Exploring the Intricacies of Magnetic Crystals
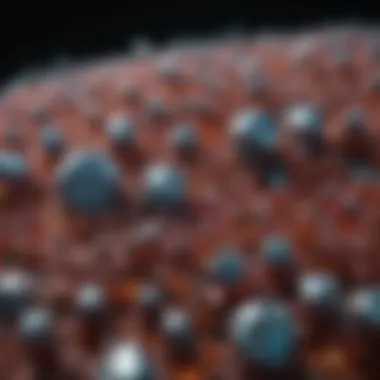
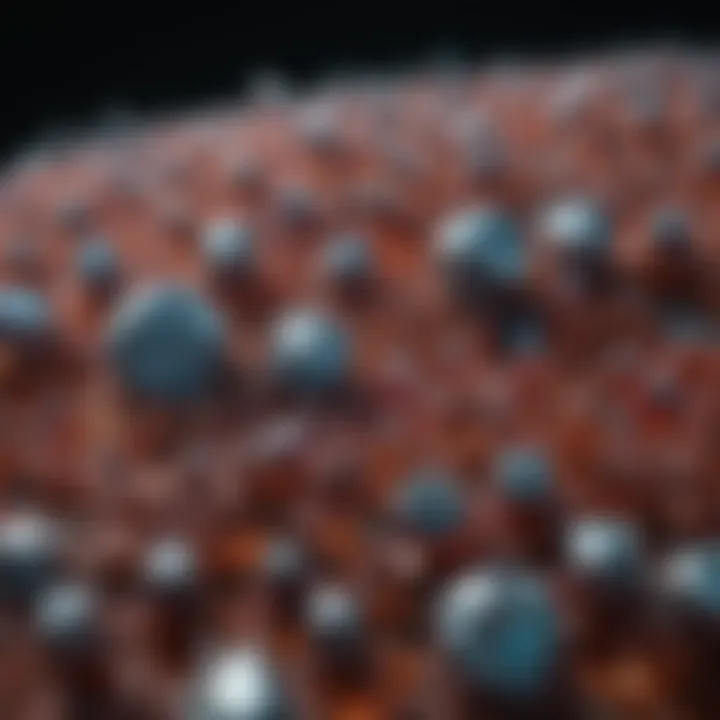
Overview of Research Topic
Brief Background and Context
Magnetic crystals have intrigued scientists for decades due to their unique properties and diverse applications. These materials possess intrinsic magnetic moments that can be influenced by external magnetic fields. The interplay between their atomic structure and magnetic ordering spices the study of condensed matter physics. By understanding the characteristics of these crystals, we not only uncover basic scientific principles but also pave the way for advancements in technology.
Importance in Current Scientific Landscape
The relevance of magnetic crystals cannot be overstated. In today’s technological age, their applications stretch across multiple fields, from electronics to medicine. Innovations in data storage, such as hard disk drives, rely heavily on magnetic materials to perform effectively. Furthermore, in quantum computing, magnetic crystals play a pivotal role in qubit systems, enabling faster and more efficient computations. Researchers continue to investigate their properties to exploit them in emerging domains like spintronics and nanotechnology.
Methodology
Research Design and Approach
This article employs a combination of theoretical and empirical approaches to explore magnetic crystals in depth. By first establishing a robust theoretical framework, we analyze foundational concepts such as ferromagnetism and antiferromagnetism. Subsequently, we shift focus towards hands-on experiments that solidify these theories, enabling practical insights into the behavior of these unique materials.
Data Collection Techniques
Data collection involves a variety of techniques, tailored to capture the fundamental properties of magnetic crystals.
- Magnetometry techniques are employed to measure magnetic properties and behaviors in response to different stimuli.
- X-ray diffraction serves as a principal method to determine the crystal structures, providing insight into arrangement of atoms.
- Scanning tunneling microscopy allows researchers to visualize the surface interactions at atomic levels.
The integration of these methods helps paint a thorough picture of magnetic crystals, ensuring that both theoretical insights and practical observations are discussed.
"The exploration of magnetic crystals not only deepens our understanding of material science but also reconnects various scientific fields such as condensed matter physics, materials engineering, and applied magnetism."
