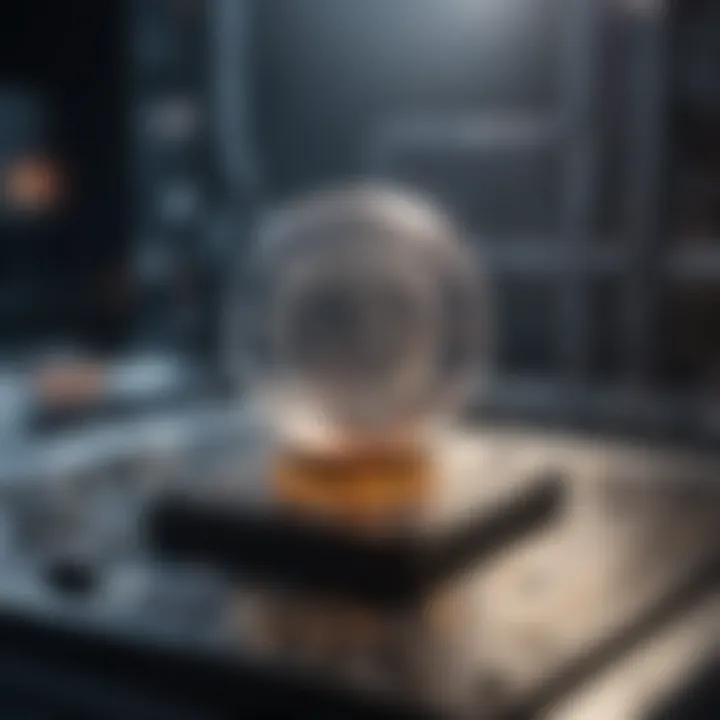
Integrated Circuits
Integrated circuits, also known as ICs, represent the pinnacle of semiconductor advancements. They combine numerous transistors, diodes, resistors, and capacitors into a single chip, significantly enhancing functionality while minimizing size. This packaging is not just about saving space; it also improves performance and reduces manufacturing costs.
The invention of integrated circuits has led to miniaturization in electronics, something that has transformed everything from consumer gadgets to space exploration equipment. They serve a host of applications, including microprocessors in computers, signal processing chips in audio systems, and sensors in smartphones.
In summary, the classification of semiconductor devices into diodes, transistors, and integrated circuits is not merely theoretical but practical. Each type serves a unique purpose and contributes to the vast landscape of technological applications, playing a crucial role in the evolution and future advancements in electronics.
Materials Used in Semiconductor Devices
The choice of materials in semiconductor devices is paramount, as it has a direct impact on performance, efficiency, and applicability across various technologies. In todayâs rapidly evolving electronics landscape, understanding the materials used goes beyond just identifying them; it involves recognizing their distinct properties, the advantages they bring to the table, and the limitations they might pose. Choosing the right material can make or break an electronic device. The two most pivotal players in the semiconductor gameâsilicon and gallium arsenideâstand as testaments to this.
Silicon: The Dominant Material
Silicon has been the cornerstone of the semiconductor industry for decades. Its significance canât be overstated; about 90% of semiconductor devices are made from silicon. The very tune of our electronic world strikes the chords of siliconâwith its abundant supply, cost-effectiveness, and stable electrical characteristics making it an obvious pick.
One of silicon's unique attributes is its capability to form a protective layer of silicon dioxide, which is essential in the manufacturing process. This property allows for better isolation and protects other components from contamination and external interference. Notably, the extensive research and development surrounding silicon have honed its functionality, continually improving its efficiency in diverse applications.
Moreover, siliconâs ease of doping has led to a diversification of its use in integrated circuits. By adjusting the concentration of impurities, silicon can be turned into n-type or p-type semiconductors suitable for various applications. Such adaptability has enabled a wide range of electronic devicesâfrom simple diodes to complex microprocessors.
"Silicon's versatility and cost-effectiveness have made it the backbone of everything from smartphones to supercomputers."
