Exploring Carbon Nanotube Wires: Structure and Applications
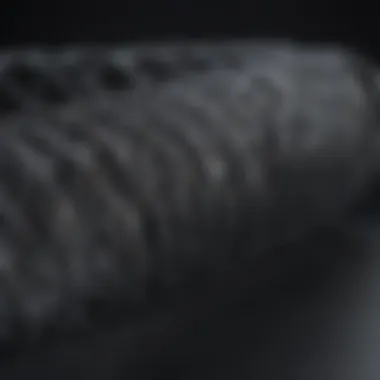
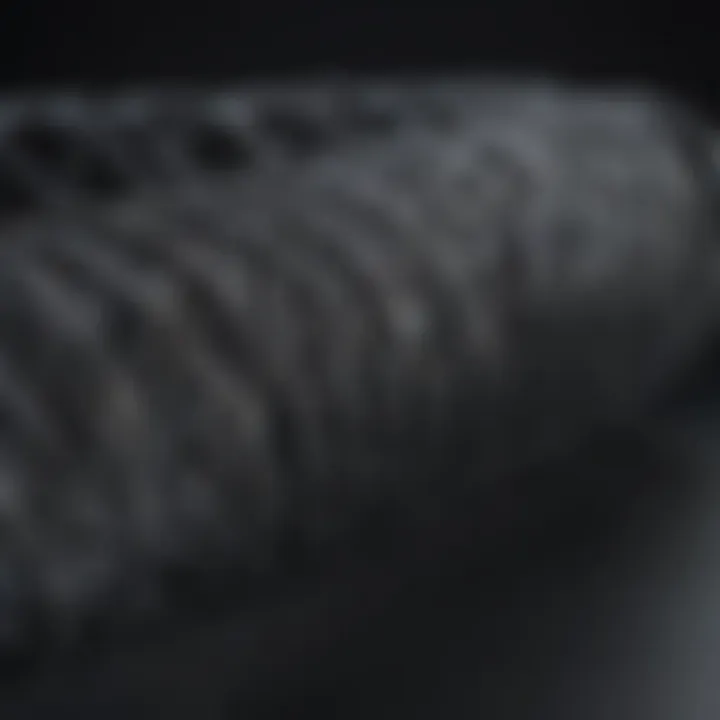
Intro
Carbon nanotube wires present a fascinating area of research in materials science and nanotechnology. These wires are characterized by their unique structural features, exceptional electrical properties, and extensive potential applications. Scientists have recently put substantial focus on carbon nanotube wires due to their possible innovations in various fields ranging from electronics to medicine. The investigation into their structure and properties requires in-depth understanding, which this article aims to provide.
Overview of Research Topic
Brief Background and Context
Carbon nanotube wires emerge from the remarkable properties of carbon nanotubes, which are cylindrical structures made of carbon atoms arranged in a hexagonal lattice. Discovered in the 1990s, they have gained popularity due to their mechanical strength, electrical conductivity, and thermal properties. These characteristics make them suitable for applications in sensors, batteries, and composite materials.
Importance in Current Scientific Landscape
The role of carbon nanotubes in advancing technology cannot be understated. Their unique structure enables new perspectives on nanoelectronics, energy storage, and even drug delivery systems. The versatility and efficiency of carbon nanotube wires are critical in addressing modern technological challenges. Researchers prioritize understanding these wires, given their potential to revolutionize industry standards.
Methodology
Research Design and Approach
To examine carbon nanotube wires effectively, a robust research design is essential. This includes experimental studies and theoretical simulations focusing on structural analysis, electrical characterization, and performance evaluation. Collaborative work among researchers, materials scientists, and engineers is often necessary to explore the diverse aspects of carbon nanotube wires thoroughly.
Data Collection Techniques
Data collection varies depending on the specific focus area within the research. Common methods include:
- Microscopy Techniques: Scanning Electron Microscopy (SEM) and Transmission Electron Microscopy (TEM) are used to observe structural features.
- Electrical Characterization: Techniques such as four-point probe measurements gauge electrical conductivity.
- Mechanical Testing: Tests like tensile strength measurements evaluate the mechanical properties.
