Exploring Saltwater Direct: Principles and Impact
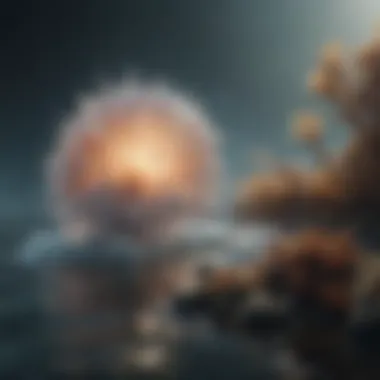
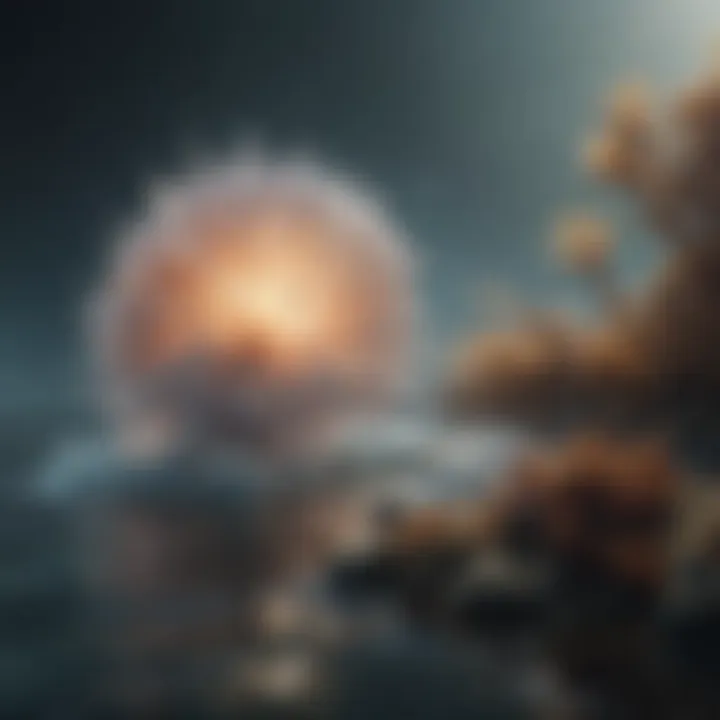
Intro
Saltwater direct refers to the innovative and strategic use of saltwater across various fields, creating a new paradigm in resource management. Traditionally, saltwater has been viewed primarily as a waste product or a challenging environmental factor in fresh water systems. However, recent advancements in technology and research have paved the way for utilizing saltwater in productive ways that address pressing global challenges. This article seeks to explore the fundamental principles behind saltwater direct, its applications across different sectors, and its environmental implications.
Overview of Research Topic
Brief Background and Context
The concept of saltwater direct is rooted in the need for sustainable resource management. With an increasing population and dwindling freshwater supplies, the exploration of saltwater as a key resource becomes imperative. This is especially true in coastal regions, where saltwater is abundantly available. Researchers and practitioners are investigating methods to harness saltwater for agricultural irrigation, energy production, and even in industrial processes.
Importance in Current Scientific Landscape
Understanding saltwater direct is crucial for various reasons:
- Resource Scarcity: As fresh water becomes scarce, utilizing saltwater can mitigate water shortages.
- Sustainability Goals: Saltwater use aligns with global sustainability objectives, aiding in reducing agricultural water stress and reliance on freshwater resources.
- Technological Advancements: Innovations such as desalination and salt-tolerant crops are becoming viable and showcase the potential of saltwater in sustaining food and energy production.
The relevance of this topic cannot be overstated, considering the environmental challenges that demand urgent attention, such as climate change and population growth. Saltwater direct presents a multifaceted solution that holds promise for future generations.
Methodology
Research Design and Approach
To understand the principles and applications of saltwater direct, the research adopts a systematic literature review approach. This involves compiling existing studies, reports, and case analyses that focus on saltwater applications in various domains. A detailed examination of the data helps in identifying gaps in knowledge and uncovering innovative practices.
Data Collection Techniques
The data collection focuses on multiple sources:
- Academic Journals: Articles that provide insight into experimental and theoretical studies of saltwater usage.