Gallium Arsenide and Other Alternatives
While silicon takes the crown, gallium arsenide is quickly gaining traction, especially in high-frequency and optoelectronic applications. Unlike silicon, gallium arsenide has a direct band gap, allowing it to emit light efficiently. This makes it a go-to for producing lasers and light-emitting diodes. The efficiency at which gallium arsenide operates at high frequencies makes it vital for communications equipmentâthink of your smartphoneâs network connectivity.
In addition to gallium arsenide, there are other materials, although not as mainstream yet, that show promising characteristics:
- Indium Phosphide: Favored in high-speed applications, itâs prevalent in fiber-optic devices due to its high electron mobility.
- Silicon Carbide: Known for its high thermal conductivity and electric field strength, making it ideal for high-power applications.
- Organic Semiconductors: Lighter on the wallet and more versatile in applications like flexible electronics and displays.
Each alternative has its own set of advantagesâsome may boast higher electron mobility, while others might be more suitable for specific applications. However, they often come with trade-offs in manufacturing complexity and cost.
Thus, understanding the material landscape is essential for design considerations in semiconductor devices, unveiling the various layers of technology that dictate how these components shape the electronics of tomorrow.
Manufacturing Processes of Semiconductor Devices
The manufacturing processes of semiconductor devices are the backbone of the entire semiconductor industry. These processes transform raw materials into, well, the chips that power your gadgets, control your household items, and even guide autonomous vehicles. Understanding how these processes work is not just an academic exercise; it's crucial for realizing the efficiency, performance, and potential errors that can arise in semiconductor fabrication.
Crystal Growth Techniques
Crystal growth is a foundational step in semiconductor manufacturing. The most commonly used method is the Czochralski process, where a seed crystal is dipped into molten silicon and slowly pulled upwards, forming a single crystal. This method is vital because the quality of the crystal affects the electrical properties of the device.
There are several key aspects to consider:
- Purity: The silicon used must be extremely pure, often reaching levels of 99.9999999% (commonly referred to as 9N purity). Impurities can alter the electrical characteristics of the semiconductor, leading to reliability issues.
- Orientation: The orientation of the crystal can greatly influence the electronic properties. Different orientations can affect how carriers move, akin to how roads influence traffic flow.
- Crystal defects: Any imperfections in the crystal lattice may lead to issues in device performance. These defects can come from the crystal growth process itself or from external factors like temperature fluctuations.
This technique isn't merely about growing a chunk of crystal; itâs about creating something that can work effectively under various conditions and demands. The dominance of silicon in the semiconductor world is no accident; its advantageous electronic properties owe a great deal to how these crystals are grown.
Photolithography and Etching
Once the crystal ingots are produced, the next phase is where the really detailed work beginsâphotolithography and etching. This process is akin to putting a stencil on an art piece; you want to make sure that the structure and features are precise.
Photolithography involves coating a wafer with a light-sensitive photoresist. Using a mask, ultraviolet light exposes specific areas of the resist:
- Exposure: The areas exposed to light become either soluble or insoluble, depending on whether a positive or negative photoresist is used.
- Development: The wafer is then developed, washing away the soluble areas and leaving behind a pattern that resembles the design on the mask.
Following this, we move on to etching:
- Dry Etching: This technique uses plasma to remove unwanted material, leaving the desired pattern intact. Itâs highly controlled and mostly used for fine structures.
- Wet Etching: This is a simpler method involving chemical solutions. It is especially effective for bulk removal but is less precise than dry etching.
Together, photolithography and etching are instrumental in creating the intricate designs of circuits. They allow for the micro-manufacturing of components with dimensions that reach into the nanoscale, pushing boundaries that were unimaginable just a few decades ago.
In summary, understanding these manufacturing processes highlights the delicate balance between art and science in semiconductor production. They are not just steps in production but are the very fundamental techniques that shape the future of technology. As we navigate challenges in material limitations and environmental concerns, refining these processes will be key to advancing semiconductor technology further.