These techniques enable researchers to gather substantial data, helping build a comprehensive understanding of carbon nanotube wires.
Carbon nanotube wires embody the future of materials science, linking theoretical research with practical applications across a spectrum of industries.
Prologue to Carbon Nanotube Wires
Carbon nanotube wires represent a significant advancement in material science. Their unique properties, such as exceptional strength and electrical conductivity, make them pivotal in numerous applications. With increased interest in sustainable technologies, the exploration of their capabilities is more relevant than ever. This article aims to provide a comprehensive overview of carbon nanotube wires, covering their structure, properties, manufacturing methods, and various applications. By delving into this subject, we can better understand how carbon nanotube wires contribute to technological innovation and sustainability.
Definition and Overview
Carbon nanotube wires are cylindrical nanostructures composed entirely of carbon atoms. These wires can be single-walled or multi-walled, depending on their construction. The unique arrangement of carbon atoms gives these wires remarkable mechanical, thermal, and electrical properties that are not found in traditional materials.
The diameter of carbon nanotube wires typically ranges from 1 nanometer to several nanometers, while their lengths can extend to several micrometers or more. Their electronic properties can be modified by changing their diameter and chirality, which defines how the carbon atoms are arranged. This flexibility allows for tailored applications in various fields, from electronics to energy storage and biotechnology.
The potential uses of carbon nanotube wires are extensive and include applications in high-strength materials, conductive composites, and nanodevices. Their lightweight nature combined with high conductivity makes them attractive for various industries, especially where performance and efficiency are critical.
Historical Context
The journey of carbon nanotube wires began in 1991 when Sumio Iijima discovered these structures while researching carbon materials for a different purpose. His findings opened a new field in nanotechnology, leading to extensive research on their properties and practical applications. The initial excitement generated by their discovery was palpable, yet it took several years for the scientific community to fully grasp their potential.
By the late 1990s, researchers began to investigate the electrical properties of carbon nanotube wires. Early studies revealed that these wires could conduct electricity nearly as well as copper, while offering much higher tensile strength. The ability to resist deformation under stress presented new possibilities for developing lightweight yet strong materials.
Over the past two decades, advancements in synthesis techniques have made it possible to produce carbon nanotube wires with greater efficiency and consistency. As research matured, various applications emerged in electronics, materials science, and even medicine. Today, the continued exploration of carbon nanotube wires holds promise for the development of next-generation technologies.
Structure of Carbon Nanotube Wires
Understanding the structure of carbon nanotube wires is fundamental to grasp their unique attributes and applications. The arrangement of carbon atoms in nanotubes results in several key benefits, including superior mechanical, thermal, and electrical properties. These attributes arise from the atomic composition and geometric configuration of the nanotubes, influencing their performance in various fields, from electronics to materials science. By focusing on the intricacies of their structure, we can better appreciate their versatility and functionality in advanced technologies.
Atomic Composition
Carbon nanotubes are primarily composed of carbon atoms arranged in a hexagonal lattice. This specific arrangement is crucial as it creates sp2 hybridized bonds, which offer exceptional strength and flexibility. The bonding structure allows nanotubes to endure stress while maintaining their shape and integrity. The density of the material also adds to their uniqueness, contributing to lightweight characteristics that are invaluable in many applications.
Another notable aspect of the atomic composition is the uniformity of these structures, which leads to consistent electrical and thermal conductivity. Understanding the composition is vital since small variations can significantly impact the properties and potential applications of carbon nanotube wires.
