Exploring Radioactive Counters: Principles and Applications
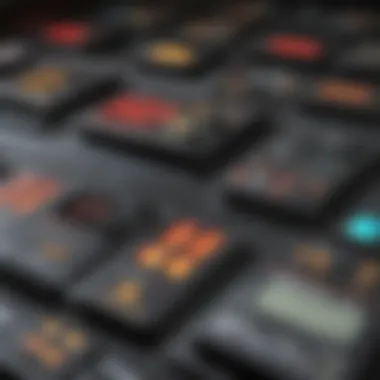
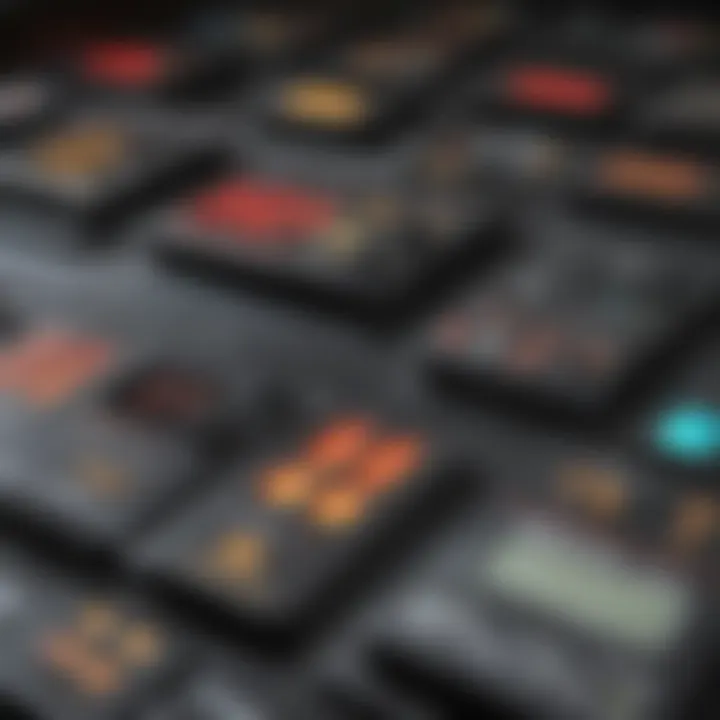
Intro
In the realm of science, particularly in fields dealing with nuclear physics and radiation safety, the radioactive counter stands out as an essential instrument. Its ability to detect and measure radioactive decay serves a multitude of purposes, ranging from scientific research to health monitoring. As we navigate through the workings of these devices, we’ll explore not only the principles guiding their operation but also their practical applications and advancements that have enhanced their effectiveness.
Overview of Research Topic
Brief Background and Context
Radioactivity, the phenomenon where unstable atomic nuclei lose energy by emitting radiation, has garnered significant attention since its discovery. Understanding how to measure the decay of these radioactive isotopes is crucial in various scientific disciplines. Radioactive counters, such as Geiger-Müller counters and scintillation counters, have evolved over the decades to become sophisticated tools that can quantify radiation levels effectively.
Importance in Current Scientific Landscape
With growing concerns about nuclear energy, medical imaging, and radiation therapy, the role of radioactive counters has never been more pertinent. They contribute to safety protocols in hospitals, environmental monitoring, and even archaeology—where they assist in dating artifacts. Their applications stretch far and wide, illustrating their significance in the ongoing conversation about public health, environmental protection, and scientific research.
Principles of Radioactive Counters
Radioactive counters operate based on fundamental principles of physics and radioactivity. At the heart of these devices is the detection of ionization produced by radioactive particles as they traverse a medium.
- Geiger-Müller Counters: These utilize a filled tube that becomes conductive when ionizing radiation passes through it, triggering a measurable current.
- Scintillation Counters: These convert light emitted from scintillating materials into an electrical signal, allowing for precise counting of photons produced by radiation.
Understanding these principles illuminates the technological advancements in improving sensitivity and specificity in measurements, aiding researchers in various fields from medicine to environmental science.
Applications of Radioactive Counters
Medical Fields
In healthcare, radioactive counters play a pivotal role in diagnostics and treatment. They are invaluable in ensuring safety during radiological procedures. For instance:
- Monitoring patient exposure during X-rays or CT scans.
- Assessing dosage for radioactive therapeutics in cancer treatment.
Environmental Monitoring
These instruments are also critical for detecting radiation levels in the environment. Agencies utilize them to:
- Measure background radiation.
- Ensure compliance with safety standards near nuclear facilities.
Research and Academia
In laboratories, radioactive counters enable experimental physicists and nuclear chemists to conduct research on nuclear reactions and isotope behavior, refining the scientific community’s knowledge base.
