Amino Acid Structure: A Comprehensive Overview
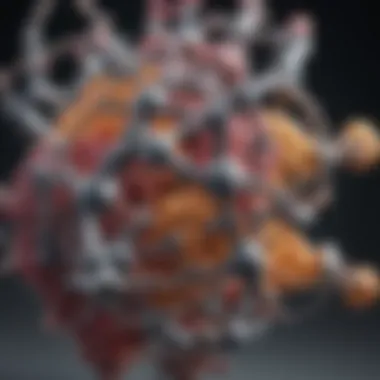
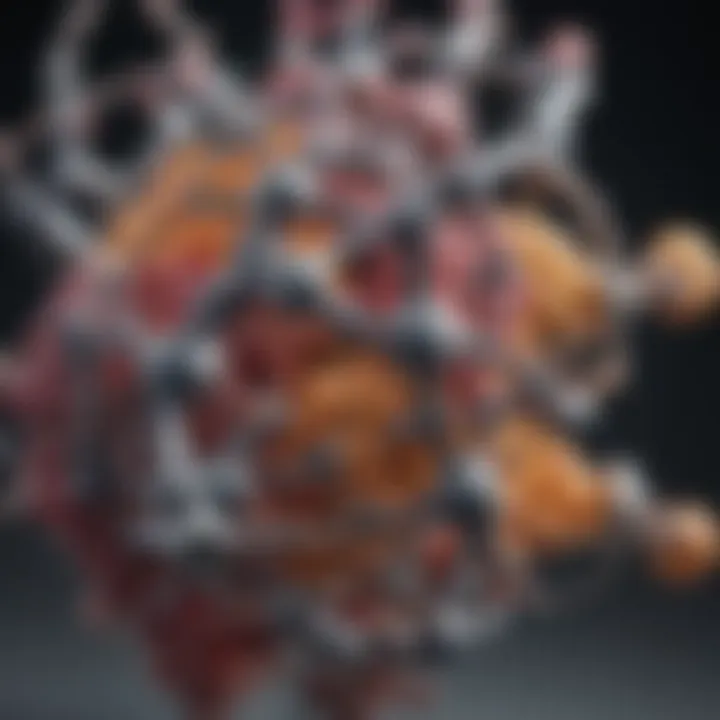
Intro
Amino acids serve as the cornerstone of biological life. They are not merely the subunits of proteins but play an essential role in various metabolic processes. Understanding their structure is pivotal in grasping how they function in tandem with each other and the overall biochemistry of living organisms.
The structure of amino acids consists of several key components: the central carbon atom, an amino group, a carboxyl group, a hydrogen atom, and a variable side chain known as the R group. Each amino acid possesses unique properties based on its side chain, influencing its role in protein formation and other cellular activities. This article aims to dissect these structures to enhance comprehension of amino acids' biological significance.
Overview of Research Topic
Brief Background and Context
Amino acids are organic molecules characterized by a core structure, a central carbon atom bound to an amino group (-N), a carboxyl group (-COOH), and a hydrogen atom. The diverse range of amino acids arises primarily through variations in the R group, which dictates their chemical behavior. Given that proteins are constitutive elements in cells, studying amino acid structures becomes crucial in numerous scientific domains, including biochemistry, molecular biology, and nutrition.
Importance in Current Scientific Landscape
The understanding of amino acids is pivotal in health sciences and medicine. They significantly influence various biological processes, including enzyme activity, hormone production, and neurotransmission. As research progresses, amino acids are also recognized for their roles in therapeutic applications. Categorizing amino acids into essential and non-essential helps elucidate their dietary significance. Essential amino acids must be obtained through food, while non-essential ones can be synthesized by the body. This distinction is vital for nutritional sciences, as it impacts dietary recommendations and health outcomes.
Methodology
Research Design and Approach
The exploration of amino acid structures employs a systematic approach, integrating theoretical knowledge with experimental data. This dual methodology includes structural analysis through techniques such as X-ray crystallography and nuclear magnetic resonance (NMR) spectroscopy to obtain a comprehensive view of amino acid formations and interactions.
Data Collection Techniques
Data on amino acids can be gathered from various primary sources, including:
- Laboratory research studies that provide insights into specific amino acids and their roles.
- Literature reviews that compile existing knowledge on amino acids and their significance in metabolism and protein synthesis.
