Iron Magnetism: Principles and Real-World Applications
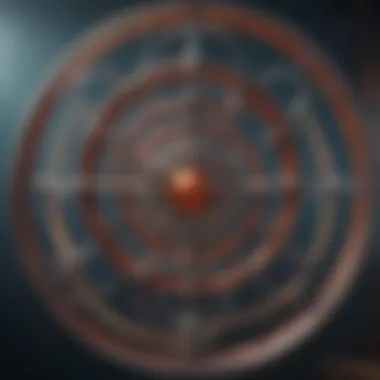
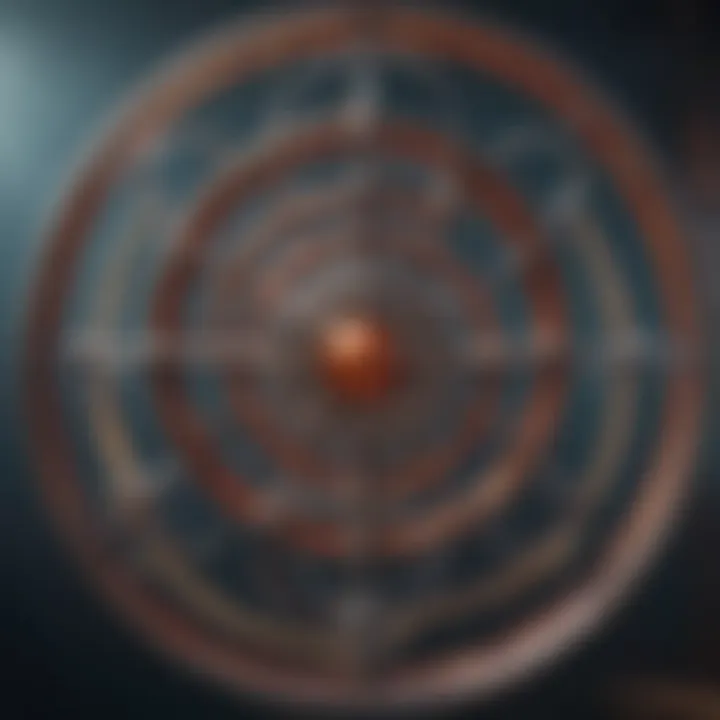
Intro
Iron magnetism represents a pivotal area in the field of electromagnetism and materials science. This phenomenon is not merely of academic interest but has profound implications across various technological domains. From information storage in computers to the functionality of electric motors, understanding iron magnetism is essential for both practical applications and theoretical advancements.
To fully grasp the significance of iron magnetism, one must explore the atomic structure that underlies these magnetic properties, the behavior of magnetic domains, and the impact of external influences. This exploration reveals not only the fundamental principles but also innovative applications in modern technology. It serves as a gateway to comprehending how materials interact with magnetic fields and how this interaction can be manipulated for practical use.
By delving into these aspects, this article aims to provide a comprehensive understanding of iron magnetism, guiding readers through its complexities and applications. It will serve as a valuable resource for students, researchers, and professionals who wish to deepen their knowledge and appreciate the role of iron in the magnetic landscape.
Prologue to Iron Magnetism
Iron magnetism represents a critical aspect of both physics and engineering, influencing the development of numerous technologies and driving innovations across various fields. Understanding iron magnetism is vital for students and professionals as it lays the foundation for the principles of electromagnetism and material science. By grasping this subject, readers gain insight into how magnetic properties emerge at the atomic level and how these properties can be manipulated in practical applications.
Defining Magnetism
Magnetism refers to the physical phenomenon that arises from the motion of electric charges. Specifically, it involves the forces exerted by magnetic fields on materials. Various forms of magnetism exist, including diamagnetism, paramagnetism, and ferromagnetism. In this article, we focus on ferromagnetism, the type of magnetism exhibited by iron and its alloys. Ferromagnetism is characterized by the alignment of magnetic moments in a material, resulting in a significant net magnetization.
Iron, as a ferromagnetic material, has unique properties that make it essential for various applications, such as transformers, electric motors, and data storage devices. The understanding of magnetism is not merely theoretical but has profound implications on technology and industry.