Advancements in Detection Technology
Technological progress continues to shape the landscape of radioactive detection. Innovations focus on improving accuracy, reducing response times, and integrating smart technology features into devices. Some of the notable advancements:
- Minaturization of detectors, making them portable and easier to use in fieldwork.
- Application of digital technology, allowing for real-time data analysis and remote monitoring.
"The ongoing advancements in radioactive counter technology not only enhance measurement capabilities but also contribute to broader safety measures in various sectors."
Finale
To conclude, radioactive counters embody a blend of historical significance and modern technological evolution. Their fundamental principles and diverse applications demonstrate an ongoing commitment to research, safety, and public health. The road ahead for these instruments is promising, with innovations hinting at further improvements that could transform how we measure and understand radioactivity in our world.
Preface to Radioactivity
Radioactivity is a fundamental phenomenon that lies at the heart of many scientific explorations and varied applications. Recognizing its significance is pivotal for students, researchers, and educators aiming to grasp the scope of modern science. Delving into radioactivity not only enunciates its historical underpinnings but also sheds light on the principles governing radioactive decay, vital for comprehending the intricate mechanisms behind radioactive counters. These counters serve as essential instruments in detecting and measuring radioactivity, making them increasingly relevant in our scientifically driven world.
Historical Context
The narrative of radioactivity is richly layered, extending back to the late 19th century. It's when pioneers like Henri Becquerel, along with Marie and Pierre Curie, opened a new chapter in understanding the natural world. They stumbled upon the phenomenon almost accidentally while studying phosphorescent materials. Their discovery of radium and polonium transformed chemistry and physics, prompting further inquiries into the stability of the atomic nucleus.
Prior to these remarkable findings, the atom was seen merely as indivisible particles. But the advent of radioactivity revolutionised this notion, illustrating that these particles could decay, emit energy, and transform into different elements over time. This landmark revelation laid the groundwork for not just nuclear physics but also established a connection to countless applications ranging from medical treatments to energy production. Recognizing this historic development is crucial because it informs how today's scientists approach problems related to radiation and safety protocols, grounding their methodologies in a well-established past.
Fundamental Concepts of Radioactive Decay
Understanding radioactive decay involves grasping some key principles that govern this natural process. At its core, radioactive decay refers to the disintegration of unstable atomic nuclei, resulting in the emission of particles and energy. Various types of decay exist, with alpha decay, beta decay, and gamma decay representing the most common forms.
- Alpha Decay: Here, an atomic nucleus releases alpha particles, composed of two protons and two neutrons. An example is the decay of uranium-238 into thorium-234.
- Beta Decay: This involves the conversion of a neutron into a proton, resulting in the emission of a beta particle, which is essentially an electron. Carbon-14, for instance, undergoes beta decay, making it instrumental in radiocarbon dating.
- Gamma Decay: In this process, a nucleus emits gamma rays—a type of high-energy electromagnetic radiation—without changing the number of protons or neutrons. It's often associated with alpha and beta decay as the nucleus sheds excess energy.
The rate of these decay processes is characterized by their half-lives—the time required for half of a sample to decay. This concept, along with the various decay modes, illustrates the ephemeral nature of radioactive materials, emphasizing the need for effective detection methods.
In summary, comprehending the historical dimension and fundamental concepts of radioactivity is critical for anyone stepping into the field of nuclear science. This knowledge fosters a deeper appreciation for how radioactive counters are not just mere tools but windows into the atomic world, where energy, safety, and advanced technology intersect.