- Databases, such as the Protein Data Bank, which offers structural data that aids in understanding the complexities of amino acids at a molecular level.
Understanding amino acids requires a multifaceted approach, focusing on both theoretical knowledge and empirical data to underscore their significance in biological processes. With the fundamental elements and structures clearly defined, the following sections will delve deeper into how these factors influence protein synthesis and overall cellular functions.
Prologue to Amino Acids
Amino acids form the foundation of biological systems. Their study is crucial for comprehending how proteins are synthesized and function within living organisms. Understanding amino acids is not merely an academic pursuit; it has practical implications across fields such as medicine, nutrition, and biochemistry.
Amino acids are organic compounds that serve as the building blocks of proteins. Each amino acid consists of a basic structure, but they differ at the molecular level through their side chains, known as R groups. This structural variability contributes to the diverse functions that proteins can perform in the body. An in-depth examination of amino acids reveals more than their chemical makeup; it underscores their central role in metabolic processes, cell signaling, and maintaining homeostasis.
In this section, we will define amino acids and provide a historical context that highlights their importance in scientific discovery.
Definition of Amino Acids
Amino acids are simple organic molecules that contain both an amino group (-N) and a carboxyl group (-COOH). This dual functionality allows them to act both as acids and bases, contributing to their significant role in biochemical reactions.
In biological terms, amino acids are classified into two main categories: essential and non-essential amino acids. Essential amino acids cannot be synthesized de novo by the body and must be obtained through diet. Non-essential amino acids can be produced internally; however, dietary intake still plays a role in optimal health and physiology. The structure of an amino acid typically includes:
- An alpha carbon
- An amino group
- A carboxyl group
- A hydrogen atom
- An R group, which varies among different amino acids
This precise arrangement of atoms leads to the extensive variations and functions of proteins.
History and Discovery
The discovery of amino acids dates back to the early 19th century. In 1806, the first amino acid, asparagine, was isolated from asparagus juice. The field of amino acid research expanded significantly with the later isolation of other amino acids and the determination of their structures.
By the early 20th century, scientists like Emil Fischer played a fundamental role in mapping the structures of amino acids and synthesizing them in the laboratory. Fischer’s work laid the groundwork for understanding how proteins are formed through peptide bonds between these amino acids. His contributions emphasized the importance of amino acids not only as individual molecules but also as critical components of proteins that carry out a myriad of biological functions.
Amino acids have evolved from a niche interest in organic chemistry to a central focus in molecular biology and genetics. As researchers delve deeper into the molecular functions of amino acids, the implications for health, disease treatment, and nutritional science continue to grow. Their significance warrants ongoing investigation and understanding among professionals and students alike.
Basic Structure of Amino Acids
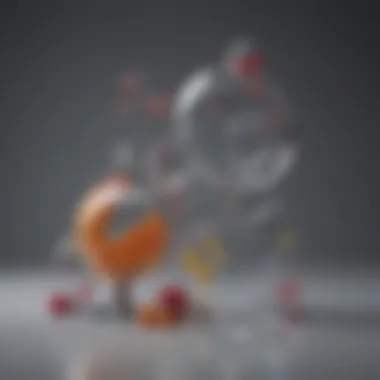
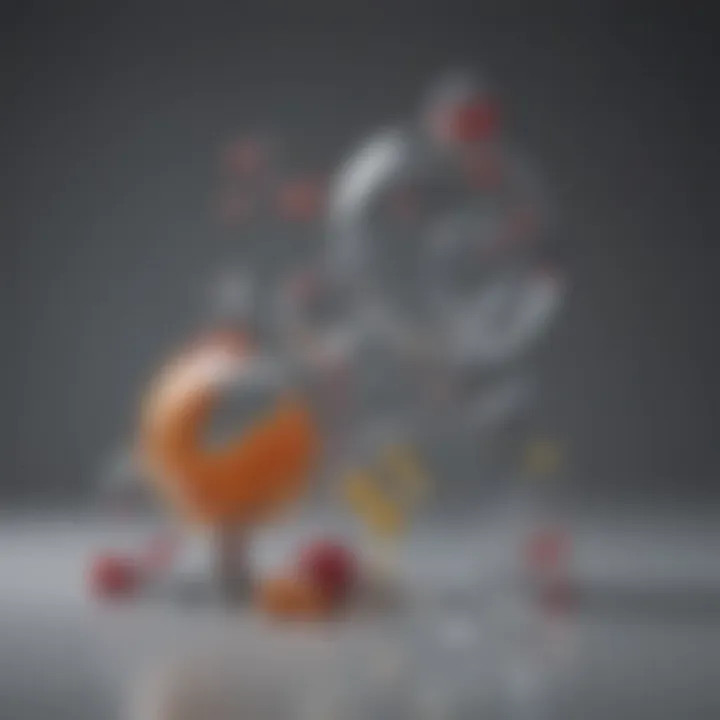
Understanding the basic structure of amino acids is crucial as it forms the foundation for how proteins are built and function in biological systems. Each amino acid consists of a core structure that includes an alpha carbon, an amine group, a carboxyl group, and a hydrogen atom. These components are essential because they directly influence the shape, stability, and reactivity of proteins. Furthermore, variations in the R group associated with each amino acid lead to the incredible diversity of proteins found in nature. This section systematically breaks down these core components, elucidating their significance in constructing the building blocks of life.