Historical Context
The journey of understanding magnetism began centuries ago. Early records of magnetic materials date back to ancient civilizations, where lodestones were observed to attract iron. However, the scientific exploration of magnetism gained momentum in the 19th century.
In 1820, Hans Christian Oersted discovered that electric currents create magnetic fields. This discovery established a crucial link between electricity and magnetism, leading to a deeper investigation into the nature of magnetic forces. Following Oersted, many scientists contributed to the understanding of magnetism. Michael Faraday, in the 1830s, explored electromagnetic induction, while James Clerk Maxwell formulated the theory that unified electricity and magnetism.
The study of iron magnetism continued into the 20th century, where advancements in quantum mechanics provided new insight into the behavior of magnets at the atomic level. Today, iron remains a cornerstone in the field of magnetism, influencing both theoretical research and practical applications.
Magnetic Properties of Iron
The magnetic properties of iron are crucial for understanding its behavior in various applications. Iron exhibits unique magnetic characteristics, primarily due to its electron configuration and crystal structure. These properties enable iron to serve as a key component in technologies ranging from electrical devices to industrial machinery. By exploring these properties, we can gain insights into both theoretical and practical implications of iron magnetism.
Ferromagnetism Explained
Ferromagnetism is the phenomenon that allows certain materials, like iron, to exhibit permanent magnetism. In ferromagnetic materials, atoms with unpaired electrons align their magnetic moments in the same direction, even without an external magnetic field. This alignment creates a net magnetic moment for the entire material.
When an external magnetic field is applied, these moments strengthen and orient along the field. Once the magnetic field is removed, the alignment can persist, making the material magnetically 'hard' or 'soft'. Hard ferromagnets, like those used in permanent magnets, retain their magnetism, while soft ferromagnets, used in transformers, can easily lose it. The temperature effects further complicate this behaviour, as higher temperatures can disrupt alignment, leading to a loss of magnetism.
Role of Electrons
Electrons play a critical role in the magnetic properties of iron. The arrangement of electrons within iron's atomic structure creates unpaired spins, giving rise to intrinsic magnetic moments. These moments interact with each other under specific conditions, resulting in the ferromagnetic behavior mentioned previously.
The electron configuration of iron, particularly its 3d and 4s electrons, contributes significantly to its magnetic properties. Additional factors include exchange interactions and spin alignment, both of which are influenced by external magnetic fields and temperature changes. Understanding the electrons' role is essential for applying iron magnetism in various technological fields, including data storage and magnetic sensing devices.
Crystal Structure of Iron
The crystal structure of iron significantly influences its magnetic behavior. Iron exists in two primary allotropes: body-centered cubic (BCC) at lower temperatures and face-centered cubic (FCC) at higher temperatures. Each allotrope has a distinct arrangement of atoms, leading to different magnetic properties.
In the BCC structure, the arrangement of iron atoms allows for higher magnetic anisotropy, which is the dependency of magnetic properties on direction. This can result in stronger magnetism in certain orientations. In contrast, the FCC structure tends to exhibit softer magnetic properties but allows for greater ease of magnetization.
The transformation between these structures due to temperature changes underlines the importance of understanding the crystal structure in applications. The unique behaviors of these structures continue to be a subject of research, contributing to advancements in materials science and technology.
"Understanding the interplay between electron behavior and crystal structure is fundamental to advancing iron's applications in technology."
Magnetic Domains
Magnetic domains are regions within ferromagnetic materials, such as iron, where the magnetic moments of atoms are aligned in the same direction. This alignment is crucial for understanding how magnetism works at the microscopic level. The concept of magnetic domains provides insight into why materials become magnetized and emphasizes the importance of each domain's contribution to the overall magnetic behavior of the material.
The formation, behavior, and movement of these domains can significantly influence the material's magnetic properties. Understanding magnetic domains helps in developing various applications, ranging from data storage to medical imaging.
Formation of Domains
When iron is magnetized, the process begins with the formation of magnetic domains. These domains are typically very small, ranging from micrometers to nanometers in size. Initially, in an unmagnetized state, the magnetic moments of atoms point in random directions, cancelling out any net magnetism.