Principles of Radioactive Counters
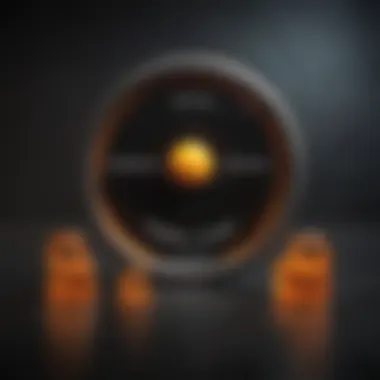
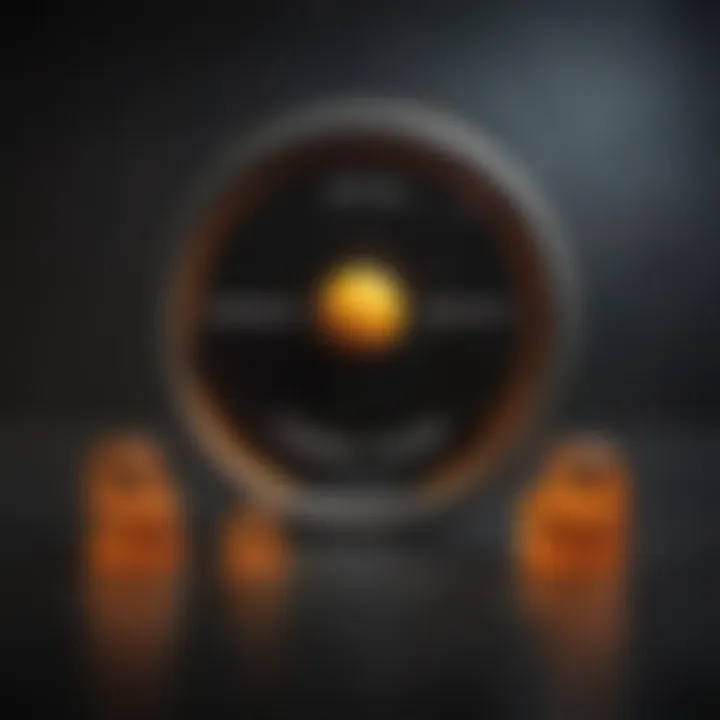
Understanding the principles behind radioactive counters is crucial for grasping how these instruments effectively measure ionizing radiation. Each counter operates on specific detection mechanisms that allow for accurate readings, while calibration ensures the reliability of the results. The interplay between different detection methods and their calibration is vital for professionals in fields such as nuclear physics, health physics, and environmental science. This section explores the ways radioactive counters function, the mechanisms that underlie their detection capabilities, and the importance of proper calibration and sensitivity.
Detection Mechanisms
Detection mechanisms play a key role in how radioactive counters function effectively. There are several types of detectors, each with unique characteristics and benefits.
Gas-Filled Detectors
Gas-filled detectors are well-known for their simple operational mechanics. They consist of a sealed chamber filled with gas, usually a noble gas like argon or helium, which becomes ionized when radiation passes through it.
- Key Characteristic: One major advantage is their ability to give relatively rapid responses to radiation, making them suitable for real-time monitoring.
- Unique Feature: They can measure alpha particles, beta particles, and gamma rays depending on their design.
- Advantages/Disadvantages: However, while they're cost-effective, these detectors may show lower precision in energy measurements. For tasks requiring pinpoint accuracy, alternative detectors might be preferred.
Scintillation Counters
Scintillation counters utilize special materials that emit light when struck by radiation. This light is then converted into an electrical signal, which can be measured.
- Key Characteristic: Their sensitivity makes them a popular choice for detecting low levels of radiation, embodying a useful tool for radiation surveillance.
- Unique Feature: Scintillation counters can effectively measure gamma rays and various nuclear particles, providing detailed energy information with each interaction.
- Advantages/Disadvantages: While they excel in accuracy, their dependence on photomultiplier tubes raises the cost and complexity, making maintenance more challenging.
Semiconductor Detectors
Semiconductor detectors function differently than gas-filled or scintillation counters. They use solid materials like silicon or germanium to detect radiation. When radiation interacts with the semiconductor, electron-hole pairs are created, leading to a measurable current.
- Key Characteristic: Their energy resolution is another notable advantage, allowing these detectors to differentiate between various energy levels of radiation.
- Unique Feature: They can measure alpha, beta, and gamma radiation accurately, making them versatile instruments in various settings from labs to hospitals.
- Advantages/Disadvantages: Yet, they require careful temperature control and can be quite expensive, which may limit their practicality in some applications.
Calibration and Sensitivity
Calibration refers to the process of adjusting the instruments to ensure their readings are accurate. This is crucial in maintaining the reliability of radioactive counters. Proper calibration can also enhance sensitivity, which is vital for detecting small amounts of radiation.
In practice, the calibration process involves comparing the measurements of the counter with a known radiation source, identifying any discrepancies, and making necessary adjustments. Regular calibration ensures not only that the instrument remains within safety standards but also that it provides precise data for further analysis.
Sensitivity is another critical aspect. The ability of a counter to detect low levels of radiation is vital across varied settings—from environmental monitoring to medical diagnostics. High-sensitivity counters can identify subtle changes in radiation levels, which inform safety protocols in areas such as nuclear facilities or clinical environments.
In summary, the principles governing radioactive counters involve exploring the functions of different detection mechanisms and understanding the importance of calibration and sensitivity. Appropriate factors in their operating principles can lead to effective measurement of ionizing radiation, guiding professional applications and safety measures.
Types of Radioactive Counters
Understanding the types of radioactive counters is essential because each type serves unique roles, catering to specific objectives in research, diagnostics, and environmental monitoring. The significance lies not only in how they detect radiation but also in their varied applications across multiple fields. Selecting the right counter for the task at hand can greatly influence the accuracy of results and the safety of procedures. The distinctions among these counters allow scientists and professionals to tailor their approach according to the nature of the radiation they aim to measure.