Core Components
Alpha Carbon
The alpha carbon is central to the structure of an amino acid. It is the chiral center that binds to four different groups: the amine group, the carboxyl group, a hydrogen atom, and the R group. The chiral nature of the alpha carbon means it can exist in two enantiomeric forms. This property is significant as it can influence the overall conformation of proteins. The unique feature of the alpha carbon is its ability to facilitate the structural characteristic of amino acids, allowing for the diverse configuration of proteins. This configurational flexibility is advantageous in terms of protein folding and function, allowing for various biological roles.
Amine Group
The amine group provides an essential nitrogen atom to the structure of an amino acid. This functional group participates in the formation of peptide bonds, which link amino acids together in the synthesis of proteins. The amine group is characterized by its basic nature; it can accept protons, impacting the overall charge of the amino acid in different pH environments. Its unique ability to interact with acids enhances its role in protein interactions and enzyme catalysis. This property is particularly beneficial for the stability of protein structures and facilitates various biochemical reactions that are vital to life.
Carboxyl Group
The carboxyl group plays a key role in defining the acidic character of an amino acid. This group contributes a carbonyl and hydroxyl, leading to a functional group important for forming peptide bonds when linking amino acids. The unique feature of the carboxyl group is its ability to ionize, donating a proton, which influences the overall charge of the amino acid in different environments. This behavior is advantageous as it affects the amino acid's solubility in water and its interactions with other molecules, reflecting its significance in metabolic processes.
Hydrogen Atom
The hydrogen atom, although seemingly simple, supports the structure and stability of the amino acid. It also contributes to the overall geometrical arrangement around the alpha carbon. While it does not carry a charge, the presence of the hydrogen atom allows the amino acid to maintain its integrity and reactability in biological conditions. This atom is crucial for maintaining the integrity of the amino acid backbone and plays a supportive role in the chemistry of amino acids.
R Group Variability
The R group, or side chain, dictates the unique characteristics of each amino acid, leading to their classification and functional diversity.
Definition of R Group
The R group refers to the variable side chain attached to the alpha carbon in amino acids. This side chain can vary in size, shape, charge, and polarity, defining the specific properties of each amino acid. The R group plays a pivotal role in determining how amino acids interact with each other and their environments. Its diverse nature allows for complex protein structures and functions, contributing to the vast array of biochemical processes.
Classification by R Group Properties
Classification based on R group properties divides amino acids into categories such as polar, non-polar, acidic, and basic. This classification is beneficial as it allows researchers to predict the behavior of amino acids in different environments, facilitating more effective studies in protein chemistry and biochemistry. Understanding these classifications helps to elucidate amino acid roles in metabolic pathways and their interactions within proteins.
Types of Amino Acids
Amino acids are classified based on their necessity for human health and their synthesis in the body. Understanding the different types of amino acids is crucial for various biological and nutritional applications. This knowledge aids not only in comprehension of protein synthesis but also in the dietary needs of individuals. Amino acids play versatile roles, from energy production to acting as precursors for critical biomolecules. Thus, each category of amino acids holds unique importance that can impact overall health outcomes.