Upon exposure to an external magnetic field, the domains can grow or shrink, with some aligning more closely to the field direction. This is primarily due to a reduction in energy. When domains align, the total magnetic moment of the material increases, enhancing its magnetic properties.
Influence of Temperature
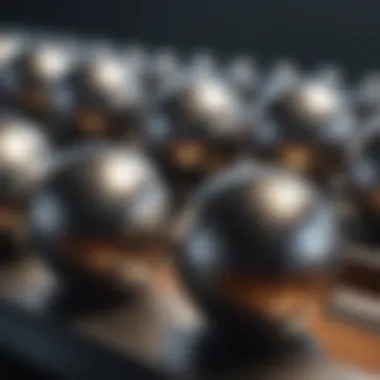
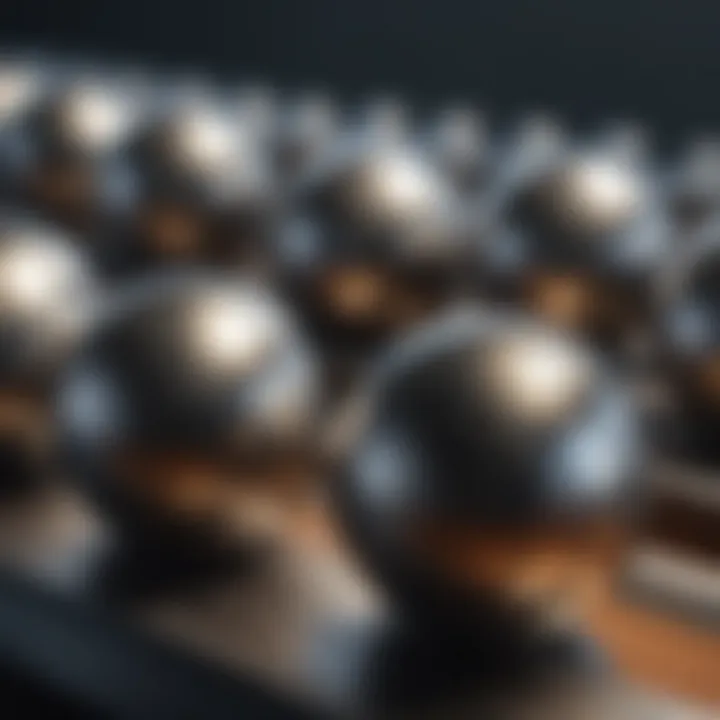
Temperature plays a crucial role in the behavior of magnetic domains. As the temperature rises, thermal agitation increases, causing the magnetic moments within the domains to misalign. This phenomenon can lead to a decrease in magnetization. A critical point in this context is the Curie temperature, the temperature at which a ferromagnetic material loses its magnetism and becomes paramagnetic.
Below the Curie temperature, the magnetic domains can reorganize and form upon cooling, resulting in re-magnetization. It is essential to consider temperature effects when designing applications that rely on stable magnetic properties.
Domain Wall Movement
Domain wall movement is another vital aspect of magnetic domains. The wall separates two different magnetic domains with distinct magnetization directions. When an external magnetic field is applied, these domain walls can move and change their position, leading to changes in the overall magnetic structure of the material.
This movement allows a material to be magnetized or demagnetized. It also means that the performance of devices such as electromagnets and transformers can be influenced by how easily domain walls move. A low energy barrier for domain wall movement is typically desired for efficient magnetic materials.
"Understanding magnetic domains is key to advancing technologies that rely on magnetic fields, from everyday electronics to advanced medical applications."
By studying magnetic domains, researchers can develop more effective magnetic materials and potentially discover new applications that harness the unique properties of magnetism. Overall, the exploration of magnetic domains is fundamental in both theoretical studies and practical technologies.
Measuring Magnetic Properties
Understanding the magnetic properties of iron is essential in various scientific and industrial applications. Measuring these properties provides insights into the behavior of iron under different conditions. This data is critical for designing efficient devices that rely on iron magnetism. It also aids in enhancing materials for improved performance and reliability.
Hysteresis Loop
The hysteresis loop is a graphical representation that illustrates the relationship between magnetic induction (B) and magnetic field strength (H) in a magnetic material. This loop reveals important characteristics about a material's magnetism, including its retentivity and coercivity.