Geiger-Müller Counters
Design and Functionality
Geiger-Müller counters, often simply referred to as Geiger counters, are popular devices in the field of radiation measurement. Their design typically consists of a Geiger-Müller tube filled with gas and electrodes. When radiation interacts with the gas inside this tube, it ionizes the gas atoms, creating a cascade of charged particles, which ultimately generates an electrical pulse. This pulse is then converted into an audible click or a reading on a scale, thereby alerting users to the presence of radiation.
One significant characteristic of the Geiger-Müller counter is its ability to detect a wide range of radiation types, including alpha particles, beta particles, and gamma rays. This versatility makes it a favored choice in both educational settings and fieldwork.
However, this design isn’t without limitations. For instance, while they are excellent for detecting high levels of radiation, Geiger counters can be less effective in measuring very low levels, leading to potential underreporting of hazardous exposure. This reliance on gas ionization poses a disadvantage when precision is critical in scientific measurements.
Applications
The applications of Geiger-Müller counters extend across various sectors. They are particularly important in environmental monitoring, helping track background radiation levels or detect radioactive contamination after accidents. In medical fields, they find utility in monitoring safety levels when using radioactive isotopes in treatment.
A key feature of their applications is the ease of use; even those without extensive training can operate them effectively. That being said, their disadvantage arises from their inability to provide specific energy information about radiation sources. Thus, while they can indicate the presence of radiation, they do not offer insight into the type or energy of the particles detected—this gap may require pairing them with more specialized devices for complete analysis.
Gamma Ray Spectrometers
Gamma ray spectrometers are crucial for analyzing the energy spectrum of gamma radiation. They help identify different isotopes based on the energy of the gamma rays they emit. Unlike Geiger counters, these devices provide detailed information by using crystals or semiconductor materials to convert gamma rays into detectable pulses. The energy of these pulses reveals nuances about the radioactive materials, helping professionals make informed decisions in nuclear research, security, and safety.
Alpha Particle Detectors
Alpha particle detectors are tailored to specifically capture alpha radiation, which consists of heavy, charged particles. These detectors typically utilize materials like silicon or gas that respond accurately to alpha emissions. Their unique design allows for the sensitivity needed to measure alpha particles, which, due to their larger mass and charge, have limited penetration ability compared to beta particles and gamma rays.
One specific advantage of these detectors is their pivotal role in nuclear safety and health physics, especially in environments where alpha-emitting isotopes are handled. However, a common disadvantage is that their usage primarily hinges on having direct contact with the source of radiation, necessitating caution and careful handling practices.
Overall, understanding the types of radioactive counters equips scientists, educators, and industrial professionals with the necessary insights to choose the appropriate instruments according to their unique requirements and the challenges presented by different radiation types.
"Selecting the right radioactive counter is not merely about preference; it's about ensuring accuracy, safety, and efficiency in measurements."
This layered approach to understanding radioactive counters is vital for anyone involved in fields where radiation is a factor, ensuring they make informed choices founded on the inherent strengths and weaknesses of each type.
Applications of Radioactive Counters
Radioactive counters, these essential instruments, play a pivotal role across various fields by measuring ionizing radiation. Their applications are vast, influencing sectors like environmental science, medicine, and research. Understanding these applications is critical, particularly in an era where safety and accuracy are non-negotiable. By exploring specific elements, benefits, and considerations surrounding the use of radioactive counters, we can appreciate their significance in maintaining both public health and environmental integrity.