Essential Amino Acids
Essential amino acids are those that cannot be synthesized by the body and must therefore be obtained through diet. There are nine essential amino acids: histidine, isoleucine, leucine, lysine, methionine, phenylalanine, threonine, tryptophan, and valine.
These amino acids are vital due to several factors:
- Dietary Requirements: Since the body lacks the ability to produce these amino acids, their presence in dietary sources such as meat, eggs, and dairy is necessary.
- Muscle Protein Synthesis: Essential amino acids play a critical role in muscle recovery and growth. For instance, leucine is widely recognized for its influence on protein synthesis, making it particularly important for athletes and active individuals.
"Essential amino acids are fundamental for protein synthesis and overall physical well-being."
- Hormonal Regulation: Some essential amino acids are precursors for hormones and neurotransmitters. For example, tryptophan is a precursor for serotonin. This illustrates their broader role beyond just protein production.
Non-Essential Amino Acids
Non-essential amino acids can be synthesized in the body and are thus not strictly required from dietary sources. There are 11 non-essential amino acids, including alanine, aspartic acid, and glutamic acid. Their significance includes:
- Biosynthesis: The body synthesizes these amino acids from essential amino acids and other substances, which allows for metabolic flexibility.
- Energy Metabolism: Non-essential amino acids can be converted into energy, especially during times of fasting or intense exercise. This adds an extra layer of utility in managing energy metabolism.
- Neurotransmitter Functions: Several non-essential amino acids serve as neurotransmitters or precursors. For example, glutamate is a key excitatory neurotransmitter in the brain.
Conditional Amino Acids
Conditional amino acids are generally considered non-essential but may become essential in specific conditions, such as illness or stress. Examples include arginine, cysteine, glutamine, tyrosine, glycine, proline, and serine. Their relevant features consist of:
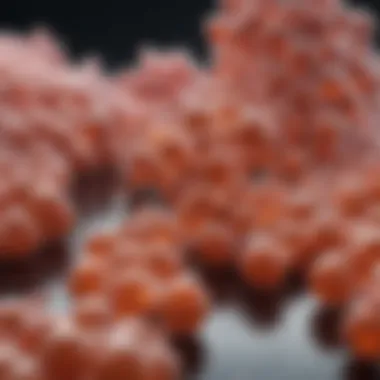
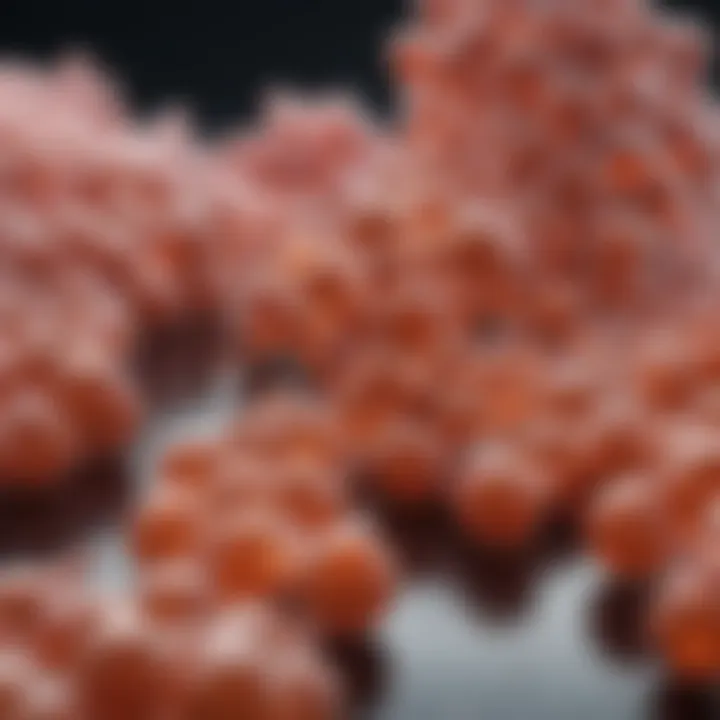
- Stress Response: During physical or emotional stress, the body's demand for certain amino acids increases. For instance, glutamine is crucial for immune function and is often supplemented in critically ill patients.
- Healing and Recovery: Arginine is important in wound healing and recovery post-surgery, indicating when nutrition must be personalized based on health conditions.