Operational Mechanisms
In semiconductor devices, understanding operational mechanisms is key to grasping how these components facilitate current flow and signal processing. The intricate dance of electrons and holes, along with various physical phenomena, forms the backbone of these devices. This section digs into critical elements, benefits, and considerations of operational mechanisms, focusing primarily on two significant aspects: current flow and charge carriers, alongside the p-n junction theory.
Current Flow and Charge Carriers
At the essence of semiconductor function lies the concept of current flow, largely governed by charge carriers. The two primary types of charge carriers are electrons and holes. Electrons, possessing a negative charge, contribute to conductivity when they move through the material. Conversely, holes represent the absence of electrons and behave as positive charge carriers. This duality allows semiconductor materials to be manipulated in ways that metals cannot.
For instance, in an n-type semiconductor, where extra electrons are introduced via doping with elements like phosphorus, the mobility of these electrons enhances conductivity. On the other hand, p-type semiconductors, which are doped with materials such as boron, create an abundance of holes that also facilitate current flow through the positive charge movement.
"The unique ability to control current flow through charge carriers is what sets semiconductors apart from traditional conductive materials."
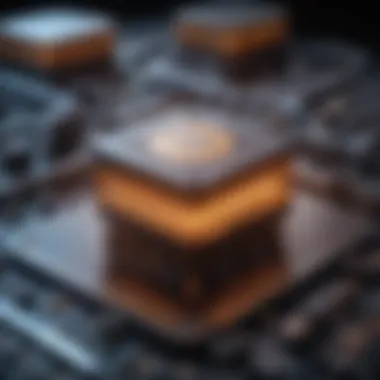
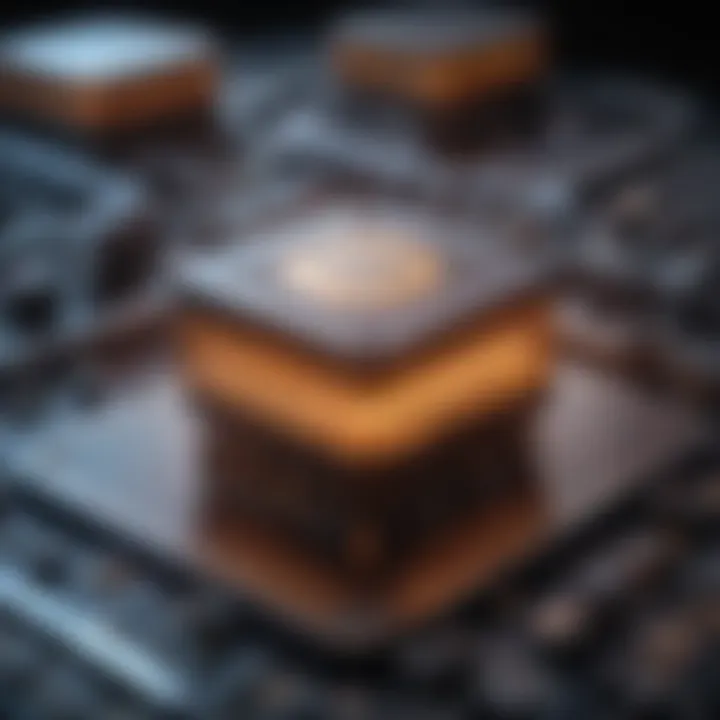
Understanding the dynamics of these charge carriers is critical for applications in electronic circuits. For example, when a voltage is applied across a semiconductor, electrons will flow toward the positive terminal, while holes will move toward the negative terminal. This behavior is vital in the functionality of diodes and transistors, underpinning their operation in various electronic devices.
Moreover, the temperature of the semiconductor affects the mobility and availability of these charge carriers. As temperature increases, more electrons gain enough energy to break free from their bonds, thus increasing the conductivity of the material. This characteristic exemplifies the temperature-sensitivity of semiconductor devices, a consideration that impacts performance in real-world applications, particularly in environments with fluctuating temperatures.