As we delve further into the world of magnetic crystals, each topic discussed will highlight their essential features, classifications, and a variety of applications, establishing a comprehensive guide for the academically inclined and the practically focused.
Prelude to Magnetic Crystals
Understanding magnetic crystals is crucial in diverse fields such as material science, physics, and engineering. These crystals exhibit unique magnetic properties, making them valuable for various applications, from data storage to medical tools. Moreover, the study of magnetic crystals opens doors to advancements in nanotechnology and quantum computing.
An in-depth examination of the underlying principles behind these materials can lead to innovations that enhance technology. This section aims to provide foundational knowledge that informs the subsequent sections of this article. A clear comprehension of magnetic crystals sets the stage for discussions about their fundamental properties, classifications, and applications.
Definition of Magnetic Crystals
Magnetic crystals are solid materials whose atomic structure allows them to exhibit magnetic properties. These properties can include attraction to magnets and the capability to be magnetized. The phenomena arise due to the alignment of magnetic moments, associated with the electron spins within the crystal structure. The ability of these materials to do this makes them essential in industrial and scientific applications.
When classifying magnetic crystals, it is important to categorize them based on their magnetic behavior. Three principal magnetism types—diamagnetism, paramagnetism, and ferromagnetism—characterize the different response of these materials under magnetic fields. Understanding these definitions is essential for grasping how these materials behave in practical situations.
Historical Background
The study of magnetic crystals has a rich history that dates back several centuries. Early investigations into magnetism were primarily philosophical, with notable contributors like Aristotle, who speculated about the nature of magnetism. It wasn't until the 19th century that systematic experimentation began to yield more precise insights.
One pivotal figure was James Clerk Maxwell, who, in the mid-1800s, formulated theories about electromagnetism. His work laid the groundwork for understanding the relationship between electricity and magnetism. Later, Henri Becquerel and Pierre Curie further expanded knowledge by exploring magnetic properties in deeper ways.
The 20th century witnessed a surge in research surrounding magnetic materials, especially during and post-World War II. Advances in technology allowed for more sophisticated experimental techniques, leading to significant breakthroughs in the characterization of magnetic properties. This historical development helped pave the way for contemporary applications, such as in electronics and medical imaging.
Fundamental Properties of Magnetic Crystals
The fundamental properties of magnetic crystals form the core of their functionality and application in various scientific fields. These properties are integral to the understanding of magnetism at a microscopic level and influence how these materials can be utilized in technology and research. By examining aspects such as magnetization, types of magnetism, and crystal structures, one can appreciate the diverse behaviors exhibited by magnetic crystals and how they interact with external magnetic fields.
Magnetization and Its Measurement
Magnetization refers to the degree of magnetization a magnetic material can achieve when subjected to an external magnetic field. It is a central concept in the study of magnetic crystals, as it quantifies the density of magnetic moments in the material. Measurement of magnetization can be conducted through various techniques, such as magnetometry, which provides valuable insights into the properties of magnetic materials. Accurate measurement is critical for understanding behaviors like saturation magnetization, remanence, and coercivity which define a material's magnetic capabilities.
Types of Magnetism
Magnetism is classified into several types based on the material response to applied magnetic fields. Each type exhibits unique characteristics that warrant individual attention.
Diamagnetism
Diamagnetism is a form of magnetism that arises in materials where electrons tend to oppose an external magnetic field by generating a weak magnetic field of their own. The key characteristic of diamagnetic materials is their lack of permanent magnetization; they are generally repelled by magnetic fields. This property makes diamagnetism a popular choice for specific applications such as magnetic levitation and magnetic resonance imaging (MRI). One unique feature of diamagnetic materials is their uniform response regardless of the field strength, which provides a stable characteristic in contrast to other forms of magnetism.
