Understanding EDTA's Role in Cell Culture Dynamics
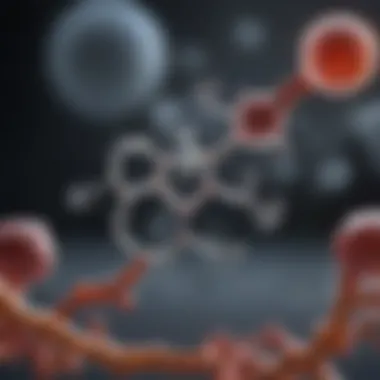
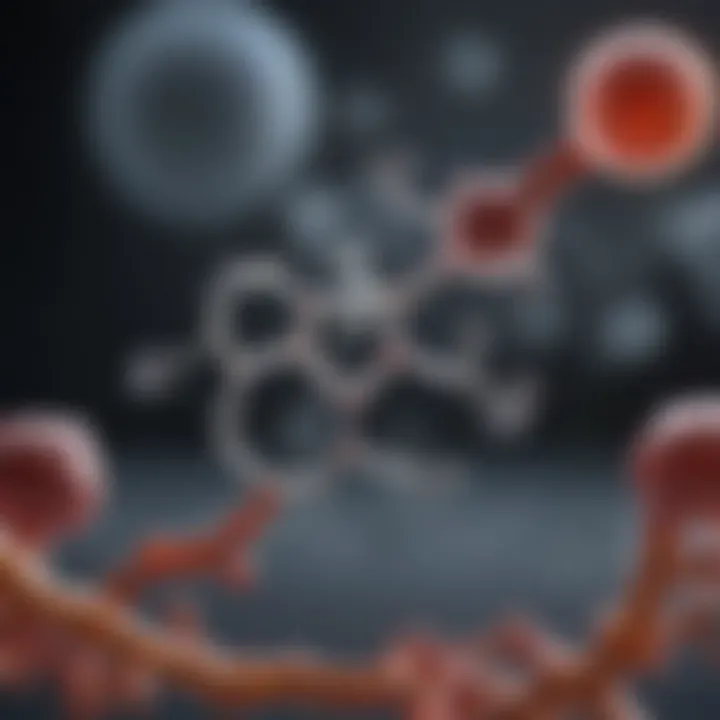
Intro
In the realm of cell biology, the intricacies of cell culture often take center stage. Among the compounds that have garnered significant attention is EDTA, or ethylenediaminetetraacetic acid. This simple chelating agent plays a multifaceted and vital role in maintaining cellular health and enhancing the reliability of experimental outcomes. While one might overlook its significance due to its straightforward chemical structure, a closer look reveals its impact on cellular viability, an essential concern for researchers across various fields.
In cell culture environments, maintaining the delicate balance of ions and molecules is crucial. EDTA aids in this process by chelating metal ions that could otherwise react unfavorably with cellular components. However, it's not just a matter of keeping the peace; understanding how EDTA works paints a fuller picture in the toolbox of researchers. By examining its mechanisms and applications, one gains insight into enhancing cell culture protocols, ultimately leading to better research results.
Foreword to EDTA and Its Role in Cell Culture
In the world of cell culture, EDTA holds a significant position, acting as a vital compound widely utilized in various scientific disciplines. EDTA, or ethylenediaminetetraacetic acid, plays a key role in maintaining a favorable environment in which cells can thrive and reproduce. This section aims to shed light on why understanding EDTA is paramount for anyone venturing into cell culture.
One of the striking aspects of EDTA is its ability to chelate metal ions. These metal ions, when present in excess, can create a hostile environment for cells, leading to stress or even cell death. By binding to these metals, EDTA makes them unavailable, thus facilitating a more stable environment for cellular growth and proliferation.
Furthermore, the compound's influence extends beyond just ion binding. It can also modulate cellular signaling pathways, impacting how cells respond to their surroundings. In essence, EDTA acts not just as a safeguard but also as an active player shaping the cellular environment.