P-N Junction Theory
The p-n junction forms the heart of many semiconductor devices, acting as a barrier that governs current flow. This junction is created by joining p-type and n-type materials, leading to a fascinating interaction at the boundary. When these two types of semiconductors come into contact, electrons from the n-side migrate into the p-side, while holes from the p-side move toward the n-side. This movement results in the formation of a depletion region where the charge carriers cancel each other out, creating an electric field that opposes further movement.
This fascinating mechanism has several benefits. For one, it allows the p-n junction to act as a diode, permitting current flow in one direction while blocking it in the opposite direction. Likewise, when a forward voltage is applied, the electric field is dismantled, allowing charge carriers to recombine and current to swiftly flow through the junction. Conversely, applying a reverse voltage strengthens the electric field, preventing current from flowing.
Understanding p-n junction behavior is crucial for both students and professionals engaged in electronics. It serves as a fundamental principle for how diodes, transistors, and many other devices operate. The linear and nonlinear characteristics of a p-n junction can be graphically represented using current-voltage (I-V) curves, showcasing the relationship between voltage applied across the junction and the resultant current passing through it. This theoretical framework shows how semiconductor devices efficiently manage electrical energy.
As we continue to explore the diverse applications and implications of these mechanisms, it's evident that they are integral not just to semiconductor physics, but to the overall advancement of technology in our modern society.
Applications of Semiconductor Devices
The application of semiconductor devices stands as a pillar supporting numerous sectors of modern technology. These devices are not only integral to the functionality of electronic components but drive innovations across various industries. The importance of studying these applications in semiconductor devices arises from their ability to enhance performance, efficiency, and reliability in technology. With a more profound understanding of how these applications operate, one can appreciate the transformative impact semiconductor technology has had on our daily lives.
Consumer Electronics
In the realm of consumer electronics, semiconductor devices are the unsung heroes powering a significant portion of the devices we take for granted. Smartphones, laptops, tablets, and smart home gadgets all leverage these technologies to operate seamlessly. Here are some specific roles semiconductor devices play in consumer electronics:
- Microprocessors: These tiny powerhouses manage the computing tasks and operate user-interaction features that define modern gadgets. The speed, size, and efficiency of microprocessors have grown tremendously due to advancements in semiconductor materials and manufacturing techniques.
- Memory Chips: DRAM and NAND flash memories are fundamental for data storage in devices, allowing users significant capacity without bulking up devices. The speed with which these memories can read or write data has a direct impact on overall device performance.
- Display Technology: Semiconductors are crucial for LCD, LED, and OLED technologies in displays, providing better color accuracy, energy efficiency, and thinner screens. This has transformed the way we consume media, enhancing experiences in gaming and streaming.
Telecommunications
The telecommunications sector heavily relies on semiconductor devices to maintain and expand its networks. From mobile phones to the infrastructure supporting global communication, the adaptability and performance of these devices are vital:
- Signal Processing: Semiconductors are utilized in signal processors, assisting in the encoding, decoding, and filtering of signals. This ensures clearer communication over long distances, minimizing loss and distortion.
- Power Amplifiers: These devices enhance the range and clarity of signals transmitted through antennas. The efficiencies gained from newer semiconductor materials like GaN (Gallium Nitride) allow for more compact and efficient designs, enabling high performance from smaller setups.
- Fiber Optics: With light becoming the information carrier, semiconductor technology has pushed the development of optoelectronic devices which transform electrical signals into optical ones and vice versa. This has opened doors for faster and more reliable internet connections, essential in today's data-driven world.
Medical Devices
In the medical field, semiconductor devices help bridge technology and healthcare, promoting innovative solutions that enhance patient care and diagnostics. Their applications include:
- Diagnostic Equipment: Semiconductor sensors play a pivotal role in imaging devices like MRIs and CT scanners. They enhance image resolution and clarity while allowing for more rapid diagnosis which is crucial in emergency situations.