Types of Carbon Nanotubes
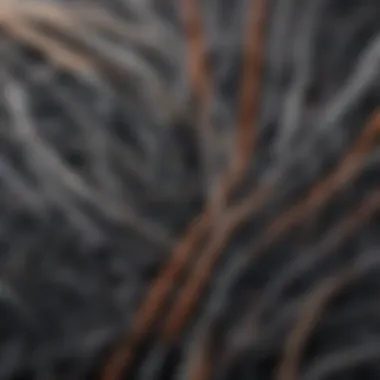
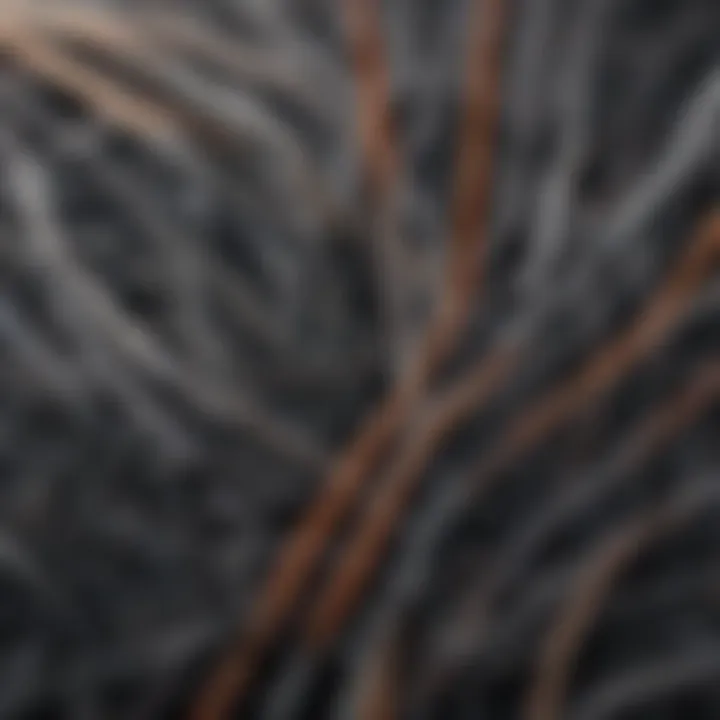
Single-walled Carbon Nanotubes
Single-walled carbon nanotubes (SWCNTs) consist of a single layer of carbon atoms rolled into a cylindrical shape. This simplicity is one of the main reasons they are favored in many research and application scenarios. SWCNTs exhibit excellent electrical properties, often functioning as metallic or semiconducting materials based on their chirality and diameter.
Key characteristics include a high surface area to volume ratio, making them ideal for applications in sensors and field-effect transistors. The unique feature of SWCNTs is their ability to conduct electricity efficiently, which is advantageous for electronic devices. However, their production can be challenging, and variability in quality can be a concern when scaling up manufacturing.
Multi-walled Carbon Nanotubes
Multi-walled carbon nanotubes (MWCNTs) consist of multiple layers of carbon atoms, forming concentric tubes. The primary characteristic of MWCNTs is their increased tensile strength and thermal stability due to the multiple layers. This makes them particularly useful in applications where durability is essential, such as composite materials and structural reinforcement.
MWCNTs also offer improved electrical conductivity compared to SWCNTs. A unique feature is the interaction between the layers, which can lead to enhanced mechanical properties. However, the complexity in their structure can sometimes lead to issues in consistency and reliability during production.
Alignment and Orientation
The alignment and orientation of carbon nanotube wires play a pivotal role in determining their effective application. A well-aligned nanotube can enhance electrical conductivity and mechanical strength, making the properties more uniform across a given material. This is particularly important in composite materials where nanotubes are integrated with other substances to form a composite matrix.
In summary, the structure of carbon nanotube wires includes the atomic composition, types of nanotubes, and their alignment. All these elements provide insights into why carbon nanotubes are considered revolutionary materials in modern science and technology.
Electrical Properties of Carbon Nanotube Wires
The electrical properties of carbon nanotube wires are critical to understanding their potential applications in various fields. These unique structures exhibit remarkable electrical conductivity, showing both metallic and semiconducting behavior depending on their configuration. The significance of these properties encompasses the advancement of electronics, energy storage, and other technologies, making them a topic of substantial interest in scientific research and industry.
Conductivity Mechanisms
Carbon nanotubes possess distinct conductivity mechanisms based on their structure. The essential feature lies in their band structure.
- Armchair Nanotubes: These have metallic properties and allow electrons to flow freely, providing excellent conductivity.
- Chiral and Zigzag Nanotubes: These exhibit semiconducting characteristics with an energy bandgap that varies with their diameter and chirality.
One notable aspect of conductivity in carbon nanotubes is the role of defects. Smaller defects can disrupt electron flow, reducing conductivity. However, the intrinsic high mobility of electrons in carbon nanotubes can often compensate for some defects. This behavior demonstrates their superior conductivity mechanisms compared to traditional conductive materials like copper.