- Retentivity: This measure indicates how much magnetization remains after an external magnetic field is removed. A material with high retentivity retains more magnetization.
- Coercivity: This reflects the required magnetic field intensity to reduce magnetization to zero.
The area within the hysteresis loop represents energy loss, which occurs during magnetization and demagnetization, highlighting inefficiencies in materials.
In practical applications, knowing these characteristics helps engineers select suitable materials for transformers and inductors.
Magnetic Susceptibility
Magnetic susceptibility is a measure of how much a material will become magnetized in an applied magnetic field. It plays a crucial role in determining how a substance responds to magnetic fields.
- Diamagnetic materials: These have negative susceptibility and do not retain magnetization.
- Paramagnetic materials: They have small positive susceptibility and align with magnetic fields but do not retain the magnetization after the field is removed.
- Ferromagnetic materials: Iron falls into this category, showing significant positive susceptibility and retaining magnetization.
The ability to quantify magnetic susceptibility allows researchers and engineers to predict how specific materials will behave under varying magnetic conditions, aiding in the development of high-performance magnetic devices.
Magnetization Techniques
There are several techniques for magnetizing iron materials, each with distinct advantages and applications. Common methods include:
- DC Magnetization: This involves applying a direct current to produce a strong magnetic field. It is simple and effective for various applications.
- AC Magnetization: Alternating current may be used to generate varying magnetic fields, useful in assessing how materials respond over time.
- Pulse Magnetization: This method applies rapid pulses of current, creating stronger and quicker magnetization effects.
Each technique explores different aspects of iron's magnetic properties. Choosing the right method depends on the application's requirements and the material's characteristics.
"Understanding how to measure magnetic properties informs better industrial practices and product design, fostering advancements across technology sectors."
External Influences on Iron Magnetism
The concept of external influences on iron magnetism is crucial for understanding how magnetic properties can be affected by various factors. These influences can dramatically alter the behavior of magnetic materials, leading to significant changes in their applications. An examination of these elements reveals how real-world conditions impact iron's magnetism, thus broadening the scope of potential applications in technology and industry.
Impact of External Fields
External magnetic fields serve as a primary influence on the magnetism of iron. When iron is subjected to these fields, its magnetic domains align in the direction of the external stimulus. This alignment increases the overall magnetization of the material. The degree to which this magnetization can be achieved depends on several factors, including the strength of the external field and the temperature of the iron.
Iron's response to external fields is also governed by the material's intrinsic properties. For instance, at high temperatures, thermal agitation disrupts domain alignment. However, at lower temperatures, the domains can align more effectively, making the iron more magnetic.
"The ability of iron to respond to external magnetic fields makes it invaluable in various technologies, including electromagnets and transformers."
Strain Effects
Mechanical strain can induce changes in the magnetization of iron. When iron experiences stress, whether through bending, compressing, or pulling, its atomic structure may undergo alterations. These changes can affect domain boundaries, impacting how closely the magnetic domains can align with one another.
Additionally, strain can lead to the phenomenon known as magnetostrictive effects. This behavior describes the change in dimensions of ferromagnetic materials under the influence of a magnetic field. Notably, the magnitude of these effects is dependent on the type of strain and the specific characteristics of the iron used. Understanding strain effects is essential in applications like sensors and actuators, where precise control over magnetic properties is required.
Impurities and Alloying
The presence of impurities or alloying elements can significantly impact iron's magnetic properties. Even trace amounts of foreign elements can disrupt the uniform structure of iron and subsequently affect its magnetism. For instance, elements like carbon and manganese are known to alter the coercivity and retentivity of iron.