- Government Reports: Documents detailing policies and initiatives regarding resource management.
- Case Studies: Real-world applications in agriculture and energy production, illustrating practical uses of saltwater direct.
This blend of qualitative and quantitative data ensures a robust understanding of the topic, revealing trends and advancements in saltwater utilization.
Prelude to Saltwater Direct
Understanding saltwater direct is crucial for grasping how this concept intertwines with many modern challenges. As freshwater resources dwindle due to population growth and climate change, the focus on saltwater utilization becomes even more significant. This article aims to elucidate the relevance of saltwater direct in diverse applications ranging from engineering to energy generation.
Saltwater direct encompasses processes and methodologies that leverage saltwater in practical and sustainable ways. The benefits include enhancing water security in arid regions and providing alternative resources for industries. Moreover, its integration into agriculture and energy sectors highlights its versatility and importance.
Definition of Saltwater Direct
Saltwater direct refers to the direct use of saltwater in various applications without prior desalination. This concept encompasses a spectrum of processes aimed at utilizing saline waters effectively. It can include methods such as saline irrigation in agriculture, where saline water is used for crop production, or the application of saltwater in energy generation through salinity gradient methods. The term captures both the resource and the innovative approaches to apply it.
A key aspect of saltwater direct is its ability to enhance sustainability. Using saltwater for agricultural practices can prevent the over-extraction of freshwater, preserving vital ecosystems. Additionally, the energy sector benefits from saltwater through technologies like salinity gradient power, showcasing the dual benefits of resource conservation and energy generation.
Historical Context
The utilization of saltwater is not a modern innovation; it has roots in ancient practices. Early civilizations often relied on coastal waters for fishing and trade, but the direct application of saltwater in agriculture and industry evolved much later. In the mid-20th century, with the rise of environmental concerns and resource scarcity, interest in saltwater application began to grow.
Innovations such as drip irrigation using saline water have emerged from researched efficiencies. For agriculturalists, particularly in arid regions, the historical journey of using saltwater reveals a changing perception toward saline environments. As technology advances, the integration of saltwater is becoming a focal point in resource management and sustainable development strategies.
"Utilizing saltwater direct not only marks a shift towards sustainability but also showcases human ingenuity in overcoming resource limitations."
In sum, the definition and historical context lay a solid foundation for discussing the broader implications and applications of saltwater direct. As the stakes rise in resource management, the examination of saltwater's role remains pertinent and compelling.
Scientific Principles
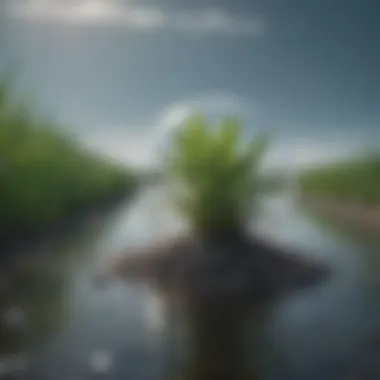
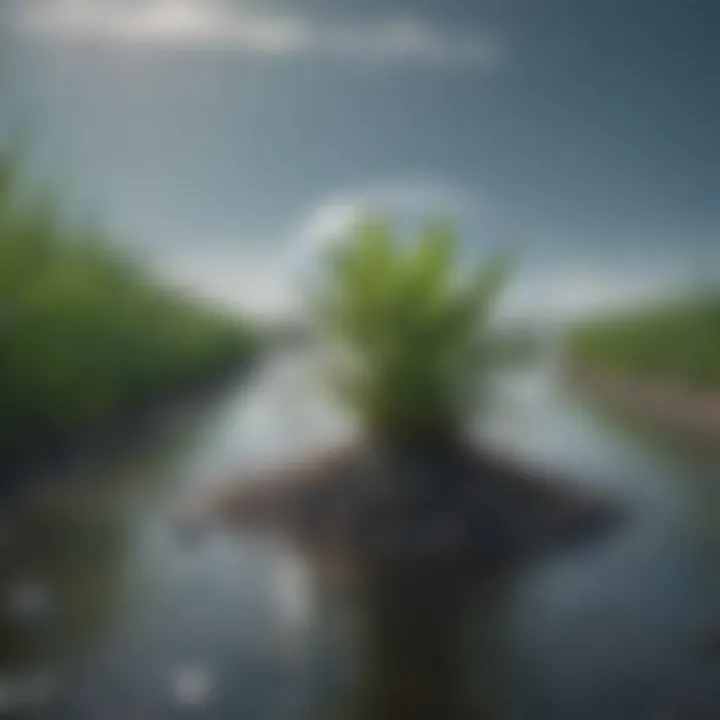
The scientific principles surrounding saltwater direct are foundational for understanding its diverse applications. These principles not only clarify how saltwater functions in various contexts but also highlight its potential benefits and limitations. By delving into the chemical aspects, physical properties, and thermodynamic behavior, we gain critical insights regarding both the possibilities and challenges of utilizing saltwater effectively.