Comparison with Traditional Conductors
When comparing carbon nanotube wires to traditional conductors, several advantages arise. Traditional conductors, such as copper and aluminum, are limited by their bulk nature and inherent resistivity. In contrast, carbon nanotubes present:
- Higher Conductivity: Due to their unique structure, carbon nanotubes can maintain higher conductivity levels.
- Lighter Weight: They are significantly lighter than conventional metals, making them attractive for use in lightweight applications.
- Flexibility: Unlike rigid metals, carbon nanotube wires offer greater flexibility without compromising their strength.
These factors lead to a potential revolution in many electronic applications where traditional conductors are commonly used. For instance, the integration of carbon nanotube wires in flexible electronics and lightweight wiring systems could enhance performance while reducing overall weight.
Potential for High-Frequency Applications
The potential for high-frequency applications is a pivotal advantage of carbon nanotube wires. Their high electron mobility allows them to operate effectively at frequencies far beyond the capabilities of conventional materials. In applications like radio frequency and high-speed data transmission, carbon nanotubes could be transformative.
Research indicates:
- Low Signal Loss: Carbon nanotube wires exhibit lower signal loss compared to traditional materials at high frequencies, which is crucial for maintaining signal integrity in telecommunications.
- High Thermal Stability: They can function effectively under high temperatures, maintaining performance in demanding environments.
This ability positions carbon nanotube wires as suitable candidates for next-generation devices in communications, radar systems, and more, as they can facilitate faster and more efficient electronic systems.
In summary, the electrical properties of carbon nanotube wires present substantial advantages over traditional conductors, highlighting their relevance in advancing technology across various fields.
Manufacturing Methods for Carbon Nanotube Wires
Understanding the manufacturing methods for carbon nanotube wires is essential as it directly influences their structural properties and potential applications. Each technique offers unique advantages and addresses specific challenges. The choice of manufacturing method impacts the scalability of production, the quality of the final product, and the cost-effectiveness of the process.
Chemical Vapor Deposition Techniques
Chemical vapor deposition (CVD) is one of the most common methods used to create carbon nanotube wires. This process involves the deposition of carbon-containing gases in a reaction chamber under controlled conditions. A substrate is placed inside the chamber where the gas interacts with the surface. This method allows for considerable control over the nanotube's diameter, length, and alignment.
The flexibility of CVD is a key benefit. It can produce both single-walled and multi-walled carbon nanotubes based on the specific gases and conditions used.
Additionally, CVD contributes to high purity and uniformity of the resulting wires, which is critical for applications in electronics and materials science.
Electrospinning Processes
Electrospinning is another innovative technique for producing carbon nanotube wires. This method involves using an electric field to draw a polymer solution containing carbon nanotubes into a fine fiber. As the fiber is drawn out, it solidifies, resulting in a nanostructured material. Electrospinning provides advantages such as the ability to create fibers with a controlled diameter and a high surface area-to-volume ratio. However, the challenge lies in achieving consistency in the material properties and integrating inorganic components efficiently.