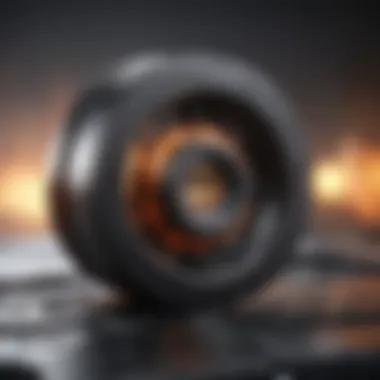
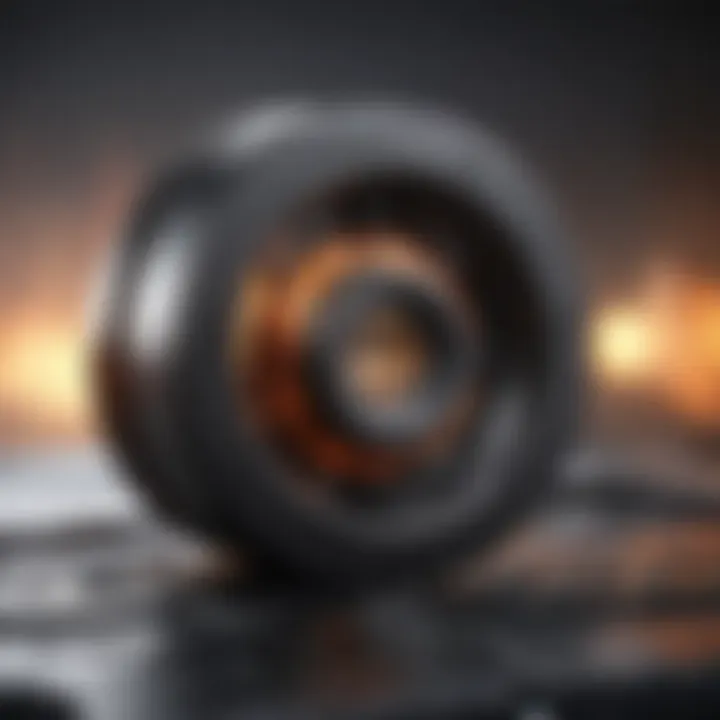
Alloying can enhance or diminish various magnetic characteristics. For example, adding nickel to iron contributes to the formation of a superlattice structure, which can improve magnetic performance. However, some impurities may induce magnetic frustration, where the magnetic interactions become complex and reduce the material's overall magnetization.
In industrial applications, controlling the purity and composition of iron is paramount. Manufacturers often engineer alloys to achieve desired magnetic properties suitable for specific uses, whether in automotive parts, electrical components, or magnetic recording media.
Applications of Iron Magnetism
The applications of iron magnetism are vast and integral to many aspects of modern technology. Iron is predominantly used for its magnetic properties in multiple fields. Understanding these applications not only highlights the practical significance of iron magnetism but also emphasizes its role as a cornerstone of numerous systems. This section will examine various domains where iron magnetism plays a crucial role, focusing on electromagnets, data storage devices, transformers, inductors, and medical applications.
Electromagnets
Electromagnets are pivotal in numerous applications, using the magnetic properties of iron to generate powerful magnetic fields when an electric current flows through. These devices feature in everything from electric motors to cranes that lift heavy metal objects. The magnetic field strength of an electromagnet is variable, allowing for control over its strength and polarity. This makes them essential for applications that require powerful and adjustable magnetic forces.
Key benefits of using electromagnets include:
- Controlled Magnetic Properties: The ability to adjust strength and direction.
- Size and Efficiency: Smaller and more efficient than permanent magnets.
- Versatile Applications: Found in electric bells, relays, and magnetic levitation systems.
Data Storage Devices
In the realm of electronics, data storage devices, such as hard disk drives (HDDs), heavily rely on iron magnetism. The data on these devices is stored using magnetic properties of iron-based alloys. Magnetic recording involves changing the magnetization of tiny regions on the disk's surface to represent binary data. The precision in magnetization not only defines data integrity but also influences storage density and accessibility speed.
The importance of iron magnetism in data storage encompasses multiple aspects:
- Data Retention: Magnetic properties aid in long-term data stability.
- High Density: Enables compact storage solutions that maximize space.
- Quick Access: Iron-based materials facilitate rapid read/write operations.
Transformers and Inductors
Transformers and inductors are electrical devices that utilize iron's magnetism to transfer or store energy efficiently. In transformers, alternating current passes through coils wrapped around an iron core. The core enhances the magnetic field, allowing for effective voltage transformation between circuits. Similarly, inductors store energy in the magnetic field generated around the conductor.