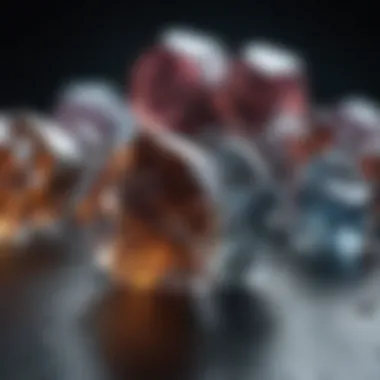
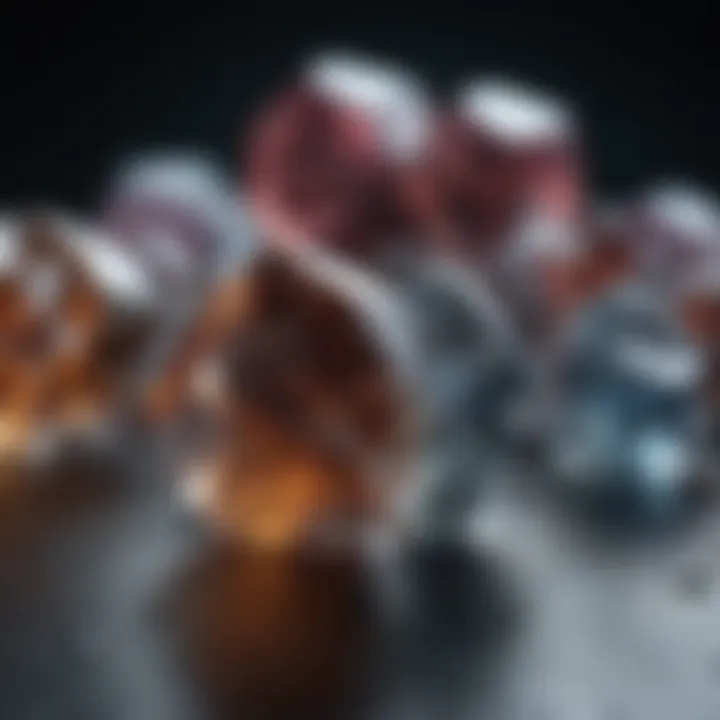
Paramagnetism
Paramagnetism occurs in materials with unpaired electrons that align with an external magnetic field, increasing the material’s overall magnetization. This characteristic makes paramagnetism an important phenomenon in both magnetic devices and research applications. However, its response is temporary and directly dependent on the strengths of the applied fields. The advantage here lies in the sensitivity of paramagnetic materials to changes in field strength, allowing for precise measurements in various scientific applications.
Ferromagnetism
Ferromagnetism is among the most recognizable forms of magnetism. It is characterized by the alignment of magnetic moments in the same direction, leading to strong magnetization even in the absence of an external field. This strength makes ferromagnetic materials essential for creating permanent magnets and storage devices. A distinct property of ferromagnetism is the hysteresis loop, which describes the relationship between induced magnetization and the external magnetic field. This aspect is both an advantage, providing consistent magnetic performance, and a disadvantage, as it can lead to energy losses in certain applications.
Antiferromagnetism
Antiferromagnetism describes a magnetic structure where adjacent moments align in opposite directions, resulting in no net macroscopic magnetization. This feature makes antiferromagnetic materials particularly interesting for use in applications such as spintronics, where the control of electron spin is crucial. Despite the lack of net magnetization, the unique interactions involved in antiferromagnetic ordering offer potential benefits, including improved performance in magnetic memory devices. However, the challenge lies in their sensitivity to temperature and external fields, which can disrupt the delicate balance of moment alignment.
Crystal Structures
The crystal structure of magnetic materials significantly affects their magnetic properties. Various lattice configurations can lead to different magnetic behaviors, making the study of crystal structures an important aspect of magnetic crystal research. The arrangement of atoms in a crystal affects how closely magnetic moments are able to interact, influencing magnetization and overall performance in applications. By understanding different crystal structures—ranging from simple cubic to more complex hexagonal arrangements—scientists can predict and manipulate the magnetic properties of materials more effectively.
Understanding the fundamental properties of magnetic crystals is crucial for advancing both academic and practical applications within numerous fields.
Classifications of Magnetic Crystals
Understanding the classifications of magnetic crystals is essential for grasping their diverse behaviors, properties, and applications. The classification not only reflects the intrinsic physical characteristics of the crystals but also aids in selecting suitable materials for specific technological applications. Moreover, knowing the differences between these classifications can inform researchers about potential avenues for further exploration and innovation.
There are primarily two notable categories in this context: single-domain and multi-domain crystals, as well as isotropic and anisotropic magnetic crystals. Each of these categories exhibits unique magnetic behaviors, which are critical for their applications in various fields, such as data storage and sensors.