Chemical Composition of Saltwater
Saltwater primarily consists of water and dissolved salts. The most prevalent salt is sodium chloride, commonly known as table salt. However, the composition varies based on location and the source of the water. Other ions such as magnesium, potassium, and sulfate can also be found in significant concentrations.
Understanding the chemical composition is crucial for several reasons:
- Desalination Processes: Different chemicals influence the efficiency of desalination techniques, such as reverse osmosis and multi-stage flash distillation.
- Agricultural Applications: The nutrient content from salts can impact crop growth, making it necessary to know the specific chemical makeup.
- Water Quality Assessment: The balance of chemicals determines the suitability of saltwater for various uses.
Physical Properties
The physical properties of saltwater are distinct from those of fresh water. For example, saltwater has a higher density and boiling point than freshwater, due to the presence of dissolved salts. These properties affect many applications, including:
- Buoyancy: Objects float more easily in saltwater, influencing maritime engineering.
- Temperature Regulation: Saltwater can retain heat better, which plays a role in climate dynamics and energy generation.
- Solubility Dynamics: The presence of salts alters how substances dissolve in water, impacting chemical reactions.
The importance of understanding these properties cannot be overstated, as they dictate the feasibility of utilizing saltwater in specific contexts.
Thermodynamics Involved
Thermodynamics plays a pivotal role in the behavior of saltwater systems. This field of study focuses on heat transfer and energy transformations. In the context of saltwater, several thermodynamic principles are key:
- Phase Changes: Saltwater does not freeze or boil at the same temperatures as freshwater. The presence of salt lowers the freezing point and raises the boiling point. This knowledge is crucial for processes like desalination.
- Energy Requirements: Different desalination techniques require varying amounts of energy. Being aware of these requirements enables developers to choose more efficient methods based on their specific needs.
- Entropy Considerations: Saltwater systems often exhibit unique entropy characteristics, which can affect the feasibility of various processes.
By grasping these thermodynamic aspects, it becomes easier to optimize processes involving saltwater, from energy generation to water purification.
Applications of Saltwater Direct
The applications of saltwater direct encompass converging fields crucial to modern society. This includes techniques in desalination, agricultural practices using saltwater, and energy generation from marine resources. Each of these applications highlights how saltwater can address sustainability challenges. They serve not only to meet the demands of a growing global population but also to utilize an abundant resource. As freshwater supplies dwindle, saltwater becomes more relevant in diverse sectors.
Desalination Techniques
Reverse Osmosis
Reverse Osmosis (RO) is a prominent desalination method. This technique uses a semipermeable membrane to separate water from salts. The key characteristic of reverse osmosis is its efficiency. It can remove up to 99% of salt and impurities from seawater. This makes it a popular choice for providing potable water in arid regions.
A unique feature of reverse osmosis is its ability to operate at lower energy levels compared to other methods. This results in reduced operational costs. However, it also has disadvantages, such as membrane fouling. Regular maintenance is necessary to ensure optimal performance and longevity of the system.
Multi-Stage Flash Distillation
Multi-Stage Flash Distillation (MSF) is another established desalination process. This method relies on the heating of seawater and its subsequent conversion to vapor. This vapor is then condensed into freshwater. The key characteristic of MSF is its capacity to produce large quantities of water.
One notable feature of MSF is its scalability. It can be designed to meet specific water demands for large populations. On the downside, it requires significant energy input, often resulting in elevated costs in energy-intensive regions.