- Immune Function: Cysteine is involved in detoxification processes and is central to the synthesis of glutathione, a significant antioxidant.
In summary, the classification of amino acids into essential, non-essential, and conditional categories illustrates their diverse roles in health and nutrition. This understanding is not merely academic; it has practical implications for dietary planning, athletic performance, and medical interventions.
Peptide Bonds and Protein Structure
Peptide bonds are crucial to understanding amino acid interactions and their role in forming proteins. These bonds dictate how individual amino acids link together to create larger, functional molecules. Recognizing the structure of peptide bonds provides insight into the overall architecture of proteins, making it essential in the study of biochemistry and molecular biology.
Peptide bonds are formed through a dehydration synthesis reaction, where the carboxyl group of one amino acid reacts with the amine group of another. This process eliminates a molecule of water, resulting in a covalent bond. The creation of peptide bonds establishes the basis for protein chains, ultimately influencing protein stability and function.
Formation of Peptide Bonds
The formation of peptide bonds is not merely a chemical reaction; it is a fundamental process that underpins protein synthesis. When amino acids juxtapose, the interaction between their functional groups catalyzes the formation of peptide bonds. This bond is a primary linkage in proteins, creating a dipeptide and extending into longer polypeptide chains.
As these chains grow through successive peptide bond formations, the sequences of amino acids convey critical information for protein function. Any alteration in this sequence can lead to significant changes in protein behavior, illustrating the importance of peptide bond formation.
Levels of Protein Structure
Protein structure is categorized into four distinct levels: primary, secondary, tertiary, and quaternary. Each of these contributes uniquely to a protein's overall conformation and functionality.
Primary Structure
The primary structure refers to the unique sequence of amino acids in a polypeptide chain. This sequence is linear and is defined by genetic coding. The key characteristic of primary structure lies in its specificity; it determines how proteins fold and function.
A beneficial aspect of discussing primary structure is its direct correlation to genetic information. It serves as a foundational element for engineers and scientists aiming to understand genetic diseases. However, due to its linear nature, it does not account for the complex interactions that occur in higher structural levels, which must also be considered.
Secondary Structure
Secondary structure involves local folding patterns within the polypeptide chain, predominantly in the form of alpha helices and beta sheets. The hydrogen bonds between backbone atoms stabilize these formations. Secondary structure is a popular focus in protein studies because it provides an initial insight into the potential folding of proteins, paving the way for understanding their function.
Yet, secondary structures can sometimes create limitations, as they do not encompass the entire protein context. This narrow view can be misleading when trying to predict the entire structure's functionality.
Tertiary Structure
The tertiary structure represents the overall three-dimensional shape of a protein, resulting from the spatial arrangement of its secondary structures. This arrangement is stabilized by various interactions, such as hydrogen bonds, ionic bonds, and hydrophobic interactions. The key feature of tertiary structure is its complexity and the role it plays in enzyme-active sites. It is a beneficial discussion point because it relates closely to how proteins interact with other molecules.
Despite its advantages, tertiary structure can be sensitive to environmental changes like pH and temperature. Such factors can lead to denaturation, affecting protein activity.
Quaternary Structure
Quaternary structure involves the assembly of multiple polypeptide chains or subunits into a single functional protein. This structure is crucial for proteins that perform complex tasks requiring the cooperation of different polypeptides. The unique feature of quaternary structure lies in its capacity to create multi-functional entities. Each subunit can contribute distinct characteristics to the protein.
The quaternary structure is beneficial because it allows for dynamic regulation of protein function. However, this complexity can also introduce difficulties in understanding how individual units interact, warranting careful study.
Understanding the various levels of protein structure is essential for unraveling the complexities of biological processes and disease mechanisms.
Physiological Role of Amino Acids
Amino acids are fundamental players in numerous physiological processes within the body. Their roles reach far beyond being mere protein components; these organic compounds are involved in a variety of metabolic pathways and contribute significantly to cellular functions. Understanding the physiological role of amino acids helps to highlight their relevance in both health and disease. Without them, the intricate web of biological interactions would be incomplete.
Role in Protein Synthesis
Protein synthesis is one of the most vital functions facilitated by amino acids. During this process, amino acids are linked together in a specific sequence, dictated by the genetic code found in DNA. This sequence determines the structure and functionality of proteins, which are crucial for life.