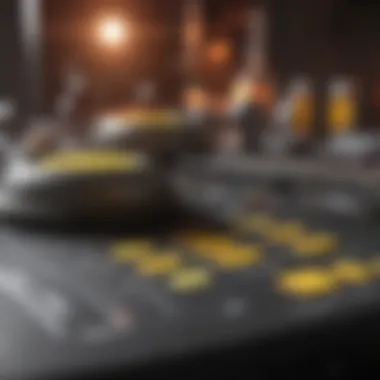
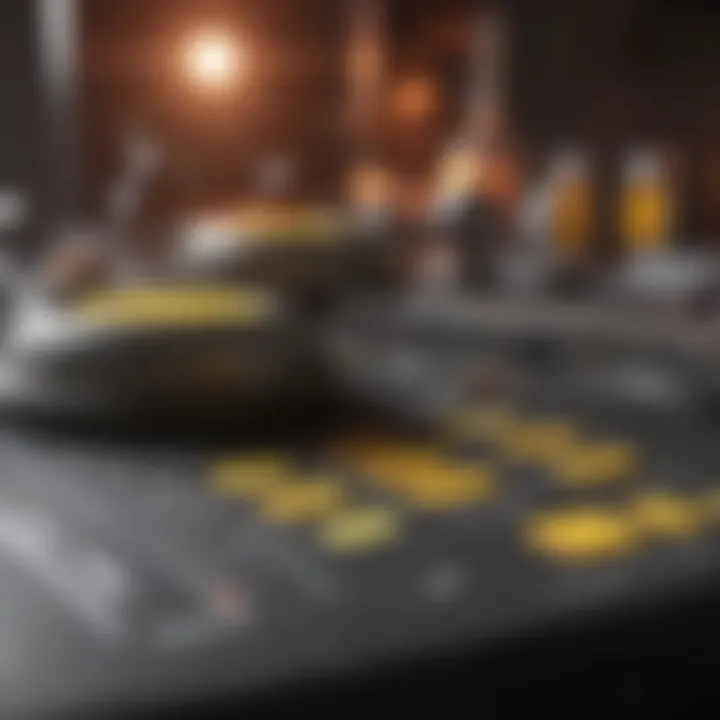
Environmental Monitoring
Environmental monitoring involves assessing the levels of radiation present in our surroundings. It's crucial for ensuring that ecosystems remain healthy and that human exposure to harmful levels of radiation is minimized. Radioactive counters are indispensable in this field for several reasons.
- Assessing Pollution: Radioactive counters are used to measure radiation levels in soil, air, and water. This is vital in areas that have experienced nuclear accidents, such as Chernobyl or Fukushima.
- Wildlife Studies: Monitoring wildlife in terms of radiation exposure helps understand the ecological impact of radioactive materials. For instance, scientists often use these counters to track radiation levels in animal populations living near contaminated sites.
- Waste Management: Effective waste management practices require consistent monitoring of radioactive waste sites. By employing these counters, organizations can track leaks and prevent environmental disaster.
"Radioactive counters not only measure the unseen; they safeguard our future by tracking radiation sources that must be managed responsibly."
The benefits of employing radioactive counters in environmental monitoring extend beyond mere detection. They help build comprehensive databases that inform policy decisions regarding land use and environmental protection strategies. Plus, they foster community awareness about radiation exposure and environmental health.
Medical Diagnostics and Treatment
In the realm of healthcare, radioactive counters are instrumental in diagnostics and treatment, offering life-saving insights and facilitating advanced therapies. From diagnosing conditions to treating cancer, these instruments hold immense value in medical applications.
- Imaging Techniques: Devices like gamma cameras use scintillation counters to create images of the inside of the body, enabling healthcare professionals to identify abnormalities accurately.
- Radiation Therapy: In cancer treatment, precision is paramount. Radioactive counters help healthcare providers deliver targeted doses of radiation, minimizing damage to surrounding healthy tissues. This careful balance is what makes the treatment effective while reducing side effects.
- Thyroid Disorders Management: Radioactive iodine therapy is commonly used to treat hyperthyroidism. By measuring how much iodine is absorbed by the thyroid, medical professionals can determine appropriate treatment plans.
Utilizing radioactive counters in medicine brings remarkable advancements in diagnostic capabilities, encourages earlier disease detection, and optimizes treatment effectiveness. As technology evolves, we can expect even greater integration of these devices in healthcare initiatives.
Research and Development
The world of research and development thrives on precision and accuracy, and radioactive counters provide the backbone for many scientific inquiries. Their roles in research are multifaceted, supporting everything from fundamental physics to cutting-edge biomedical studies.
- Nuclear Physics Research: Scientists employ radioactive counters to study nuclear decay processes and interactions, contributing to the broader understanding of atomic structure and stability.
- Development of New Materials: In various industries, from aerospace to electronics, research teams utilize radiation measurements to ensure that new materials can withstand high radiation levels without deteriorating.
- Public Health Studies: These counters assist in epidemiological studies, monitoring populations for radiation exposure and its effects on health, thereby guiding public health policies and interventions.
As research expands, the sophistication of radioactive counters is also increasing. Emerging technologies and methodologies promise to enhance their capabilities, allowing for more comprehensive studies and increased reliability in findings.
In summary, the applications of radioactive counters are vital and far-reaching, impacting environmental safety, medical practices, and scientific research. Recognizing their importance helps underscore the continuing need for innovation and excellence in radioactive detection technologies.
Impact on Health and Safety
The impact of radioactive counters on health and safety cannot be overstated. These devices play a pivotal role in safeguarding individuals and communities from the potential harms associated with radiation exposure. In a world where radioactive materials are present in medical facilities, research laboratories, and even construction sites, the effective measurement of radiation levels is crucial. With the ability to detect radioactive materials before they pose a significant health risk, these counters contribute directly to ensuring safety standards and compliance with regulations.