The consideration of EDTA's properties is crucial for both seasoned researchers and newcomers to the field. Its applications in various cell culture methods underscore the need for a deeper understanding of how it functions. The necessity is particularly pronounced when it comes to novel applications in experimental designs, where the subtleties of EDTA interactions with other components can either enhance or hinder research outcomes.
In the sections that follow, we will address the nuances of EDTA's chemical structure, its historical developments in cell culture, and its far-reaching implications in various laboratory settings.
"The importance of EDTA in ensuring cell culture reliability cannot be overstated; it transforms a simple culture into a robust experimental model."
Definition and Chemical Structure of EDTA
EDTA is a synthetic compound characterized by its ability to bind metal ions. Structurally, it is a hexadentate ligand, meaning that it can form multiple bonds with a single metal ion. The exact structure consists of two amino groups and four carboxylic acid groups combined into a single molecular entity. This unique arrangement allows EDTA to trap a variety of metal ions such as calcium, magnesium, and iron effectively.
From a practical standpoint, it's often used in solutions where unwanted metal presence can compromise cell health. By chelating these metals, it leads to increased efficacy in culturing a wide range of cell types, maintaining cellular integrity and function.
Historical Context and Development in Cell Culture
EDTA's journey in the realm of cell culture is quite noteworthy. Initially synthesized in the 1930s, it found early applications in various fields, including medicine and biochemistry. However, its prominent role in cell culture didn't come until much later, notably the 1950s.
As researchers began to realize the importance of keeping metal ions in check to promote optimal cell growth, the addition of EDTA to culture media became commonplace. Back then, the compound was a game-changer. It effectively addressed the need for a consistent approach to reduce unwanted elements that stress cells. With its incorporation into standard culture protocols, many breakthroughs in cellular biology became possible, paving the way for innovations in tissue engineering and regenerative medicine.
In an age of advancing technologies, understanding these historical contexts equipped today’s researchers with crucial insights into how EDTA operates. Knowledge of its past allows for better utilization in current experimental designs, making it an indispensable tool in modern lab settings.
Mechanisms of Action of EDTA
Understanding the mechanisms behind EDTA's functionality is crucial for researchers in cell culture. This knowledge not only bridges gaps in existing literature but also provides insights into how EDTA can be effectively utilized to enhance cell culture practices. By comprehending how EDTA operates at a molecular level, researchers can optimize experimental conditions, leading to more reliable outcomes.
Complexation of Metal Ions
EDTA, or ethylenediaminetetraacetic acid, is primarily known for its ability to complex metal ions. This property stems from its unique structure, which contains multiple carboxyl and amine groups. When EDTA binds to metal ions, it forms stable complexes that can dilute the adverse effects of these ions on cellular environments. Many of these metal ions, such as calcium, magnesium, and iron, can serve as catalysts for destructive processes in culture media.
The complexation process does wonders in various scenarios. For instance, by sequestering calcium ions, EDTA prevents unwanted cellular signaling activation. This can be particularly vital when working with adherent cell cultures, where the right ion balance is crucial for maintaining cell attachment and function. Unintended calcium signaling may disrupt cell behavior, leading to inconsistent results, which researchers absolutely want to avoid.
Not stopping there, EDTA's action also impacts nutrient availability. By removing metal ions that could otherwise precipitate essential nutrients, it ensures a more stable and usable nutrient pool for cells. When applied in different cell lines, researchers must keep an eye on the concentration of EDTA being used since too much of it could lead to suboptimal conditions.
"The role of metal ion complexation by EDTA can't be overstated; it fundamentally shifts how cells interact with their environment, prompting a cascade of biological effects that can make or break an experiment."