- Wearable Technology: Devices like heart rate monitors, glucose trackers, and fitness trackers rely on semiconductor technology to gather, analyze, and wirelessly transmit health data. This data can assist healthcare providers in making timely decisions regarding patient care.
- Therapeutics and Actuation Devices: Semiconductor components control drug delivery systems and robotic surgical devices, improving accuracy and outcomes in treatments. The miniaturization trend allows these devices to be integrated into smaller, less invasive formats, benefitting patient experiences.
"The merging of semiconductor technology with healthcare not only revolutionizes treatment methodologies but also reminds us of the potentially life-saving consequences of these devices."
As we've seen, semiconductor devices are indeed everywhere, with critical roles in consumer electronics, telecommunications, and medical applications. Each sector benefits from the advancement of semiconductor technology, enhancing performance, reliability, and innovation. Understanding these applications not only enlightens our perception of technology but also emphasizes the continuous need for development in semiconductor devices.
Emerging Trends in Semiconductor Technology
The landscape of semiconductor technology is ever-evolving, driven by the constant demand for more efficient, powerful, and versatile devices. Understanding these emerging trends is crucial for academics and professionals alike, as they hold the key to future innovations and breakthroughs. In this section, we will delve into the latest advancements, chiefly focusing on quantum dots, nanoelectronics, and organic semiconductors. The significance of these trends cannot be overstated; they are reshaping industries and laying the groundwork for the next generation of electronic devices.
Quantum Dots and Nanoelectronics
Quantum dots represent a striking innovation in semiconductor technology. These nanoscale particles, which are just a few nanometers in size, exhibit unique optical and electronic properties that differ significantly from bulk materials. One of their most notable features is quantum confinement, whereby the electronic properties alter based on their size and shape. This variability opens the door to an array of applications, from more efficient solar cells to advanced display technologies.
In electronics, quantum dots are being explored for their potential to revolutionize transistors. Traditional transistors have limitations in terms of speed and power consumption. However, by utilizing quantum-dot-based transistors, researchers are hopeful to achieve faster operation with lower energy usage. This energy efficiency is particularly important in the context of sustainable technology, where reducing power consumption is becoming a priority.
Moreover, quantum dots are also making waves in the field of imaging technologies. They are used in biological probes that improve the imaging quality in medical diagnostics, enhancing both resolution and efficiency.
The benefits of integrating quantum dots into semiconductor devices extend beyond performance. They also promise cost reduction due to their potential scalability in manufacturing processes.
Organic Semiconductors
Organic semiconductors, made up of carbon-based materials, are emerging as a game-changer in the semiconductor scene. Unlike traditional silicon-based materials, organic semiconductors boast unique benefits, such as flexibility, lightweight properties, and the possibility for low-cost production. This flexibility is especially desirable for applications in wearable technology and flexible displays, where conventional materials fall short.
The operational mechanism of organic semiconductors revolves around their ability to transport charge carriers effectively. Though they have traditionally faced challenges regarding stability and efficiency compared to their conventional counterparts, recent advancements have significantly improved their performance. For instance, organic light-emitting diodes (OLEDs) are now commonly used in screens for televisions and mobile devices, providing better color reproduction and contrast ratios than many traditional displays.
Additionally, organic photovoltaics, a form of solar energy technology, are gaining attention for their potential to create lightweight and flexible solar panels. Although still in the developmental stage, these products show promise for wider application in diverse environments.
In summary, both quantum dots and organic semiconductors are pushing the boundaries of semiconductor technology, encouraging novel innovations and addressing the pressing needs of modern electronic devices. As these technologies progress, their implications span across various sectors, shaping the future of electronics in ways previously thought unattainable.
"The future belongs to those who believe in the beauty of their dreams."
Adopting these emerging trends not only cultivates a more resilient semiconductor industry but also propels advancements that hold the potential for transformative impacts on society and everyday life.
The Role of Semiconductors in Future Innovations
Semiconductors form the backbone of modern technology, playing a pivotal role in innovations that are set to shape our future. Their versatility enables countless applications across varying fields, such as artificial intelligence and renewable energy. Understanding their significance is crucial to grasping how advancements in these areas can drive societal progress.