Challenges in Production
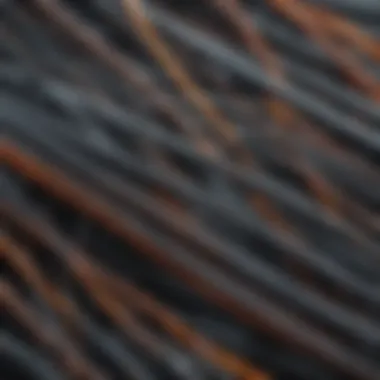
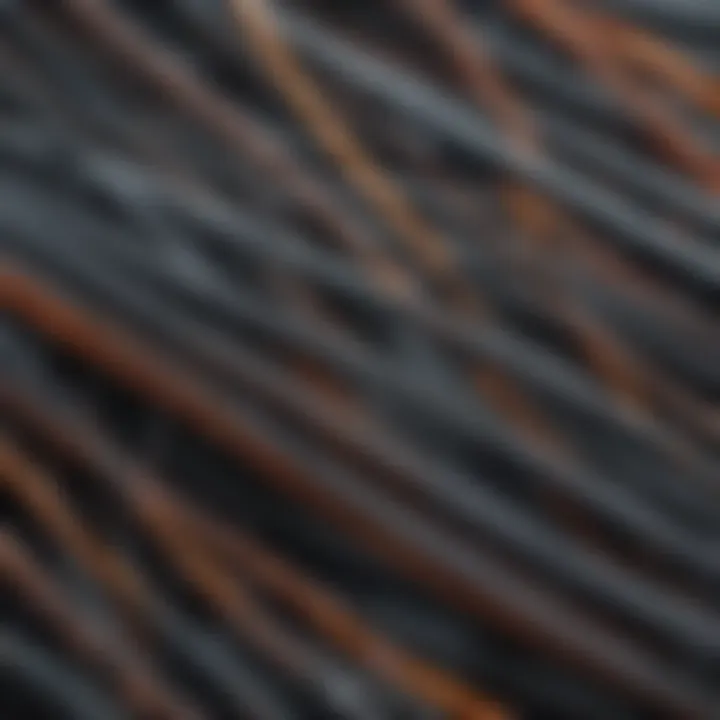
Scalability
Scalability is a critical aspect of manufacturing carbon nanotube wires. Achieving large-scale production while maintaining quality is a major concern. Several factors contribute to scalability. The choice of method must be able to handle increased production rates without sacrificing the properties of the nanotubes. For instance, while CVD can produce high-quality nanotubes, scaling it up to meet industrial demands requires careful consideration of energy consumption and raw material availability.
The unique feature of scalability lies in its ability to enhance the commercial viability of carbon nanotubes. This method can be a beneficial choice as it allows industries to meet the growing demand for superior materials effectively. The downside is the initial investment and technological adaptation required for mass production.
Quality Control
Quality control in the production of carbon nanotube wires is vital. It ensures that the end product meets the necessary specifications and performance criteria. Effective quality control involves monitoring the fabrication process to detect defects or inconsistencies early.
One key characteristic of quality control is consistency. Maintaining uniformity in the size and properties of the nanotubes is essential for their application in sensitive technologies. The unique feature of quality control is that it directly influences the reliability of the final applications, whether in electronics or biomedicine.
However, the rigorous testing required can increase production time and costs, presenting an ongoing challenge.
Improving manufacturing methods will significantly impact the future integration of carbon nanotube wires across various industries, enhancing both performance and sustainability.
Mechanical Properties of Carbon Nanotube Wires
The mechanical properties of carbon nanotube wires are crucial for understanding their potential applications and benefits. These properties dictate how these materials behave under different physical stresses and conditions. The exceptional mechanical characteristics of carbon nanotube wires make them suitable for a variety of fields, including electronics, nanocomposites, and biomedical applications.
Tensile Strength and Flexibility
Tensile strength refers to the capacity of a material to withstand being pulled apart. Carbon nanotube wires exhibit remarkably high tensile strength, often exceeding that of steel by a factor of several times. This property is largely due to their unique tubular structure, which allows for efficient load distribution across the entire length of the wire. The atomic arrangement within the nanotube makes it resistant to deformation, ensuring that it can endure significant stress without failing.
In addition to high tensile strength, flexibility is another important characteristic of carbon nanotube wires. This flexibility allows them to bend and twist without breaking, unlike many traditional materials that are brittle under similar conditions. The combination of these two properties—strength and flexibility—presents significant opportunities for innovation in various materials and applications.
Researchers have been exploring how to capitalize on these mechanical properties, especially for applications that require lightweight yet strong materials, such as in aerospace and automotive industries.
Impact Resistance
Impact resistance measures a material's ability to absorb energy upon impact without fracturing. Carbon nanotube wires demonstrate excellent impact resistance due to their molecular structure, which allows them to dissipate energy effectively. This property is particularly valuable in situations where materials are subject to sudden forces or shocks.
The inherent toughness of carbon nanotubes means they can be integrated into composite materials, enhancing their overall resilience. This application is particularly relevant in protective gear, construction materials, and various consumer products where both strength and impact resistance are necessary.