Single-Domain and Multi-Domain Crystals
Single-domain crystals possess a uniform magnetic direction throughout the entire material. This means that all magnetic moments are aligned in the same direction. Such uniformity leads to more significant magnetic effects, often required in specific applications. These crystals are typically smaller in size compared to their multi-domain counterparts, which makes them easier to manipulate in certain scenarios. For example, single-domain particles are useful in biomedical applications such as magnetic resonance imaging and targeted drug delivery.
On the other hand, multi-domain crystals contain several magnetic domains, each with a different orientation of magnetic moments. The presence of multiple domains allows for more significant overall stability in larger structures. However, the complex interactions between these domains can also lead to diminished magnetic performance. Multi-domain crystals are widely found in various materials, especially in the manufacturing of permanent magnets and magnetic recording media.
"Single-domain crystals are crucial for applications needing consistent magnetic behavior, while multi-domain crystals offer stability in larger structures."
Isotropic and Anisotropic Magnetic Crystals
Isotropic magnetic crystals exhibit uniform magnetic properties in all directions. This means that their magnetic response does not change with the orientation of the applied magnetic field. Isotropic behavior simplifies practical applications since these materials show consistent performance regardless of their alignment or orientation.
In contrast, anisotropic magnetic crystals have directional dependence in their magnetic properties. This means their magnetic response varies based on the direction of the applied magnetic field. This characteristic can be advantageous in technologies where specific magnetic behaviors are desired. An example includes high-density magnetic data storage systems, which benefit from the controlled anisotropy of materials to enhance data retrieval accuracy.
The classification of magnetic crystals into single-domain and multi-domain, as well as isotropic and anisotropic, plays a critical role in their development and application in modern technologies. Understanding these classifications equips researchers and engineers with the information needed to select the right materials for their innovations.
Theoretical Framework
Understanding the theoretical framework of magnetic crystals is essential for grasping their complexities and real-world applications. This framework lays down the foundations upon which our comprehension of magnetism is built. It serves to unify various concepts from physics and materials science, providing insight into how magnetic properties arise at the atomic and molecular level.
In this section, we will discuss two crucial elements of the theoretical framework: quantum mechanics of magnetism and the Landau-Lifshitz-Gilbert equation. Each of these components plays a vital role in addressing how magnetic behaviors manifest in different materials, ultimately allowing for advancements in technology and scientific research.
Quantum Mechanics of Magnetism
Quantum mechanics provides a fundamental lens through which the behavior of magnetic materials can be understood. The magnetic moments of electrons originate from two distinct properties: their spin and orbital motion around atomic nuclei. Spin is an intrinsic form of angular momentum carried by electrons, which generates a magnetic moment. This is crucial in determining the way atoms and molecules interact magnetically.
The Pauli exclusion principle imposes restrictions on how electrons can occupy quantum states, leading to a variety of magnetic behaviors. For instance, in ferromagnetic materials, unpaired electrons align their spins parallel to each other, creating a strong overall magnetic moment. This collective behavior is absent in diamagnetic materials, where the electron spins tend to cancel each other out due to paired configurations.
Furthermore, quantum mechanics allows for the description of phenomena such as superparamagnetism. This occurs at the nanoscale, where thermal fluctuations can disrupt the alignment of magnetic moments, causing transient magnetization. Understanding these intricate behaviors is fundamental in the development of modern magnetic technologies.
Landau-Lifshitz-Gilbert Equation
The Landau-Lifshitz-Gilbert equation is a pivotal mathematical formulation in magnetism, detailing the time evolution of magnetization within magnetic materials. This equation combines Landau-Lifshitz dynamics with damping effects, enabling a comprehensive examination of magnetization's response to external magnetic fields.
The general form of the equation can be expressed as:
In this equation, M represents the magnetization vector, H the external magnetic field, γ the gyromagnetic ratio, and α the damping constant. The first term describes the precessional motion of the magnetization vector, while the second reflects the damping effects that cause the vector to align with the external field over time.