Radiation Protection Standards
Standards for radiation protection are designed to limit exposure and minimize the risks associated with ionizing radiation. Various organizations, like the International Commission on Radiological Protection (ICRP) and the Nuclear Regulatory Commission (NRC), have established guidelines that outline safe exposure limits for both workers and the general public. These standards are important because they provide a framework for ensuring that radioactive counters are used effectively in various contexts.
- Principles of Radiation Protection:
- Justification: No action involving ionizing radiation should be undertaken unless it produces sufficient benefits.
- Optimization: Radiation exposure should be kept as low as reasonably achievable (ALARA).
- Limitation: There should be defined dose limits for radiation exposure.
Radiation protection standards are essential for regulating the use and operation of radioactive counters within different environments. They inform how detectors should be calibrated and maintained, ensuring that they provide accurate readings that help mitigate health risks. Moreover, such standards promote the regular training of personnel on how to use these instruments safely and effectively.
Role of Radioactive Counters in Safety Protocols
Radioactive counters serve as a critical component of safety protocols across various sectors. Their functionalities enable the timely detection of radiation levels, which is essential in risk assessment, emergency response, and regulatory compliance.
For instance, in a medical setting, devices like Geiger-Müller counters can be used to monitor radiation exposure for healthcare workers as well as patients undergoing certain diagnostic procedures. In the event of an unexpected radiation leak or spill, these counters can quickly pinpoint the source and determine the extent of contamination, thereby facilitating swift action to minimize exposure.
- Best Practices for Safety:
- Regular calibration and maintenance of radioactive counters ensure accuracy and reliability.
- Personnel training on operational protocols boosts confidence in handling potentially hazardous situations.
- Continuous monitoring with advanced counters provides real-time data, enabling timely decision-making.
"The penny drops when the importance of real-time monitoring during emergencies is understood; it is not just a matter of compliance but a lifesaver."
The reliance on accurate readings from radioactive counters can not only prevent accidents but also reinforce the trust of the public in safety measures undertaken by organizations. By integrating these devices into routine safety inspections and protocols, facilities can maintain stricter control over radioactive exposure and ensure adherence to established safety standards.
In summary, as society grapples with the presence of radioactive materials in everyday life, understanding the impact these counters have on health and safety becomes fundamental. The balance between harnessing the benefits of radioactivity and protecting human health is a delicate yet essential endeavor.
Advancements in Detection Technology
In the field of radioactivity, detection technology is not merely an auxiliary tool; it stands at the forefront of progress in understanding and managing radiation. As various industries increasingly embrace the importance of measuring radioactive decay with precision, advancements in detection technology have become crucial. These enhancements not only improve accuracy but also offer novel applications that were once deemed unattainable. From environmental conservation efforts to medical diagnoses, the far-reaching implications of these advancements merit a thorough exploration.
Modern Innovations in Counter Design
The journey of radioactivity detection began with rudimentary designs that primarily emphasized simplicity. Fast forward to today, and one can observe striking innovations that have dramatically transformed counter design. For instance, the Geiger-Müller counter has undergone significant refinements. Originally limited in its sensitivity and range, modern versions are tailored to respond to a broader spectrum of radiation types. They include sophisticated features such as energy discrimination, which enables the differentiation between alpha, beta, and gamma radiation.
Another remarkable example is the scintillation detector. This technology has seen improvements in its scintillation materials, with new compositions that result in enhanced light output and reduced background noise. Such advancements ensure that faint signals, crucial for specific applications, can be detected with greater reliability.
Key attributes of modern counter design include:
- Enhanced sensitivity: Allows detection of lower levels of radiation.
- Robustness: New materials and designs improve durability under various environmental conditions.
- Ergonomic integration: Actively designed for user comfort and ease of operation, adapting to diverse settings.
As these counters continue to evolve, researchers remain excited about the potential for emerging materials, such as graphene, which could redefine detection capabilities altogether.