"The combination of high tensile strength and exceptional flexibility, along with impact resistance makes carbon nanotube wires unique for future applications."
By understanding these mechanical properties, researchers can better utilize carbon nanotube wires in developing innovative solutions that push the boundaries of current technology. The insights gained from studying these properties are crucial for future research efforts and the advancement of carbon nanotube applications across multiple sectors.
Applications of Carbon Nanotube Wires
Carbon nanotube wires hold significant promise across multiple industries due to their unique properties. Innovations in electronics, energy storage, nanocomposite materials, and biotechnology are greatly influenced by this material. The applications of carbon nanotube wires enhance the performance and efficiency of devices while addressing challenges in conventional conductive materials. Understanding these applications is crucial for both scientific advancement and industrial utilization.
Electronics and Conductive Polymers
In the realm of electronics, carbon nanotube wires are increasingly being utilized for their remarkable conductivity and flexibility. Their small diameter, combined with high conductivity, makes them ideal for interconnects in microelectronics. They enable the design of thinner, lighter devices without compromising performance. Moreover, when integrated into conductive polymers, carbon nanotubes can provide enhanced electrical properties, which are beneficial for various applications.
The unique alignment of carbon nanotube wires within polymer matrices improves electronic performance. This results in materials that are not only flexible but also maintain high electrical properties. Consequently, these composites are used in applications such as flexible displays and wearable electronics where traditional materials fall short.
Energy Storage and Conversion
This section can further be subdivided to discuss batteries, capacitors, and solar cells, two key aspects where carbon nanotube wires are making a substantial impact.
Batteries and Capacitors
Carbon nanotube wires find a crucial role in batteries and capacitors. Their high surface area allows for better ion transport, which increases the efficiency of energy storage. This characteristic makes them a popular choice for lithium-ion batteries, where they provide rapid charging and discharging. The incorporation of carbon nanotubes improves not only the capacity but also the lifespan of batteries.
However, the production cost can be a limiting factor. It is essential to balance quality and manufacturability. Despite this, the advantages of using carbon nanotube wires in batteries often outweigh the disadvantages, making them a compelling option in energy storage solutions.
Solar Cells
For solar cells, carbon nanotube wires serve as conductive pathways that facilitate charge transfer. This enhances the efficiency of the cell by allowing for quicker energy conversion. The lightweight nature of carbon nanotubes also means that they can be used in thin-film solar technologies, which is increasingly attractive for reducing the weight and cost of renewable energy systems.
On the downside, scaling up production for commercial use could present challenges. Yet, the benefits of enhanced performance make carbon nanotube wires a significant area of research in solar energy applications.
Nanocomposite Materials
The versatility of carbon nanotube wires extends to nanocomposite materials. When incorporated into other materials, they can significantly improve mechanical strength and thermal conductivity. This ability to enhance material properties is crucial in various industries, including aerospace and automotive, where weight reduction is vital without sacrificing performance. The application in coatings also provides anti-corrosion features, extending the lifecycle of materials in harsh environments.