- The synthesis occurs in two main stages: transcription and translation. In transcription, messenger RNA (mRNA) is created based on a DNA template.
- In translation, ribosomes read the mRNA and assemble amino acids in the correct order to form polypeptides.
The quality and availability of amino acids directly affect protein synthesis. For instance, essential amino acids must be obtained through diet, since the body cannot synthesize them. Their deficiency can impede protein synthesis, leading to various metabolic disorders.
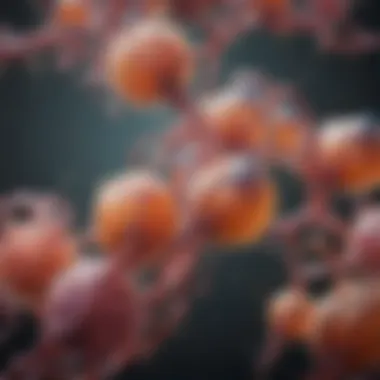
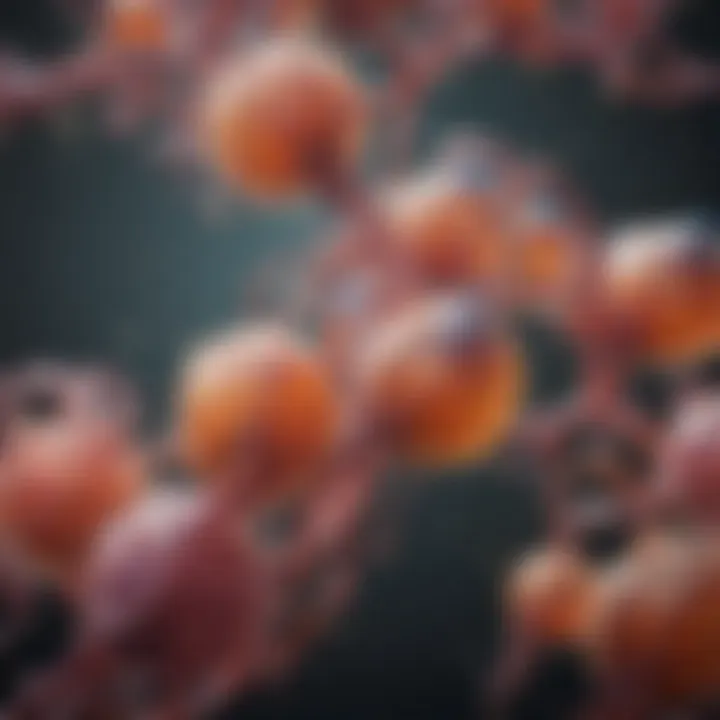
Metabolism of Amino Acids
Amino acid metabolism encompasses both catabolism and anabolism. These processes are essential for energy production and the synthesis of other important biomolecules.
Catabolism
Catabolism refers to the breakdown of amino acids for energy or conversion into other molecules. This process is crucial because it allows the body to utilize amino acids not just for protein production but also as an energy source when needed. The key characteristic of catabolism is its ability to generate energy during times of fasting or strenuous physical activity.
One unique feature of catabolism is its involvement in the urea cycle, which converts ammonia, a byproduct of amino acid breakdown, into urea for excretion. This mechanism prevents toxic accumulation in the body. The advantage of catabolism is its versatility, as it enables amino acids to participate in various metabolic pathways.
Anabolism
On the flip side, anabolism involves the synthesis of new compounds from amino acids, primarily targeting the formation of proteins and certain hormones. Anabolism is considered a beneficial choice in this article because it highlights both the preservation and utilization of amino acids in building cellular structures.
The unique feature of anabolism is its demand for energy, as this process requires ATP to create complex molecules. A potential disadvantage is the risk of imbalances when the supply of necessary amino acids is insufficient to meet anabolic needs, potentially halting processes like muscle repair and growth.
Significance in Cellular Functions
Amino acids are significantly involved in cellular functions including signaling, immune response, and cellular repair. They are not only structural components but also act as precursors for neurotransmitters, hormones, and other critical biomolecules. The balance of amino acids influences how cells communicate and respond to stimuli, ensuring proper physiological function.
In summary, the roles that amino acids play in protein synthesis, metabolism, and overall cellular health are paramount. Their physiological relevance cannot be overstated as they form the foundation for countless biological processes necessary for life.