Use in Agriculture
Sustainable Practices
Sustainable practices in agriculture with saltwater focus on efficient water usage. By applying saltwater to crops that can tolerate saline conditions, farmers can reduce freshwater reliance. This is especially crucial in areas with limited access to fresh water. The key characteristic of these practices is their environmental benefit. They promote soil health and assist in water conservation efforts.
A unique feature of these sustainable practices is the increased resilience of crops to climate variations. However, attention must be given to soil salinity levels to prevent adverse effects on crop yields.
Brackish Water Cropping
Brackish water cropping involves growing plants in environments with saline water. This method emphasizes utilizing non-potable water sources efficiently. The key characteristic of brackish water cropping is its ability to exploit salt-tolerant plant varieties. This approach is beneficial where traditional irrigation is not viable due to water scarcity.
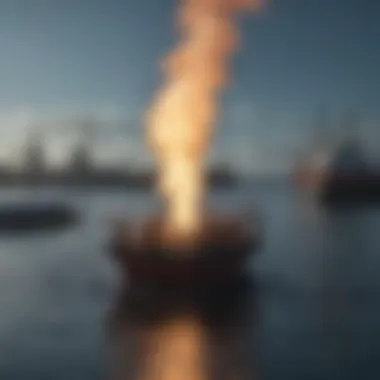
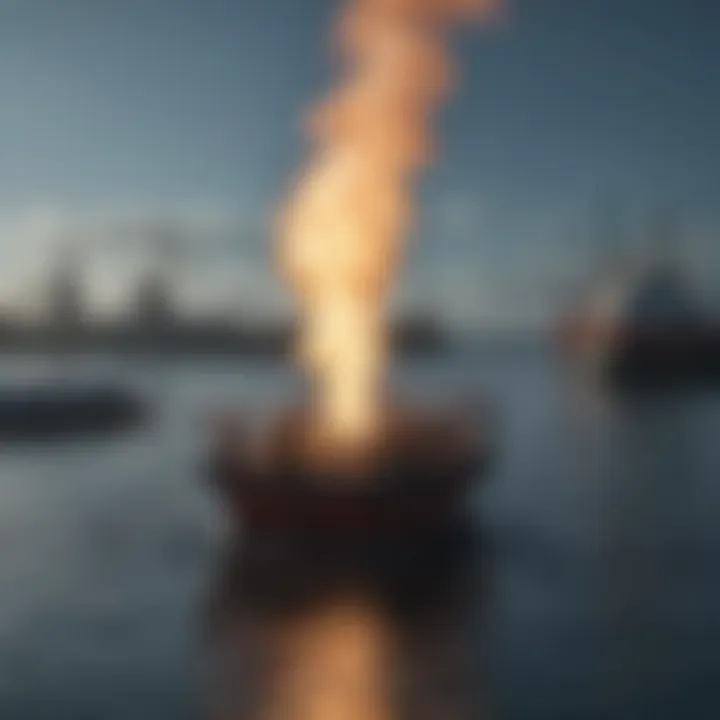
The unique feature of brackish water cropping is its potential to improve food security. However, there are challenges, such as the need for appropriate crop selection and ongoing research into salinity impact on plant growth.
Energy Generation
Salinity Gradient Power
Salinity Gradient Power (SGP) taps into the energy difference between freshwater and saltwater. This method can harness energy through processes such as Pressure Retarded Osmosis. The key characteristic of SGP is its renewable nature, as it continually utilizes the natural salinity gradient in rivers and estuaries.
A unique feature of salinity gradient power is its low environmental impact compared to traditional energy sources. However, the technology is still in developmental stages, and proper infrastructure is necessary to maximize its potential.
Wave Energy Conversion
Wave Energy Conversion (WEC) focuses on the kinetic energy generated by ocean waves. This method captures movement and transforms it into usable electricity. A key characteristic of wave energy conversion is its abundance. Coastal regions around the world present substantial opportunities for energy production.
One notable feature is its capacity to generate clean energy without the level of emissions associated with fossil fuels. However, challenges remain in terms of durability and efficiency of the technology in harsh marine environments.