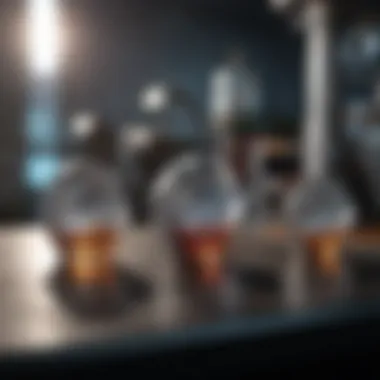
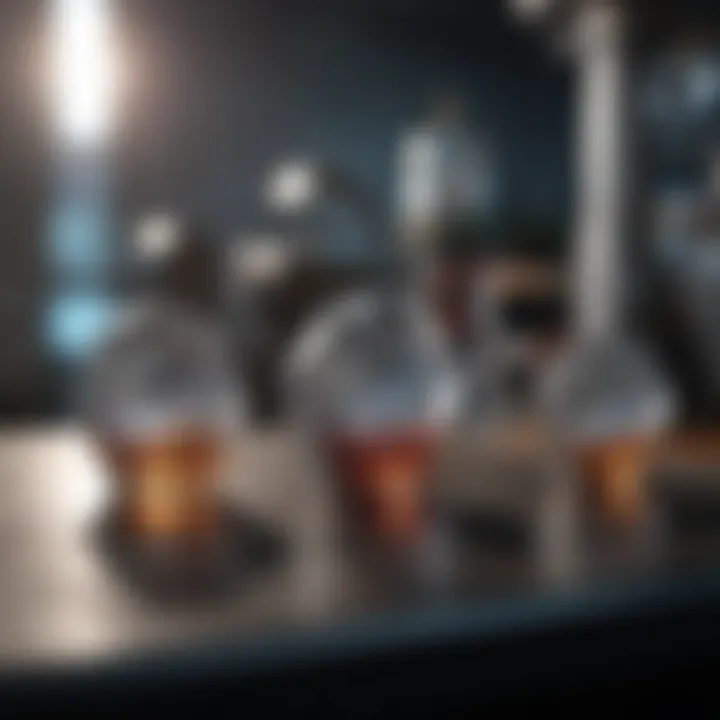
This equation is instrumental in describing processes such as magnetic switching, which is crucial for applications in data storage technology. Understanding the dynamics encoded in the Landau-Lifshitz-Gilbert equation enables researchers to better design materials with specific magnetic properties, thereby anticipating the behaviors of magnetic systems under various conditions.
The theoretical framework not only elucidates the principles underlying magnetic crystals but also enhances our capacity to innovate within fields reliant on magnetism.
In summary, the theoretical framework encompassing quantum mechanics and the Landau-Lifshitz-Gilbert equation is integral to the study of magnetic crystals. These concepts provide clarity on fundamental behaviors and phenomena, which are vital for numerous applications ranging from electronics to biomedical devices.
Experimental Techniques for Studying Magnetic Crystals
The study of magnetic crystals involves various experimental techniques. Understanding these techniques is crucial for gaining insights into the properties and behaviors of these materials. Different methods provide unique advantages, making it essential to choose the appropriate approach depending on the specific aspects of interest.
X-ray Diffraction
X-ray diffraction is a widely used technique for studying the structural properties of magnetic crystals. This method involves directing X-rays at a crystalline sample. When X-rays interact with the crystal lattice, they get diffracted at specific angles. By analyzing these angles and intensities, researchers can determine the crystal structure and identify phase transitions.
The importance of X-ray diffraction lies in its ability to elucidate the arrangement of atoms within a crystal. High-resolution x-ray diffraction can provide detailed information on lattice parameters, symmetry, and disorder. This data is vital for understanding how the atomic structure influences magnetic properties.
Magnetometry Methods
Magnetometry is another critical approach for studying magnetic crystals. It focuses on measuring the magnetic properties of materials. Two prominent techniques in this category include Vibrating Sample Magnetometry and SQUID Magnetometry.
Vibrating Sample Magnetometry
Vibrating Sample Magnetometry (VSM) is a highly effective method for measuring magnetic properties such as magnetization. This technique allows researchers to analyze samples by placing them in a uniform magnetic field. As the sample vibrates, it induces a voltage in pick-up coils. This voltage correlates directly with the sample's magnetization.