Impact on Cellular Signaling Pathways
Cellular signaling pathways are the heartbeat of cellular function, dictating responses to external stimuli. EDTA’s impact here shouldn't be taken lightly. The intricate dance of signals within a cell can be profoundly influenced by the presence (or absence) of certain metal ions. Different cell cultures have varying sensitivities to metal-induced signaling, reflecting the diversity of cellular responses based on their phylogenetic backgrounds.
One of the key effects of EDTA involves inhibiting pathways that would typically be triggered by calcium or magnesium ions. By chelating these ions, EDTA disrupts pathways that lead to cell activation, proliferation, or apoptosis. For example, in scenarios where excessive signaling could lead to premature cell division, the use of EDTA allows researchers to keep things in check. This mechanism becomes especially valuable in focused studies where precise control of cell growth is needed.
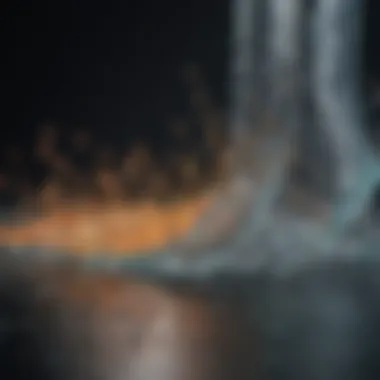
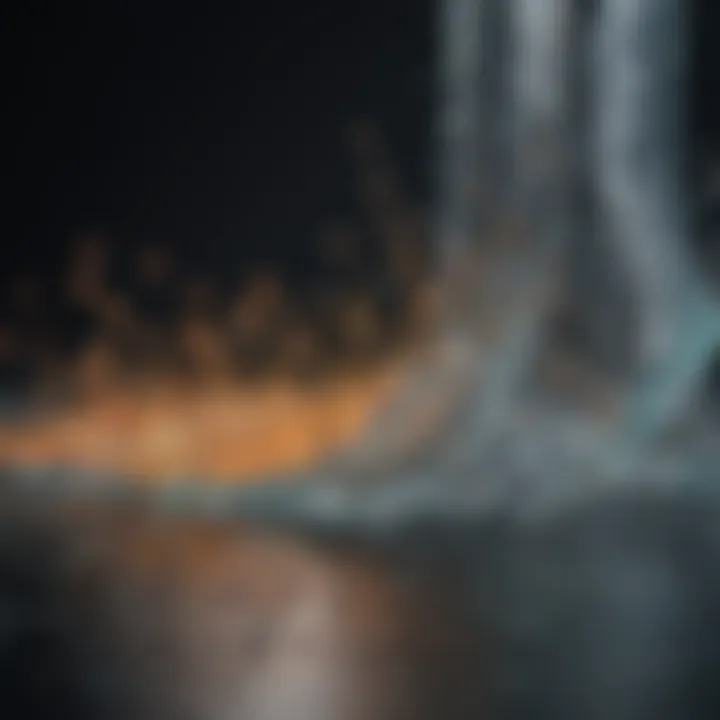
On the flip side, it’s important to acknowledge that disrupting these pathways can also yield unexpected effects. Not all signals are inherently detrimental; some are necessary for cell survival and function. Failure to carefully calibrate the use of EDTA could mean the difference between healthy cell cultures and those that exhibit compromised functionality.
Importance of EDTA in Cell Culture Practices
In the realm of cell culture, EDTA (Ethylenediaminetetraacetic acid) holds a unique and vital role. Understanding its importance encompasses not just a grasp of cellular dynamics, but also a more extensive look into various methodologies employed within the field. With an ever-growing demand for consistency and reproducibility in research results, EDTA emerges as a remarkable ally for scientists.
One of the primary elements to consider is how EDTA enhances both cell viability and proliferation. By controlling the concentrations of metal ions in culture media, EDTA helps create optimal growth conditions. Metal ions, at elevated levels, can induce cellular stress, potentially jeopardizing cell health. Therefore, by chelating these ions, EDTA acts like a safeguard, allowing cells to thrive and proliferate effectively.