Environmental Considerations
The intersection of saltwater direct with environmental stewardship offers a crucial lens through which to examine its implications and opportunities. Understanding the environmental considerations related to saltwater utilization is essential for balancing technological advancement with ecological integrity. The benefits of incorporating environmental principles into saltwater applications ensure a sustainable future, preventing degradation of natural resources while promoting innovative solutions to pressing challenges.
Impact on Marine Ecosystems
The extraction and utilization of saltwater inevitably affect marine ecosystems. While saltwater is abundant, its management must be critically assessed to prevent detrimental impacts on aquatic life. Discharges from desalination processes, for instance, may alter local salinity levels, affecting species that cannot adapt to rapid changes. Additionally, the abstraction of saltwater can disrupt habitats, particularly in regions where fish and other marine species depend on stable environments. Careful monitoring and mitigation strategies must be integrated into saltwater projects to minimize these impacts. Protecting biodiversity requires a holistic approach, considering both immediate and long-term consequences of saltwater use.
Management of Salinity Levels
Managing salinity levels is vital in both agricultural and ecological contexts. In agriculture, for instance, understanding saline balance can enhance crop resilience, allowing for the sustainable use of brackish water. Additionally, maintaining optimal salinity in coastal regions supports healthier ecosystems. This involves ensuring that practices like aquaculture and saltwater irrigation do not lead to the salinization of soils or freshwater systems. Strategies might include controlled irrigation, leaching, and the integration of salt-tolerant crops. The goal is to create a sustainable framework that secures both agricultural productivity and ecological health, effectively managing salinity while promoting resource efficiency.
Regulatory Frameworks
Establishing effective regulatory frameworks is critical for guiding the responsible application of saltwater technologies. Regulations can help balance innovation with environmental protection. Frameworks often include guidelines on discharge limits from desalination plants, ensuring that marine life is not adversely impacted by concentrated brine. Furthermore, regulatory bodies must promote transparency and accountability in saltwater projects, fostering collaboration between stakeholders. This approach can enhance public understanding and participation in decision-making processes. Regulations should also support research into new and more sustainable technologies, thereby encouraging continuous improvement in the management of saltwater resources.
"Ensuring a sustainable future begins with understanding the delicate interplay between technological advancements and environmental stewardship."
By addressing these key areas, the article lays a groundwork for understanding how saltwater direct can be harnessed responsibly, ensuring ecological balance while meeting growing demands. The sustainable management of saltwater applications not only preserves marine ecosystems but also aligns with broader environmental goals.
Technological Innovations
Technological innovations play a crucial role in the advancement of saltwater direct applications. As challenges grow in water scarcity and energy demand, innovation becomes vital to optimize the use of saltwater across various sectors. This section aims to highlight key advancements that create opportunities for improved efficiency and sustainability.
Advancements in Desalination
Desalination is a key technology in managing saltwater resources. Recent advancements have focused on enhancing efficiency and reducing costs. For instance, the introduction of advanced membranes in reverse osmosis has significantly increased water permeability, which lowers energy demands.
Moreover, researchers are working on integrating solar energy with desalination plants, making the process more sustainable. This method not only utilizes renewable energy but also addresses the high operational costs associated with traditional methods.
"Innovation in desalination technology is essential for addressing global water scarcity issues while minimizing environmental impacts."
These advancements emphasize the importance of research and development in realizing a sustainable future.
Saltwater Agriculture Technologies
Saltwater agriculture is emerging as a viable solution to combat food shortages in arid regions. Innovative techniques have been developed to grow crops in saline conditions. In particular, halophytes are plants that thrive in saltwater and can be cultivated using brackish water.
Technologies like hydroponics and vertical farming are being adapted to incorporate salt-tolerant species. These methods not only conserve freshwater but also maximize land use efficiency. Farmers are now exploring genetically modified organisms that can withstand higher levels of salinity, further bolstering agriculture in challenging climates.