This section encapsulates two crucial aspects of semiconductor technology's future â integration with AI and machine learning, and advancements in renewable energy technologies. Both topics highlight not only the capabilities of semiconductor devices but also their transformative potential.
Integration with AI and Machine Learning
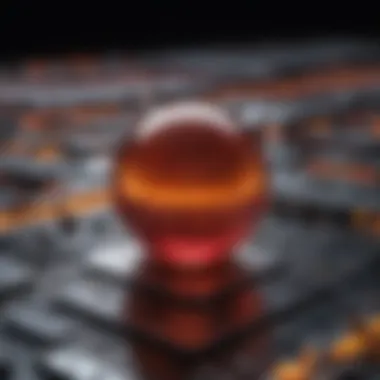
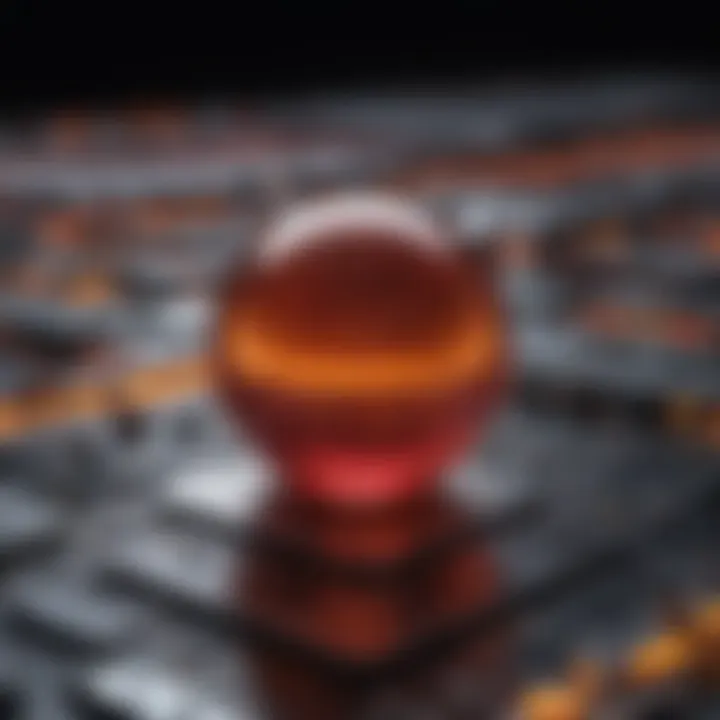
Artificial intelligence has taken the world by storm, and semiconductors are right at the heart of this technological revolution. The exponential growth of AI demands not only faster processing powers but also efficient energy consumption. Here, semiconductors like GPUs (Graphics Processing Units) and TPUs (Tensor Processing Units) come into play. These chips are specifically designed to handle complex calculations and vast datasets, enabling machines to learn and adapt in real-time.
Key elements of this integration include:
- Enhanced Processing Capabilities: The architecture of modern semiconductors allows for parallel processing, which accelerates the execution of AI algorithms significantly.
- Energy Efficiency: Power consumption is a growing concern in technology. Advanced semiconductors are engineered to deliver higher performance while using less energy.
- Miniaturization: As demands for compact devices grow, smaller, yet more powerful semiconductor technologies are emerging. Compactness is vital in creating AI-driven devices that can be easily integrated into everyday life, from smart assistants to autonomous vehicles.
The future looks bright with the ongoing developments in this sector. The semiconductor industry is advancing rapidly, paving the way for breakthroughs in machine learning algorithms and enhancing how these systems perceive and react to their environments.
Advancements in Renewable Energy Technologies
Another arena where semiconductors play a crucial role is in renewable energy technologies. As society seeks cleaner, sustainable energy solutions, semiconductors are becoming central to the development and deployment of solar panels, wind turbines, and energy storage systems.