Moreover, the reduction of contaminants in cell cultures is another crucial benefit attributed to EDTA. Contaminants, which can arise from various sources, often hinder research quality by introducing unpredictable variables into experiments. EDTA assists in minimizing these risks by complexing with and neutralizing harmful elements, thus creating a cleaner environment conducive for cell growth.
Overall, the adoption of EDTA in cell culture practices isn't merely a matter of routine—it's about embodying best practices that elevate scientific inquiry and improve outcomes. A closer look at the specifics of EDTA's application will reveal how much it truly enhances research reliability and credibility.
Enhancing Cell Viability and Proliferation
EDTA significantly influences cellular life, primarily through its metal chelation abilities. By binding to metals, it reduces unintentional interactions that may lead to cellular toxicity. Cells under duress often show stunted growth or die off, impacting experimental outcomes. In this context, functionality is paramount, and EDTA serves to bolster that.
- Cell Growth Factors: EDTA’s role also extends to maintaining critical growth factors. These factors can be inhibited or rendered ineffective by the presence of certain ions. Thus, by eliminating these detractors, EDTA essentially safeguards those elements essential for robust cell proliferation.
- Dose Considerations: It is important to point out that while EDTA is highly beneficial, its concentration must be fine-tuned. Excessive amounts can lead to adverse effects, emphasizing the need for careful dosages.
Reduction of Contaminants
The presence of contaminants in cell cultures can skew results, leading to erroneous conclusions and wasted resources. Here, EDTA functions almost like a preventive measure, ensuring that the cell culture environment remains free of unwanted agents. Rather than letting problematic substances wreak havoc, EDTA keeps them in check.
- Preventing Heavy Metal Toxicity: Common heavy metals can inadvertentlyfind their way into culture environments. By binding with such metals, EDTA reduces their bioavailability, preventing them from entering cells and causing damage.
- Cleaner Culture Media: With EDTA actively working to mitigate contaminants, cell cultures can yield purer results. This clarity is particularly important when dealing with more sensitive experimental systems, where even the slightest impurity can affect interpretation.
By integrating EDTA into cell culture practices, researchers stand to gain numerous advantages, from enhancing cell health to safeguarding the integrity of their experimental designs. This dual functionality serves as a cornerstone in the adherence to scientific rigor, ensuring that conclusions drawn from cell culture-derived data are sound and significant.
EDTA in Different Types of Cell Cultures
The utilization of EDTA in cell culture is not limited to a one-size-fits-all approach. Instead, understanding its role across different types of cell cultures can significantly optimize experimentation and enhance outcomes. The properties of EDTA, particularly its ability to chelate metal ions and reduce contaminants, can vary in effectiveness based upon the specific demands of adherent or suspension cultures. Each culture type presents its own unique challenges and benefits when EDTA is incorporated into the cell culture environment.
Adherent Cell Cultures
Adherent cell cultures, where cells attach to a substrate, play a pivotal role in biological studies. For these cultures, EDTA has several important functions. Firstly, it helps to prevent the precipitation of calcium ions, which can affect cell attachment and growth. By binding to these metal ions, EDTA ensures that cells maintain a proper environment for adherence. Without it, calcium could cooperate with phosphates, potentially leading to unwanted precipitate which could disrupt cell viability and growth.
Moreover, applying EDTA in adherent cultures can promote the detachment of cells following trypsinization – a common process for subculturing. When trypsin is less effective, the inclusion of EDTA can facilitate a smoother and more efficient detachment process.
Considerations for using EDTA include:
- Optimal Concentration: High concentrations can be toxic; thus, balancing concentrations is crucial for maintaining cell health.
- Timing of Addition: Introducing EDTA at the right stage in the culture process can improve results.
"While EDTA can offer substantial benefits to adherent cultures, it’s essential to pay attention to how it interacts with other components in the medium, to avoid any negative effects on cell behavior."