Biotechnology and Medicine
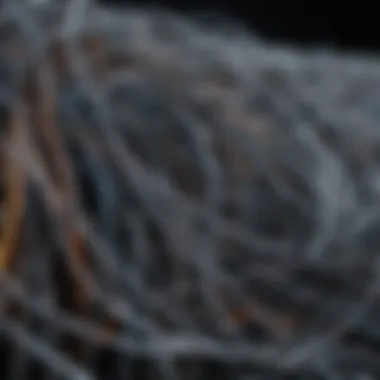
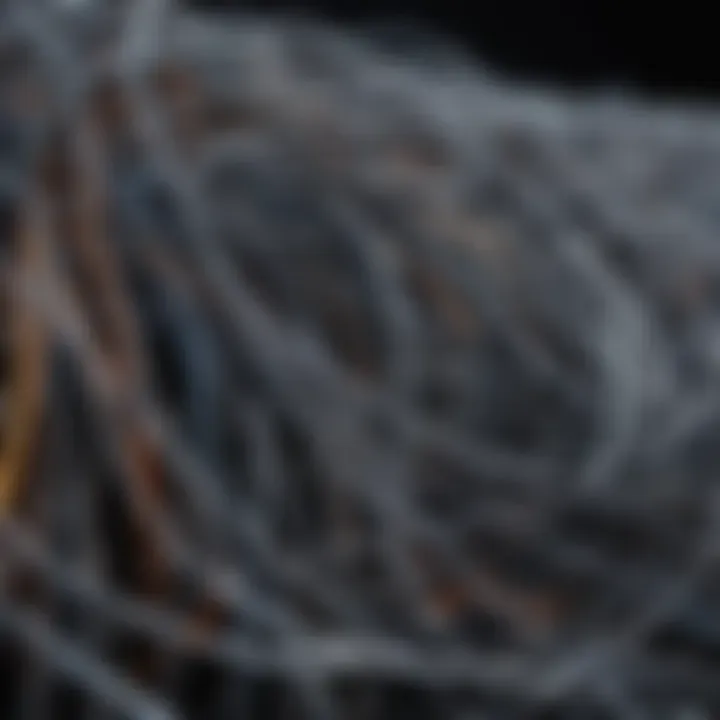
In biotechnology, carbon nanotube wires have the potential to transform medical diagnostics and drug delivery systems. Their unique properties allow for sensitive detection of biological markers. Moreover, due to their small size, they can easily penetrate cells, making them suitable for targeted drug delivery. Despite the potential, issues regarding biocompatibility and toxicity remain a concern, necessitating further research.
Carbon nanotube wires may enable advancements in medical applications, but careful consideration of their environmental and health impacts is essential.
Understanding the implications of carbon nanotube wires in these fields is essential. As research progresses, the prospects for innovative applications continue to expand, signaling a significant shift in materials science and technology.
Environmental Impact and Safety Concerns
The study of carbon nanotube wires raises significant questions related to environmental sustainability and human health. As the applications of these materials expand, understanding their impacts becomes essential for ensuring responsible use. Among the chief concerns are their toxicity, environmental effects during manufacturing, and potential risks associated with disposal and degradation. Evaluating these factors can guide researchers and industries towards safe practices.
Toxicity Studies
Toxicity studies focusing on carbon nanotubes have shown varying results based on several factors including the type of nanotube, exposure routes, and concentration levels. Research indicates that single-walled carbon nanotubes may exhibit different toxicological profiles when compared to multi-walled carbon nanotubes. Potential health risks include inflammation, oxidative stress, and even pulmonary issues upon inhalation.
Key points from toxicity studies include:
- Inhalation Risks: Some studies suggest that inhaled carbon nanotubes may cause respiratory problems, akin to traditional asbestos-related health issues.
- Effects on Cells: Laboratory studies demonstrate that carbon nanotubes can penetrate cellular membranes, potentially causing cytotoxicity.
- Skin Contact: Limited evidence indicates possible irritation from skin contact, though more research is needed.
While the full extent of risks is not entirely clear, continuous investigation is necessary. Researchers are advised to adopt stringent safety protocols during work with carbon nanotubes to minimize exposure.
Mitigation Strategies
To address the concerns surrounding the environmental impact and safety of carbon nanotube wires, a variety of mitigation strategies are being developed. These strategies aim to reduce potential hazards during both the manufacturing process and subsequent applications. Effective approaches include:
- Enhanced Manufacturing Protocols: Developing safer production methods can significantly lower the emissions of harmful substances. Techniques such as minimizing the use of toxic solvents and implementing closed systems can create a safer production environment.
- Proper Waste Disposal: Correct disposal methods of carbon nanotubes are critical. Industries must establish guidelines for the disposal of waste containing nanotubes, ensuring they do not enter landfills where they may degrade and leach harmful substances.
- Exposure Monitoring: Implementing rigorous monitoring of employee exposure in laboratories and industrial settings can help manage risks. Regular assessments can identify potential hazards before they escalate.
- Public Awareness: Educating both the public and professionals about the potential hazards associated with carbon nanotubes can facilitate responsible usage and promote safer practices within industries.
As research continues to shed light on carbon nanotubes, ongoing safety improvements will play a key role in their integration into various applications.
By adopting these strategies, the negative environmental impact of carbon nanotubes can be mitigated, ensuring their contribution to technology does not come at an unacceptable cost to human health or the environment.