Several important considerations include:
- Efficiency Improvements: High-efficiency solar cells utilize advanced semiconductor materials, such as perovskite and silicon, to convert sunlight into energy more effectively.
- Smart Grids: Semiconductors are essential in the sensors and controls that regulate power distribution and usage in smart grids, maximizing efficiency and reliability.
- Energy Storage Solutions: Cutting-edge semiconductor materials enhance the performance of batteries and supercapacitors, ensuring that renewable energy can be stored efficiently and utilized when needed.
"The advancements in semiconductor technology are essential for meeting global energy demands sustainably."
The integration of semiconductors into renewable energy technologies signifies a large step towards reducing our carbon footprint while addressing the world's growing energy needs. As the world increasingly shifts towards sustainability, semiconductors will remain indispensable in facilitating this change, enabling innovations that harness natural resources.
Understanding the role of semiconductors in these future innovations helps appreciate their broader impact on technology, society, and the environment. The ongoing evolution in this sector is not merely incremental; rather, it is a significant leap towards a smarter, greener future.
Challenges in Semiconductor Manufacturing
The semiconductor manufacturing landscape is complex and ever-changing, often presenting distinct challenges that can hinder progress and innovation. These hurdles are not merely operational inconveniences; they have far-reaching implications for the entire industry. Understanding these challenges is fundamental in realizing the future potential of semiconductor technologies. It sheds light on areas needing improvement or innovation, and points towards strategic directions for research and development.
Material Limitations
Materials science plays a critical role in semiconductor manufacturing. The choice of materials impacts the performance, durability, and efficiency of devices. However, certain limitations persist:
- Scarcity of high-quality materials: High-purity silicon is the backbone of most semiconductor devices, yet sourcing it can be a challenge due to environmental regulations and market dynamics. Other materials, like gallium arsenide, are often hard to find and more expensive, putting a strain on production costs.
- Variability in material properties: Impurities or defects in materials can lead to inconsistencies in device performance. Quality control becomes paramount, but ensuring that every batch meets exacting standards can be resource-intensive.
- Evolving requirements: As technology progresses, materials must evolve as well. Devices are getting smaller and more powerful, requiring new materials that can withstand increased operational stresses. This not only demands innovation but also incurs significant research costs and time.
"Material limitations foster a constant race for better alternatives, forcing manufacturers to innovate or risk obsolescence."
Environmental Concerns
The semiconductor industry is also under scrutiny for its environmental impact. The manufacturing processes often involve hazardous chemicals and substantial energy consumption. Consider these aspects:
- Chemical waste management: The production phase generates a considerable amount of waste, including toxic by-products that require careful disposal to prevent environmental contamination. Ensuring compliance with environmental regulations adds another layer of complexity.
- Energy consumption: Semiconductor fabrication plants, commonly known as fabs, require massive amounts of energy to operate. As the world pushes toward sustainability, the industry faces pressure to lower its energy footprint. This not only relates to direct energy usage but also encompasses the entire supply chain.
- Water usage: Water is vital in the cleaning processes during fabrication. Efficient water management is crucial, especially in regions experiencing drought or water scarcity.
In summary, addressing the challenges in semiconductor manufacturing is not just about overcoming operational kinksâthese are critical to the sustainability and future readiness of the industry. The balance between innovation, efficiency, and environmental responsibility must be achieved for the sector to thrive in the coming years.
Future Prospects of Semiconductor Devices
The semiconductor landscape is continuously reshaping itself, reflecting the ever-evolving technologies and consumer demands. This section delves into what lies ahead for semiconductor devices, highlighting the importance of understanding trends and potential avenues for growth. Itâs not just about keeping pace; itâs about anticipating the future and preparing for the waves of change.