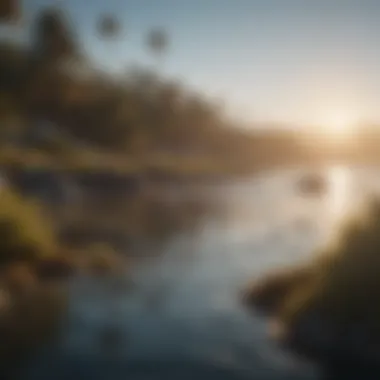
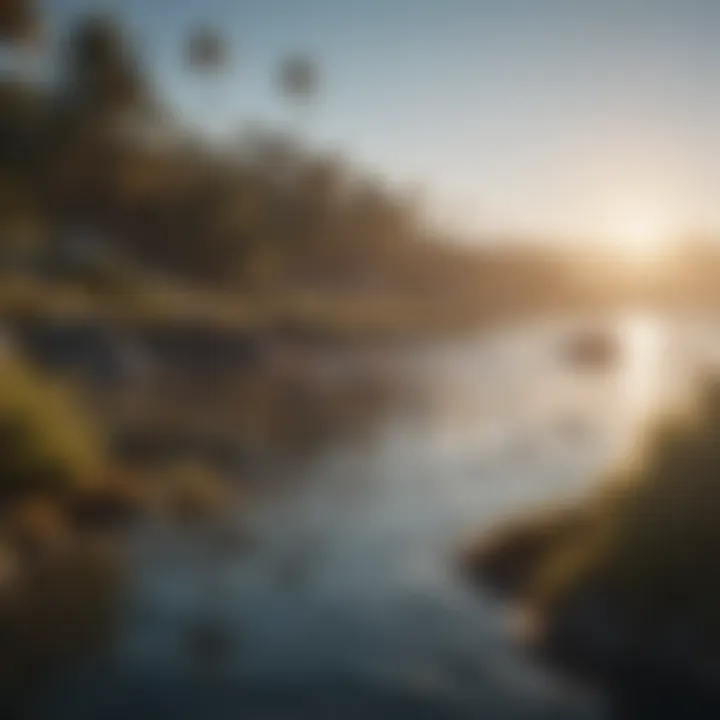
Emerging Energy Innovations
The convergence of saltwater technologies and energy production is promising. Salinity gradient power is one significant area, generating energy from the difference in salt concentrations between seawater and freshwater. This method, known as "blue energy," leverages both osmotic pressure and salinity differences to produce renewable energy.
Additionally, wave energy conversion is another area of innovation. By harnessing the kinetic energy from waves, new devices are being developed to convert that energy into usable power. Efforts to improve the efficiency of these systems could provide alternate energy sources while contributing to sustainable practices in coastal areas.
Challenges and Limitations
Understanding the challenges and limitations associated with saltwater direct is critical for harnessing its full potential. As industries increasingly turn to saltwater for various applications, addressing these issues will determine the feasibility and impact of such endeavors. Key areas to consider are economic viability, technical challenges, and the overarching policy and regulatory frameworks that govern these processes.
Economic Viability
Economic viability is a pivotal factor in the implementation of saltwater direct technologies. The costs associated with desalination, saltwater agriculture, and energy generation can be substantial. For instance, reverse osmosis, one of the most commonly used desalination methods, demands significant capital investment and operational expenses. These costs can deter small-scale operators from adopting such technologies. Moreover, fluctuations in energy prices can impact the overall economic stability of these projects.
On the other hand, successful economic models should also address how saltwater technologies can be integrated into existing infrastructure. Investment in these technologies must align with projected returns, which can include not just direct financial gains but also environmental benefits and improvements in accessibility to fresh water. Assessing long-term sustainability remains crucial for ensuring that economic considerations do not overshadow ecological impacts.
Technical Challenges
Technical challenges pose another significant barrier to the widespread use of saltwater direct technologies. Methods like multi-stage flash distillation can face difficulties relating to efficiency and material durability. Saltwater corrosiveness can deteriorate equipment, leading to increased maintenance costs and downtime.
Additionally, scalability remains a concern. Many technological solutions succeed in controlled environments but struggle to maintain performance in real-world conditions. For example, innovations in saltwater agriculture must adapt to various soil and climate conditions. This adaptability is essential to ensure crops can thrive without excessive reliance on freshwater resources. Continuous research and development in material science and engineering are necessary to overcome these obstacles and develop reliable systems that can function at scale.