These devices demonstrate why iron magnetism is essential in electrical engineering:
- Energy Efficiency: Decreases energy losses during transmission.
- Current Regulation: Maintains smooth operation in AC circuits.
- Compact Design: The use of an iron core allows for smaller and more efficient devices.
Medical Applications
Iron magnetism extends into the medical field, primarily through magnetic resonance imaging (MRI) technology. The imaging technique employs strong magnetic fields generated by electromagnets to produce detailed images of organs and tissues within the body. The precision and clarity provided by these images can lead to better diagnosis and treatment options.
In summary, the relevance of iron magnetism in medicine includes:
- Higher Resolution Imaging: Enhances the clarity and detail of images for medical assessments.
- Non-Invasive Monitoring: Allows for the observation of internal structures without surgery.
- Research and Development: Aids in innovative treatments and understanding of diseases.
Iron magnetism is not just a theoretical concept; it forms the backbone of various technologies, making it indispensable in todayβs world.
By exploring these applications, it becomes evident that iron magnetism holds significant importance across multiple sectors. Its diverse applications continue to shape advancements in technology and improve everyday life.
Theoretical Models of Iron Magnetism
Understanding the theoretical models of iron magnetism is essential for grasping the underlying principles that govern its behavior. These models offer insights into how iron exhibits its magnetic properties and the interaction between its atomic structure and magnetism. Each theoretical perspective contributes to our comprehension of key phenomena associated with iron magnetism. It is notable that these models inform practical applications in technology and materials science.
Classical Approaches
Classical approaches to explaining iron magnetism primarily rely on macroscopic models that describe bulk magnetic behavior. Traditional theories, such as those based on classical electrodynamics, tend to focus on the effects of external magnetic fields on iron materials. The Langevin model, for example, presents a framework for understanding paramagnetism and diamagnetism within materials, including iron.
In ferromagnetic materials, the interaction between localized magnetic moments is crucial. The Weiss theory explains the concept of spontaneous magnetization, where regions of ferromagnetic materials can magnetize in the absence of an external magnetic field. Furthermore, these classical models provide a framework for understanding hysteresis and magnetic domains in iron.
Quantum Mechanics Perspective
Quantum mechanics adds a layer of complexity to the study of iron magnetism. It introduces the concept of electron spin as a fundamental property of electrons that contributes to magnetic behavior. The quantum mechanical model provides a deeper understanding of how electrons occupy atomic energy levels and how their spins interact within the crystal lattice of iron.
The Stoner model is an important quantum approach that addresses ferromagnetism via band theory. It suggests that the density of states at the Fermi level plays a significant role in determining the magnetic properties of a material. As a result, this model can explain the occurrence of ferromagnetism at certain electron concentrations, which is crucial for developing materials with desired magnetic characteristics.
Spintronics and Future Directions
Spintronics, or spin transport electronics, represents an innovative and emerging field that leverages the intrinsic spin of electrons, alongside their charge, to develop next-generation devices. This approach to iron magnetism unlocks new possibilities for data storage and processing technology, moving away from conventional methods that rely solely on electrical charge.
Researchers are focusing on how the spin of electrons can be manipulated to create more efficient memory devices, such as magnetic random-access memory (MRAM). This could lead to faster, more energy-efficient computing. The integration of spintronics principles could also enhance the functionality of existing devices while paving the way for novel applications in quantum computing.