Future Directions in Carbon Nanotube Wire Research
Research on carbon nanotube wires continues to evolve rapidly. The future holds significant potential for enhanced applications, novel techniques, and increased efficiency. Understanding these future directions is crucial for students, researchers, educators, and professionals interested in materials science and technology. The advancements in this field can impact many sectors, including electronics, energy, and even medicine.
Innovative Applications
Carbon nanotube wires are poised to enable a range of innovative applications. One area of focus is in nanoelectronics, where their excellent conductivity properties can lead to smaller and more efficient electronic components. Additionally, since carbon nanotubes possess unique mechanical properties, they can be integrated into lightweight composite materials, further enhancing performance in aerospace and automotive industries.
- Energy Harvesting Systems: There is an increasing interest in harnessing energy from ambient sources using carbon nanotube technology. This could lead to self-charging devices, which may transform how we approach the design of wearable electronics.
- Biomedical Devices: In those areas, carbon nanotubes show potential for drug delivery systems and biosensors. Their large surface area allows for improved binding interactions, increasing the efficiency of these devices.
Moreover, the adoption of carbon nanotube wires in flexible electronics is gaining traction. This flexibility opens avenues for applications in smart textiles and foldable devices, catering to consumer demands for versatility and portability.
Advancements in Manufacturing Techniques
As demand for carbon nanotube wires increases, so too does the need for more efficient manufacturing methods. Current techniques like chemical vapor deposition and electrospinning are fundamental, but improvements are needed to scale production while maintaining quality control. Innovations in this area could include:
- Roll-to-Roll Processing: This technique allows for large-scale production, making it more feasible to produce carbon nanotube wires in various applications. It could help bridge the gap between laboratory research and industrial scale production.
- In Situ Synthesis: Developing methods for synthesizing carbon nanotubes directly onto substrates would eliminate the need for transfer processes, thus improving the efficiency and viability for large-scale production.
"The future of carbon nanotube wires is not merely an evolution of existing techniques but rather a revolution in how we think about materials in various domains."
These advancements in manufacturing will help address some of the challenges associated with scalability and quality. As these techniques mature, they will make carbon nanotube wires more accessible to various industries and applications. The synergy between innovative applications and advancements in manufacturing is what will drive the next wave of research and application of carbon nanotube wires.
The End
The conclusion section serves as a significant point of synthesis in this article. It encapsulates the essence of carbon nanotube wires, reiterating their structural uniqueness, remarkable electrical properties, and the wide range of practical applications. This wrap-up not only provides clarity but also highlights the importance of understanding these advanced materials. By summarizing key findings, we allow readers to contextualize the relevance of carbon nanotubes in contemporary technological advancements.
Summary of Key Points
In this article, several core themes surrounding carbon nanotube wires were discussed:
- Definition and Structure: Carbon nanotubes boast a hexagonal arrangement of carbon atoms, which contributes to their strength and conductivity.
- Properties: Powered by exceptional electrical and mechanical properties, they offer superior performance compared to conventional materials.
- Applications: Their versatility spans various fields including electronics, energy storage, and biotechnology. This adaptability supports innovations in product design and efficiency.
- Manufacturing Challenges: Techniques like chemical vapor deposition and electrospinning have made production possible, yet scalability and quality control remain critical issues.
- Environmental Impact: Safety concerns are tied to their biological interactions, necessitating thorough toxicity studies and mitigation strategies.
Implications for Future Research
The path ahead for carbon nanotube wire research is promising. Potential areas for exploration include:
- Improved Synthesis Techniques: Advancements in manufacturing methods could enhance yield and reduce costs, making these materials more accessible.
- Expanded Applications: As understanding deepens, new uses may emerge, particularly in health care and environmental technologies.
- Long-term Environmental Studies: Ongoing research into the long-term effects and safe handling practices will be crucial.
- Integration with Other Materials: Combining carbon nanotubes with other nanoscale materials might open new avenues for innovative composite materials.
Understanding carbon nanotube wires is essential to leveraging their benefits in scientific research and industry. Future research is set to enhance their application, mitigate potential risks, and ultimately reshape various technological landscapes.