Amino Acids in Health and Disease
Amino acids play a crucial role in numerous physiological processes and are fundamental to maintaining human health. Their involvement ranges from basic metabolic functions to more complex roles in disease prevention and treatment. Understanding how amino acids function in the context of health and disease enlightens the intricate relationship between nutrition and biochemistry. This section explores the implications of amino acid deficiency as well as their association with various disease states.
Deficiency and Impact on Health
Amino acid deficiency can lead to significant health issues. The human body requires a diversity of amino acids to carry out its functions effectively. Essential amino acids, which must be obtained through diet, are particularly important.
- Physical Health: A lack of essential amino acids can manifest as muscle wasting, impaired immune function, and hormonal imbalances. Individuals might experience fatigue, weakness, and a decrease in muscle mass.
- Mental Health: There is evidence that amino acid deficiencies may affect mood and cognitive function. For example, low levels of tryptophan, a precursor to serotonin, can lead to increased anxiety and depression.
- Growth and Development: In children, inadequate intake of these nutrients can impede growth and proper development. Research has shown that such deficiencies can result in stunted physical development and hinder cognitive skills.
In summary, maintaining a balanced intake of all amino acids is vital. Ensuring that individuals, especially athletes, children, and the elderly, consume sufficient protein is crucial to avoid these health challenges.
Amino Acids and Disease States
The relationship between amino acids and disease states is a field of study with growing significance. Specific amino acids can influence biological pathways that are critical in various conditions.
"Research indicates that the manipulation of amino acid levels could play a role in preventing or treating specific diseases."
- Cancer: Some studies suggest that certain amino acids, such as glutamine, might support tumor growth. Conversely, amino acid restriction could potentially limit cancer progression, indicating a need for balanced intake.
- Diabetes: Amino acids like arginine play roles in insulin regulation and glucose metabolism. Research has shown that adequate levels of arginine can improve insulin sensitivity.
- Cardiovascular Health: Amino acids such as homocysteine are linked to cardiovascular diseases. Elevated levels can indicate risk factors for heart disease, highlighting the importance of monitoring specific amino acid levels.
Understanding these interactions can help guide nutritional strategies for managing health and preventing diseases. Future research is likely to reveal additional insights into how amino acids can be harnessed in clinical settings.
Culmination
The conclusion of this article serves as a crucial synthesis of the information presented about amino acids and their structural significance. Understanding the fundamental structure of amino acids is essential not only for appreciating their biological roles but also for grasping the wider implications they have in various fields of science, including biochemistry, nutrition, and medicine.
Summary of Key Points
Throughout the article, we examined various aspects of amino acids, including:
- Basic Structure: Each amino acid consists of a central alpha carbon, an amine group, a carboxyl group, and a unique R group that determines its properties and functions.
- Types of Amino Acids: Amino acids are classified into essential, non-essential, and conditional categories, which have different implications for dietary needs and synthesis within the body.
- Peptide Bonds: The formation of peptide bonds is essential in linking amino acids to form proteins, which are critical for cellular functions and overall health.
- Health Implications: We discussed the impact of amino acid deficiencies, their role in diseases, and the importance of maintaining balanced levels for optimal health.
This summation emphasizes the intricate interplay between amino acids and their biological significance, highlighting how disturbances can lead to serious health issues.
Future Directions in Amino Acid Research
Future research on amino acids is pivotal for advancing our understanding of their roles in health and disease. Potential areas of exploration include:
- Metabolic Pathways: Investigating the specific metabolic pathways of amino acids could provide insights into diseases like diabetes, where amino acid metabolism is often disrupted.
- Therapeutic Applications: Studying the therapeutic potential of amino acids in treating mental health disorders by exploring their role as precursors for neurotransmitters.
- Amino Acid Chelation: Research into how certain amino acids can bind and detoxify heavy metals presents potential in addressing environmental health challenges.
- Technological Innovations: The ongoing development of amino acid supplementation methods could enhance understanding of their impacts on performance in fields like sports science and gerontology.
A comprehensive exploration of these areas can lead to breakthroughs in medical science and improved health outcomes. By furthering our investigation into the diverse roles of amino acids, we not only illuminate their critical functions but also pave the way for new therapeutic strategies.