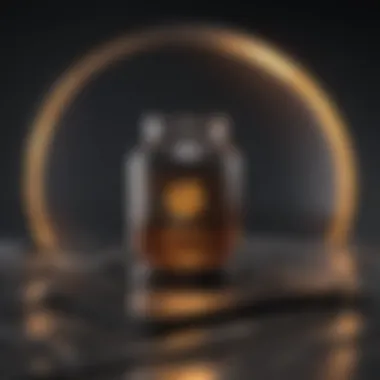
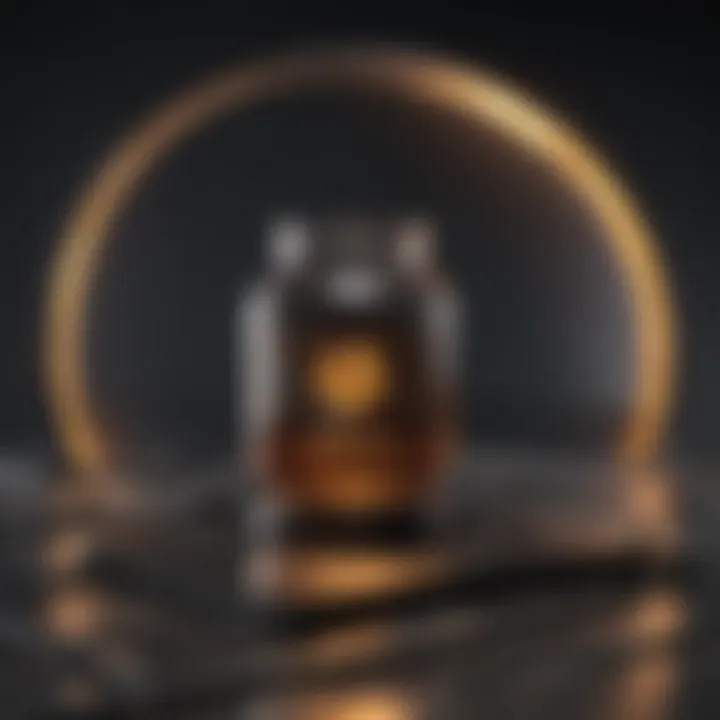
Integration with Digital Technologies
The fusion of detection technology with digital platforms has ushered in a new era for radioactive counters. This integration yields an impressive array of possibilities, which can significantly improve data collection and analysis. Sensors linked to digital interfaces allow for real-time monitoring and adjustments, creating a feedback loop that enhances control over detection processes.
One notable advancement is the application of machine learning algorithms to filter out noise and identify patterns in radiation data. By training models on large datasets, it becomes feasible to recognize anomalies that may otherwise go unnoticed. Such inteligence is invaluable in various fields, including medical diagnostics where precise interpretation of radiological data can lead to earlier interventions and better patient outcomes.
"Integrating digital technologies with radioactive counters marks a monumental shift, offering unprecedented levels of accuracy, sensitivity, and usability across applications."
Furthermore, mobile applications connected to detectors enable users to access radiation readings on-the-go. This innovation is particularly relevant for emergency responders and environmental scientists who require immediate data for decision-making.
The benefits of this digital synergy are abundant:
- Enhanced data visualization: Graphical presentations aid in the interpretation of complex radiation metrics.
- Remote monitoring: Stations can be monitored from afar, which is especially valuable in hazardous environments.
- Streamlined data management: Integration promotes efficient record-keeping and data analysis, simplifying compliance with safety standards.
In summary, as we traverse deeper into the age of digitalization, the collaborative potential of advanced detection technology and digital solutions promises an expanse of new opportunities, revolutionizing how we perceive and manage radioactive materials.
Challenges in Radioactive Detection
The detection of radioactive materials is crucial for many fields, but it comes with its own set of hurdles. Understanding these challenges is essential for improving existing technologies and ensuring accurate measurements in various applications, from environmental monitoring to medical uses. A deep dive into this section reveals both the technical and financial barriers that researchers and engineers face.
Interference and Noise
One significant issue in radioactivity detection is interference from external factors that can skew results. Environmental noise—whether from background radiation or electronic signal interference—can muddy the waters, leading to misinterpretations of data.
For example, in areas with high natural radiation, such as near granite formations, detectors may record more events than actually occur from a specific source. Similarly, electromagnetic interference from nearby equipment can introduce false positives, causing equipment to register signals that do not originate from radioactive decay.
It's important for scientists to not only understand these noise factors but to develop strategies to mitigate their effects. Some methods include:
- Shielding: Physically protecting detectors from external radiation using lead or other dense materials
- Software Filtering: Implementing algorithms that can discern between genuine signals and background noise
- Calibration: Regularly adjusting equipment to account for any environmental changes that could affect readings.
"The integrity of data in radioactive detection hinges on recognizing and mitigating interference, as even small inaccuracies can lead to large misjudgments."
Cost Implications in Development and Maintenance
While advancements in radioactive detection technologies promise greater efficiency and accuracy, these developments often come with hefty price tags. Building and maintaining sophisticated detection systems can be quite an investment. Often, funding allocation for research and development is limited, which can stall progress or create disparities in detector availability across different entities.
Budget constraints can lead to:
- Compromised Quality: Using lower-grade materials or outdated technologies to cut costs may result in less reliable measurements.
- Maintenance Disparities: Organizations with fewer resources may struggle to maintain their equipment adequately, leading to inaccuracies over time.
- Innovation Stagnation: When financial resources are tied up in maintaining existing equipment, there’s little room for exploring new technologies or methods, limiting advancements in the field.