Trends in Miniaturization
Miniaturization has been a hallmark in semiconductor fabrication, opening doors to both performance improvements and enhanced energy efficiencies. The relentless drive for smaller, faster, and cheaper devices has propelled researchers and engineers to explore every nook and cranny of innovation. In recent years, two significant aspects have come to the forefront:
- Transistor Scaling: Integrated circuits are essentially packs of transistors. The ability to fit more transistors into a single chip has been a game changer. Each new technology node, such as 5nm or 3nm processes, brings significant advantages. More transistors mean more processing power and efficiency. However, as we approach atomic scales, managing heat and quantum effects becomes increasingly tricky. It can be likened to trying to fit an elephant into a phone boothâchallenging but incredibly rewarding when done right.
- Advanced Packaging Techniques: Beyond simply shrinking chips, advancements in how these devices are packaged are enhancing performance. Technologies like 3D stacking allow chips to be layered vertically, which further saves space and optimizes performance. By thinking outside the boxâliterallyâresearchers are finding innovative solutions to traditional hurdles.
Potential Markets for Growth
The future is ripe with opportunities for semiconductor advancements, particularly in sectors poised for exponential growth. Here are a few key areas to watch closely:
- Electric Vehicles: With the global push towards sustainability, electric vehicles are on the upswing. They rely heavily on semiconductor technology for battery management systems, power conversion, and system integration. As automakers commit to electrification, the demand for robust semiconductor solutions will soar.
- Artificial Intelligence: AI and machine learning applications are becoming ubiquitous across industries. Specialized chips designed for AI tasks, like tensor processing units (TPUs), are gaining traction. Companies that recognize the demand for efficient AI processing capabilities will be critical players in the semiconductor realm.
- Telecommunication Networks: The advent of 5G networks necessitates an upgrade in semiconductor technology. High-frequency devices that can handle the increased data rates and improved connectivity will become essential. This creates a booming market for firms that can innovate rapidly.
"The semiconductor industry stands on the brink of opportunities that could redefine technology as we know it."
- Internet of Things (IoT): With the proliferation of smart devices, IoT applications are continuously expanding. Each sensor and smart gadget that connects needs efficient semiconductor solutions. From smart home devices to industrial IoT applications, the sky's the limit.
Epilogue
Reflecting on the importance of semiconductor devices allows us to understand not only the present landscape of technology but also the future that lies ahead. In this article, weâve taken a thorough journey from defining what these devices are to delving into their significance across various sectors. We explored their historical evolution, highlighted the types and materials used, and examined the complex manufacturing processes that bring them into existence. Ultimately, these devices are the unsung heroes behind modern electronics and technology.
Summary of Key Points
In summary, several critical elements contribute to the overarching importance of semiconductor devices:
- Foundation of Modern Electronics: They serve as the core component in numerous electronic products, ranging from simple home appliances to complex computer systems.
- Wide Applicability: With their diverse applications in consumer electronics, telecommunications, and medical devices, semiconductors have permeated many facets of our daily lives.
- Technological Advancements: Ongoing development in this field, including quantum dots and organic semiconductors, paves the way for new innovations, enabling possibilities previously deemed impossible.
- Future Growth: Understanding trends like miniaturization and the increasing integration with artificial intelligence is crucial for grasping how the semiconductor landscape will evolve.
Final Thoughts on the Semiconductor Landscape
The semiconductor landscape is not merely about the devices themselves but encompasses a warp of intersecting technologies and concepts. As we gaze into the horizon, several considerations arise:
- Sustainability: The environmental footprint of semiconductor manufacturing poses challenges that necessitate innovations not only in the devices themselves but also in the production processes.
- Global Supply Chain Dynamics: Recent disruptions highlighted how global dependencies can impact production and development in semiconductors. The need for more localized production and resilience in supply chains has never been clearer.
- Research and Development: Continuous investment in R&D remains vital for pushing the boundaries. New materials and methods need to be explored to maintain pace with consumer and technological demands.
"Semiconductors are not just components; they embody the potential of technology itself, influencing everything from how we communicate to how we approach energy solutions."
In closing, understanding the multifaceted nature of semiconductor devices allows students, researchers, educators, and professionals not only to appreciate their current applications but to envision the frameworks for future advancements in a world increasingly reliant on technology.