A key characteristic of VSM is its high sensitivity and versatility. It can measure small samples, making it popular for various types of magnetic materials, including thin films and nanoparticles. One unique feature is its ability to measure the magnetic response over a wide range of temperatures and magnetic fields, which provides essential data for understanding magnetic phenomena. However, VSM may struggle with non-homogeneous or bulk samples, limiting its application to specific research scenarios.
SQUID Magnetometry
Superconducting Quantum Interference Device (SQUID) Magnetometry is known for its exceptional sensitivity, capable of detecting even tiny changes in magnetization. This method employs superconducting loops to measure the magnetic signal. Because of its highly precise nature, SQUID Magnetometry is well-suited for weakly magnetic materials.
The key characteristic of SQUID Magnetometry lies in its flexibility, allowing it to operate at very low temperatures and in varying magnetic fields. This adaptability makes SQUID a preferred choice in advanced research focusing on fundamental magnetic phenomena, such as spintronics and quantum magnetism. One unique feature is its capability for time-resolved measurements, providing a dynamic view of magnetic behavior. However, the complexity and cost of SQUID systems can be prohibitive, making it less accessible than other techniques.
Neutron Scattering
Neutron scattering is an experimental technique that utilizes neutrons to probe the structure and dynamics of magnetic materials. This method is particularly effective for studying magnetic ordering and excitations, thanks to the sensitivity of neutrons to magnetic moments. Neutron scattering provides detailed insights into how magnetic atoms interact and align within a crystal lattice, thereby offering invaluable information regarding magnetic correlations and phase transitions.
Applications of Magnetic Crystals
The study of magnetic crystals extends far beyond theoretical underpinnings. Their applications are crucial across various fields. These include data storage, sensor technology, and biomedicine. Each of these applications highlights the benefit and versatility of magnetic crystals as well as the considerations for their use.
Data Storage Technologies
Magnetic crystals play a vital role in data storage technologies. Traditional hard disk drives utilize magnetic materials to store information. These materials can retain data for long periods without power. Newer technologies incorporate magnetic crystals for high-density storage solutions.
- Magnetoresistive Random Access Memory (MRAM): This technology uses magnetic crystals to store data. MRAM has faster read and write speeds compared to traditional memory types. It is also non-volatile, which means data is not lost when power is off.
- Hard Disk Drives (HDDs): Hard drives rely on magnetic crystal layers to record data. These layers allow for efficient data retrieval while providing longevity.
- Solid-State Drives (SSDs): Emerging technologies in SSDs also exploit the magnetic properties of certain materials to improve speed and stability.
Data storage technologies continue to evolve thanks to advances in magnetic crystals. This evolution enables larger capacities and faster access times.
Magnetic Sensors and Actuators
Magnetic sensors and actuators are critical in both industrial and consumer applications. Magnetic crystals enable precise measurements in various devices. Their low power consumption and durability make them attractive in many scenarios.
- Magnetoresistive Sensors: These sensors detect magnetic fields using the principle of magnetoresistance. They find applications in automotive systems, smartphones, and computers.
- Hall Effect Sensors: Used widely in electronic devices, Hall effect sensors utilize magnetic crystals to measure electric currents. This principle provides accurate positioning and speed detection.
- Actuators in Robotics: Magnetic actuators are essential in automating machinery and robotics. They provide reliable movement with minimal energy loss.
Magnetic crystals thus contribute to the reliability and efficiency of modern technology. Their integration into sensors and actuators has transformed how devices function.
Biomedical Applications
Biomedical engineering has benefited from advances in magnetic crystals. They serve crucial purposes, especially in imaging and therapy. Biocompatibility is essential in their usage, ensuring safety in medical applications.
- Magnetic Resonance Imaging (MRI): MRI machines rely on magnetic fields created by certain crystals. These fields provide detailed images of soft tissue, aiding in diagnostics.
- Targeted Drug Delivery: Magnetic nanoparticles can transport drugs directly to targeted areas within the body. This technique minimizes side effects and increases treatment efficiency.
- Hyperthermia Treatment: Magnetic nanoparticles are used in cancer treatment. Their ability to heat up in an alternating magnetic field targets malignant cells without harming healthy ones.
In medicine, the use of magnetic crystals opens doors to innovative therapies and enhanced imaging techniques.