In exploring the future, it will be essential to balance the costs and benefits of these technologies carefully. Finding ways to minimize expenses while ensuring high-quality detection can propel research and applications forward, providing communities with safer and more accurate tools for managing their radioactive materials.
Future Directions in Radioactive Counters
In the landscape of scientific research and safety, radioactive counters emerge as pivotal tools. As we look ahead, the future of these instruments is bright with possibilities. Understanding where this technology is headed is crucial, not just for enthusiasts but for practitioners in health, environmental studies, and nuclear safety. The advancements in this field shouldn't be regarded as mere upgrades; they represent the evolution of our capacity to manage and understand radioactive materials.
Predicted Advances in Calibration Techniques
One of the cornerstones of radioactivity measurement is calibration. It ensures accuracy and reliability in reading, which are vital in such sensitive and potentially hazardous environments. Future innovations in calibration techniques promise to enhance precision and lower the margin of error. Technological advancements, such as AI-driven calibrators and automated calibration processes, could emerge to provide real-time adjustments based on environmental changes.
"Precision in calibration is not just a goal, it’s a necessity for safety and accuracy in radioactive measurement."
Moreover, incorporating pseudo-random sampling techniques might also be a game changer. These methodologies would allow a more extensive range of readings over time, leading to a deeper statistical analysis. With better datasets, scientists could better interpret radioactivity patterns, improving our understanding of radiation sources and their impacts on health and environment.
Potential for Broader Applications
The realm of radioactive counters is not static, and there is much room for expansion in their applications. Beyond traditional settings such as hospitals and nuclear power plants, we see the potential for these devices in emergency response, national security, and public health surveillance. For instance, in areas prone to disasters, portable radioactive counters could help detect contamination swiftly, allowing for timely interventions.
Furthermore, industries like mining and waste management are beginning to adopt these devices in ways we had not seen before. The challenges of managing radioactive byproducts can be daunting, but with improved counters, monitoring processes can be streamlined, ensuring compliance with safety standards while reducing risks.
The technology doesn't stop at mere detection; future iterations might include real-time data sharing features, enabling collaborative responses across different sectors. Imagine a scenario where hospitals, environmental agencies, and emergency services operate on a unified platform, utilizing data from radioactive counters.
The collaborative potential is immense, promoting a safer and more informed approach to radiation management across a multitude of fields. As we usher in these technologies, one thing is certain: the capabilities of radioactive counters will undoubtedly expand, creating a ripple effect that impacts various disciplines.
End
In looking back over the scope of radioactive counters, their significance in both scientific research and society becomes abundantly clear. These instruments are not merely gadgets; they’re vital components that help us navigate through the uncertainties of radioactivity. From the initial principles of radioactive decay to the sophisticated applications and emerging technologies, the article sheds light on how understanding these counters can broaden our knowledge and improve safety standards.
Summary of Key Points
- Understanding Radioactivity: We discussed the nature of radioactivity and its historical context, highlighting how scientists have unraveled its mysteries over the years.
- Principles of Detection: Various detection mechanisms, including gas-filled detectors and scintillation counters, were explored to emphasize their unique functionalities.
- Diverse Applications: The uses of these counters range from environmental monitoring to medical diagnostics, showcasing their indispensable role in different fields.
- Health and Safety: Familiarity with radiation protection standards underscored the critical importance of these devices in safety protocols, aiming to safeguard both the public and professionals handling radioactive materials.
- Technological Advancements: The article elaborated on the innovations in detection technology that increase accuracy and reliability, ensuring that we stay ahead in this fast-evolving domain.
- Future Directions: Insights into potential advancements in calibration techniques and broader applications illuminated the promising trajectory of research in radioactive counters.
Final Remarks on the Importance of Radioactive Counters
As we conclude, it's imperative to underline the indispensable role radioactive counters play in various sectors. Their importance goes beyond mere detection; they serve as fundamental tools for scientific inquiry, monitoring environmental safety, and enhancing medical practices. Furthermore, with advancements in technology, these counters stand to become even more effective in providing accurate readings and insights.
Ultimately, every advancement in detection technology translates to better safety protocols, informed public health measures, and a deeper understanding of the radioactive phenomena that surround us. In a world increasingly reliant on science and technology, the continued investment in the study and improvement of these counters is not simply a choice—it’s a necessity.
"The understanding of radioactive counters not only protects lives but also propels research in fields that matter."
In light of all these points, the significance of radioactive counters cannot be overstated. They are tools that not only enhance our safety but also drive scientific discovery into uncharted territories. With a grasp of their principles, applications, challenges, and future directions, students, researchers, and professionals are better equipped to engage with this dynamic area of study.