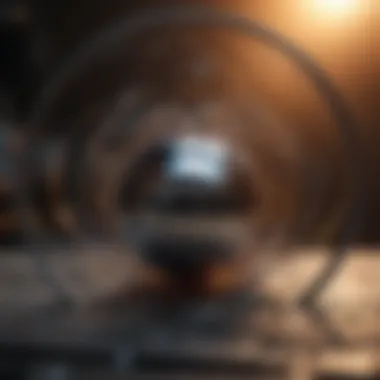
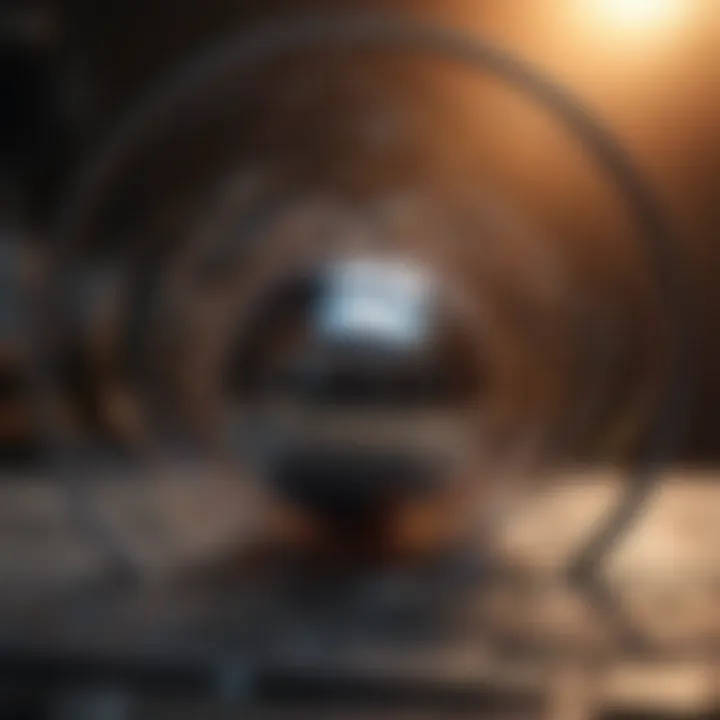
"The theoretical understanding of iron magnetism is not merely academic; it drives practical solutions in technology."
In summary, the theoretical models of iron magnetism offer essential perspectives for students, researchers, and professionals pursuing a deeper understanding of magnetic properties. The classical approaches establish foundational concepts while quantum mechanics refines our insights through electron interactions. The future of iron magnetism lies in spintronics, promising advancements that can reshape technology as we know it.
Challenges in Iron Magnetism Research
Research in iron magnetism presents a range of challenges that necessitate careful consideration and innovative solutions. Understanding these obstacles is crucial, as they can impact the effectiveness of research outcomes and the development of new technologies. Researchers face limitations in materials, difficulties in measurement, and other factors that may hinder progress.
Materials Limitations
One of the primary challenges in iron magnetism research comes from materials limitations. While iron is known for its strong magnetic properties, variations in purity and structure can significantly affect its magnetic behavior. Impurities in iron, such as carbon or sulfur, can alter the material's magnetic properties, leading to unexpected results in experiments.
The presence of alloys also poses a concern. Alloys often exhibit complex magnetic behaviors that could obscure the fundamental properties of iron itself. This requires researchers to isolate specific types of iron or create highly controlled environments to study these effects without interference.
Another aspect involves the availability of advanced materials that can offer new insights. For instance, the search for high-temperature superconductors or materials with enhanced magnetic characteristics drives the need for new strategies in synthesizing and characterizing magnetic materials. Without access to suitable materials, the exploration of iron magnetism can stagnate.
Measurement Difficulties
Measurement difficulties are another significant hurdle faced by scientists studying iron magnetism. Accurately measuring magnetic properties like susceptibility, coercivity, and saturation magnetization often requires sophisticated equipment and methodologies.
Instrument calibration is critical. Errors or inconsistencies in calibrating tools can lead to misleading data. Additionally, environmental factors such as temperature fluctuations can affect measurements. Precise control of experimental conditions is vital for obtaining reliable results.
Moreover, interpreting the data collected is not straightforward. Magnetic properties can be inherently nonlinear, necessitating complex mathematical models for proper analysis. Researchers must navigate these complexities to extract meaningful conclusions from their findings.
"The challenges in iron magnetism research are not just obstacles; they are opportunities for innovation and discovery. Tackling these issues can lead to breakthroughs in the understanding of magnetic materials."
Future Perspectives
The future of iron magnetism is a field laden with potential and opportunities. As industries evolve and technology advances, the significance of ironβs magnetic properties becomes increasingly apparent. Understanding these properties is crucial not only for improving existing technologies but also for paving the way for innovative applications in the fields of materials science and engineering. The exploration of future perspectives in iron magnetism focuses on advancements in materials science and innovations in technology. These elements will provide critical benefits to various sectors, including electronics, energy, and medical fields.