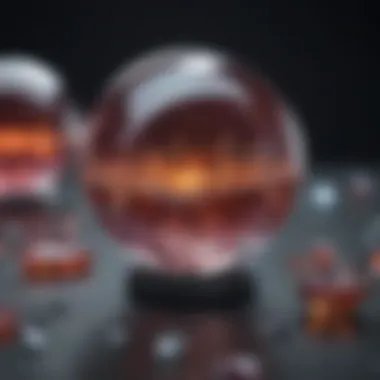
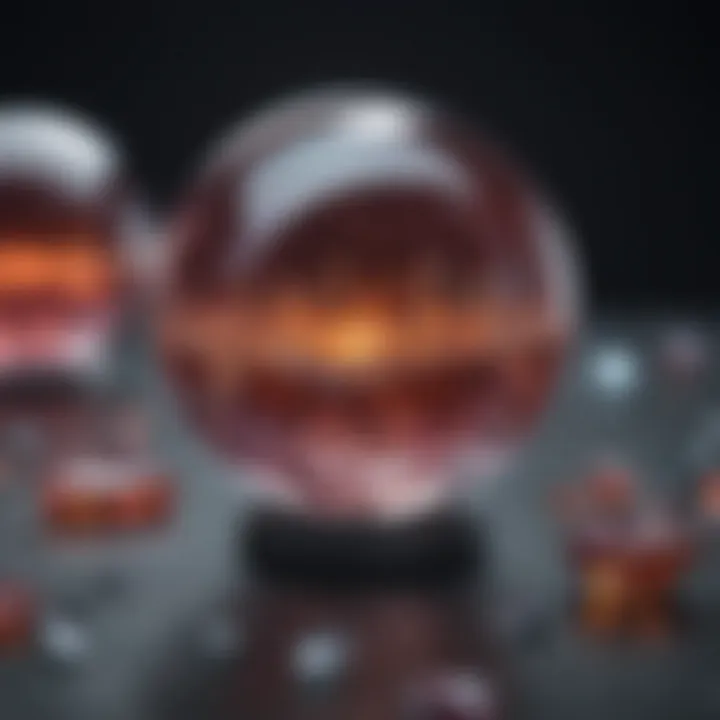
Magnetic crystals remain an area of rich potential, promising exciting developments across all sectors.
Challenges in Magnetic Crystals Research
Researching magnetic crystals comes with significant challenges that can impede progress and innovation. Understanding these challenges is crucial for advancing the field. This section will discuss the primary obstacles encountered in this realm, specifically focusing on material composition and synthesis, as well as thermal stability issues.
Material Composition and Synthesis
The composition of magnetic crystals directly influences their magnetic properties, which are critical for practical applications. Material composition involves a selection of elements that can optimize desired characteristics. In many cases, achieving an ideal composition requires intricate synthesis techniques. Common synthesis methods include sol-gel processing, hydrothermal synthesis, and vacuum deposition. Each method presents unique challenges.
For example, the sol-gel process offers simplicity and low-cost production, but it often results in inhomogeneities within the crystal structure. These inhomogeneities can negatively affect the magnetic properties of the final material, leading to unreliable performance in applications. Furthermore, controlling the particle size and shape during synthesis is crucial, as these factors influence magnetic behavior. Developing precise synthesis protocols is an ongoing challenge faced by researchers.
Some key considerations in overcoming material synthesis challenges include:
- Element selection: Choosing the right elements based on compatibility and favorable magnetic attributes.
- Controlled environment: Maintaining strict conditions such as temperature and pressure during synthesis.
- Characterization techniques: Employing advanced techniques to analyze material structures accurately.
Accomplished researchers continually work to innovate and refine these methods, indicating progress but also revealing the complexity of synthesizing high-quality magnetic crystals.
Thermal Stability Issues
Thermal stability is another decisive factor that impacts magnetic crystals, particularly when they are used in applications where temperature fluctuations are common. When exposed to high temperatures, some magnetic materials can undergo phase transitions that lead to undesirable changes in their magnetic properties. This occurrence can significantly degrade the performance of devices relying on magnetic crystals.
Maintaining thermal stability involves understanding the specific heat capacities and thermal expansion coefficients of the materials involved. Designers must create systems that are resilient against thermal degradation. One common approach to enhancing thermal stability is to develop composite materials that blend different components, which can distribute heat more effectively.