Policy and Regulation Issues
Policy and regulation play a significant role in shaping the future of saltwater direct initiatives. The formulation of effective regulatory frameworks is essential to ensure that saltwater technologies are deployed responsibly. Lack of clear policies can lead to inconsistent practices and may undermine public trust in these solutions.
For saltwater agriculture, policies must address how to optimize land use while protecting local ecosystems. Regions often have different regulatory environments, which can complicate the importation of saltwater technologies across borders. Collaboration between governments, academic institutions, and industry will be necessary to create frameworks that promote responsible practices.
Successful navigation of these challenges can potentially enhance resource management and contribute to global sustainability efforts.
Future Directions
The study of saltwater direct is evolving rapidly, making the exploration of future directions essential. Emerging challenges such as climate change, freshwater scarcity, and energy demands necessitate comprehensive strategies that incorporate saltwater utilization. Understanding how to harness this resource effectively could revolutionize various sectors, while also addressing critical societal concerns. By delving into future opportunities, we can lay the groundwork for sustainable practices and technological advancements that leverage saltwater resources.
Research Opportunities
Research in saltwater direct opens numerous avenues for innovation. For example, examining new desalination methods can improve efficiency and reduce costs. Current methods, including reverse osmosis, still require extensive energy consumption. Researchers can explore alternative materials and processes that minimize energy use while maximising output. Additionally, investigating the biological aspects of saltwater can provide insight into creating crops and plants that thrive in saline environments. This exploration can lead to breakthroughs in food security and agricultural resilience.
"Innovative research in saltwater technologies holds the key to addressing global challenges, particularly in areas facing water shortages and food insecurity."
Sustainability Goals
Sustainability remains at the forefront of discussions surrounding saltwater direct. Setting clear sustainability goals is crucial for ensuring that saltwater applications do not harm aquatic ecosystems or local communities. Strategies must include responsible resource extraction, waste management, and energy efficiency. Moreover, integrating saltwater agriculture practices can aid in developing sustainable food systems. This approach is not only viable but necessary as reliance on freshwater resources continues to escalate across the globe.
Potential for Global Impact
The potential impact of saltwater direct is significant on a global scale. Implementing effective saltwater technologies could alleviate water stress in arid regions, improving the quality of life for millions. Moreover, advancements in energy generation from saltwater sources, such as salinity gradient power, can contribute to cleaner energy solutions. This could foster energy independence in many nations while mitigating the effects of climate change. The collaboration of governments, scientists, and industries focused on saltwater applications can drive collective progress towards a better future for the planet.
Ending
The conclusion is a critical aspect of this article, synthesizing the insights discussed throughout the sections. It serves as a final distillation of the importance of saltwater direct, consolidating key themes that emerged in relation to its principles and applications. This topic is vital due to its implications for various fields, namely engineering, agriculture, and energy production. A robust understanding of saltwater direct aligns with contemporary challenges in resource management and sustainability efforts.
Summary of Key Points
The article has highlighted several essential points regarding saltwater direct:
- Definitions and History: An initial overview of what saltwater direct entails, coupled with its historical context, helps frame the discussion.
- Scientific Principles: The breakdown of the chemical composition, physical properties, and thermodynamics involved clarifies the fundamental science behind the process.
- Applications: Delving into practical uses, such as desalination techniques and agricultural practices, underlines its real-world significance.
- Environmental Impact: Addressing how saltwater direct interacts with marine ecosystems and the necessity for proper salinity management is crucial.
- Technological Innovations: Advancements emerging in desalination and agriculture accentuate the ongoing evolution in the field.
- Challenges: Economic viability and technical hurdles remind us of the complexities governing this domain.
- Future Directions: Identifying research opportunities and sustainability goals grounds our understanding in a future-oriented context.
Final Thoughts
In summation, saltwater direct is more than just a technical process; it embodies a pathway towards addressing critical global issues, such as water scarcity and energy generation. Its applications in agriculture and energy production highlight its versatility and potential for sustainable development. As our awareness and capabilities grow, the role of saltwater direct in shaping resource management will be increasingly significant.
Adopting these practices could not only alleviate environmental stress but also promote resilience in food production systems. Thus, continued exploration and investment in saltwater direct are necessary for achieving broader sustainability aims, ensuring that we rethink our relationship with water as a vital resource.