Advancements in Materials Science
In the realm of materials science, researchers are striving to develop new materials that enhance the magnetic properties of iron. Significant progress is being made in tailoring the composition of iron alloys. The addition of elements such as cobalt or nickel can lead to improvements in coercivity and saturation magnetization. This adaptability in materials allows engineers to design components that meet specific performance criteria more effectively.
Another focus is on nanostructured magnetic materials. These materials exhibit unique properties that differ from their bulk counterparts. For instance, they provide enhanced magnetic response and reduced hysteresis losses, making them ideal for high-frequency applications. Advances in synthesis techniques, such as sol-gel processing and chemical vapor deposition, are allowing for precise control over material structure and composition. This is crucial for optimizing performance, especially in applications requiring miniaturization.
The impact of renewable energy technologies also drives research in materials science. The shift towards sustainable energy solutions necessitates the development of magnetic materials that can operate efficiently in varied conditions. Thus, better understanding of how iron magnetism can be harnessed in renewable energy systems is vital.
Innovations in Technology
Technological innovation is closely linked to advancements in iron magnetism. The ability to manipulate magnetic properties opens up new vistas in various applications. In data storage technologies, for example, iron-based magnetic materials are used in hard drives and magnetic tapes. Innovations here lead towards greater data density and stability, offering considerable improvements in performance.
Moreover, in the medical field, iron magnetism plays a significant role. Magnetic resonance imaging (MRI) is a prime example. Researchers are investigating how enhanced magnetic materials can improve imaging techniques, leading to better diagnostics and treatments.
New developments in spintronics also hold promise for the future. Spintronics utilizes the intrinsic spin of electrons in addition to their charge to create devices that are faster and more efficient. Iron, due to its unique magnetic properties, remains at the heart of many spintronic devices, pushing the boundaries of performance in electronics.
Advancements in iron magnetism and materials science not only enhance practical applications but also fuel further research, creating a cycle of innovation and application.
In summary, the future perspectives regarding iron magnetism involve direct correlations to materials science advancements and technological innovation. These components are essential for creating smarter, more efficient systems across various industries, ensuring that iron magnetism remains a critical area of study.
Epilogue
Iron magnetism is a complex yet essential topic that carries significant weight in the fields of materials science and electromagnetism. This article has dissected various core aspects regarding the principles guiding iron's magnetic characteristics and the implications stemming from them.
Summary of Key Points
In summary, the exploration of iron magnetism encompasses several critical elements:
- Magnetic Properties: Understanding ferromagnetism and the role of electrons in magnetization.
- Magnetic Domains: How these domains form, their response to temperature shifts, and the dynamics of domain wall movement.
- Measuring Techniques: The significance of the hysteresis loop and the methods used to assess magnetic susceptibility.
- External Factors: The influence of external magnetic fields, strain effects, and the impact of impurities.
- Applications: A look into how iron magnetism is applied in electromagnets, data storage devices, transformers, and medical technologies.
- Challenges: Identifying the limitations surrounding materials and measurement difficulties.
- Future Perspectives: Examining advancements in materials science and potential innovations in technology.
Through this article, readers aimed to grasp a deeper understanding of how these factors interrelate and contribute to practical applications, thus broadening their perspective on the significance of iron magnetism in scientific and technological contexts.
Significance of Iron Magnetism
The significance of iron magnetism cannot be understated. It forms the bedrock for various technologies that impact daily life. From small-scale applications, such as magnetic sensors in smartphones, to large-scale implementations like transformers in electrical grids, the applications are immense.
Understanding iron magnetism illuminates pathways for innovations that may revolutionize materials used in electronics, renewable energy storage, and various advanced technologies.
As students, researchers, and professionals delve deeper into iron magnetism, they face challenges that encourage further exploration. This rich area of study not only helps in comprehensively understanding fundamental principles but also inspires new inventions that drive modern science and technology forward.
"The true beauty of iron magnetism lies in its ability to bridge theory and practical application, profoundly influencing countless technologies that define our modern world."
By synthesizing the information presented throughout this article, the reader prepares to engage with the complexities of iron magnetism not just as a concept, but as a vital component integral to future advancements.