The following factors highlight key challenges in addressing thermal stability:
- Material degradation: Understanding how various magnetic materials respond to high temperatures over time.
- Heating mechanisms: Identifying the primary heating mechanisms at play when magnetic crystals are in operational environments.
- Mitigation strategies: Developing effective cooling solutions that can operate alongside magnetic crystals to prevent overheating.
"The relationship between thermal stability and magnetic property preservation is essential in designing next-generation magnetic materials."
Overall, challenges in magnetic crystal research underscore the need for innovative solutions and thorough exploration. Addressing issues in material composition and thermal stability not only enhances the performance of magnetic materials but also expands their potential applications in technology, science, and medicine.
Future Directions of Research
Research in magnetic crystals is on the brink of significant advancements. These developments are driven by the pursuit of newer, more efficient, and versatile materials that can fill specific roles in various applications. Evaluating future directions is critical, as it opens avenues for innovative solutions to both existing challenges and those yet to be presented in scientific and technological fields.
Emerging Magnetic Materials
Emerging magnetic materials, such as nanoscale and 2D materials, show great promise. Researchers are investigating materials like molybdenum disulfide (MoS₂) and iron diselenide (FeSe) for their unique magnetic properties. These materials could lead to breakthroughs in electronic devices. Their size and structure allow for increased functionality and adaptability in applications.
In addition, hybrid materials that combine organic and inorganic components are attracting attention. These materials present opportunities where traditional magnetic materials may fall short. The flexibility and low manufacturing cost of these new hybrids can drive down production expenses significantly, making them suitable for consumer electronics.
Integration with Quantum Technologies
The integration of magnetic crystals with quantum technologies represents a thrilling frontier. Quantum computing relies on robust and responsive materials capable of maintaining coherence over time. Magnetic materials that exhibit quantum states provide the groundwork for qubits, the basic units of quantum information.
Utilizing magnetic crystals in quantum systems emerges as a key factor in developing stable quantum components. Research focuses on optimizing how these materials interact within quantum environments. This will enhance the overall performance of quantum computers, paving the way for faster processing capabilities and more reliable algorithms.
"As we approach the intersection of magnetic materials and quantum technology, the future holds a potential for exponential growth in computational power."
End
The conclusion serves as a critical synthesis of insights gained throughout this exploration of magnetic crystals. It encapsulates the essential findings and underscores the relevance of magnetic crystals in both academic endeavors and real-world applications. By highlighting their unique properties, classifications, and varied applications, this article emphasizes the integral role magnetic crystals play in advancing technology and scientific knowledge.
Summary of Key Points
In summarizing the key points discussed, several elements stand out:
- Definition and Historical Significance: Understanding what constitutes a magnetic crystal and its evolution over time sets the foundation for further exploration.
- Fundamental Properties: The types of magnetism and their underlying crystal structures are vital for appreciating the behavior of magnetic materials.
- Classifications: Differentiating between single-domain and multi-domain crystals informs the understanding of their applications and behaviors under various conditions.
- Experimental Techniques: Methods like X-ray diffraction and magnetometry provide essential tools for analyzing these materials, facilitating advancements in research.
- Applications: The diverse fields utilizing magnetic crystals highlight their practical importance in modern technology, from data storage to biomedical innovations.
- Challenges and Future Directions: Recognizing the limitations of current research leads to informed predictions about emerging materials and their potential integration with quantum technologies.
This structured overview not only reinforces knowledge but also encourages ongoing inquiry into the field.
The Impact of Magnetic Crystals on Future Technologies
The future of technology is intricately linked to the progress in magnetic crystals research. The following points outline their potential impact:
- Emerging Materials: New magnetic materials are being developed constantly. These will enhance data storage capabilities and improve energy efficiency.
- Integration into Quantum Technologies: As research in quantum computing evolves, magnetic crystals could play a pivotal role in the development of qubits, thereby reshaping computational possibilities.
- Biomedical Advancements: Innovations in targeted drug delivery and magnetic resonance imaging could see significant improvements, benefiting countless patients globally.
- Environmental Applications: Magnetic materials could aid in pollution remediation by offering new methods for waste treatment and material recovery.
The exploration of magnetic crystals is not just an academic pursuit; it serves as a gateway to revolutionary advancements in technology and healthcare.