Suspension Cell Cultures
In contrast, suspension cell cultures, where cells float freely in a liquid medium, encounter a different set of requirements where EDTA's presence can be incredibly beneficial. For suspension cultures, EDTA predominantly plays a role in maintaining a clean culture environment. These types of cultures are particularly sensitive to contamination; thus, reducing the bioavailability of metal ions helps limit microbial growth.
Additionally, the dynamic nature of suspension cultures means that free-floating cells are more susceptible to shear stress. Here, the inclusion of EDTA may ameliorate toxicity by complexing with harmful substances that could potentially impact cell health.
Key aspects for consideration include:
- Impact on Growth Conditions: EDTA could affect nutrient availability, hence its compatibility with the specific cell line needs thorough investigation.
- Influence on Assays: Its presence might interfere with certain assays; thus, careful planning when designing experiments is imperative.
In summary, when incorporating EDTA into both adherent and suspension cultures, understanding the specific needs and characteristics of each type is essential. The benefits may be significant, yet they come with considerations that must be respected to exploit EDTA’s properties fully.
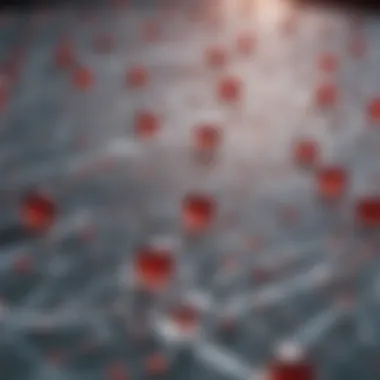
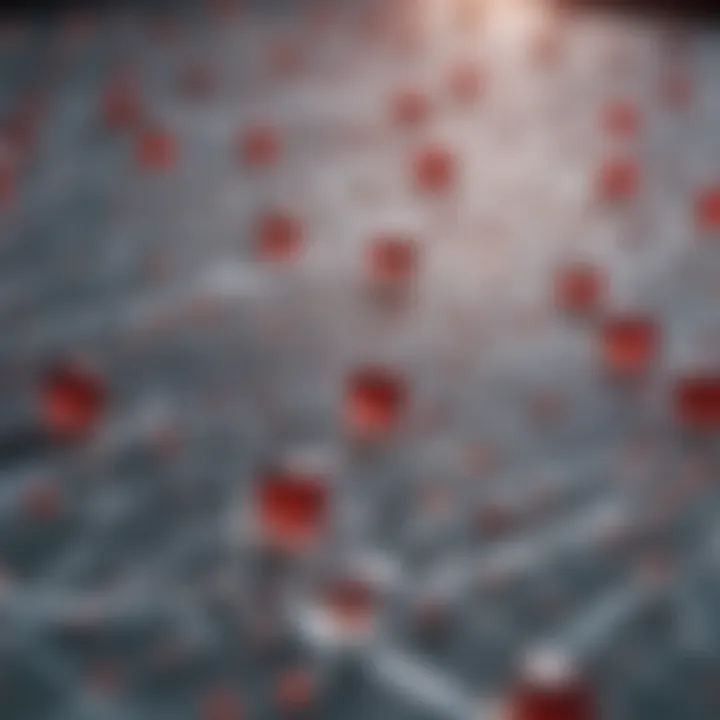
Interactions Between EDTA and Other Culture Media Components
Understanding how EDTA interacts with other components in cell culture media is pivotal for researchers aiming to optimize their experimental outcomes. These interactions can influence the availability of essential nutrients and the overall viability of cultured cells, creating a dynamic balance that researchers must navigate. Consideration of these factors not only enhances cell culture integrity but also informs the design of more reliable experiments.
Influence on Nutrient Availability
EDTA’s ability to chelate metal ions has profound implications for nutrient availability in cell cultures. For example, in cell media where critical metals like zinc and copper are essential for growth, EDTA captures these ions, potentially preventing their interaction with other nutrients or cellular receptors.
- Nutrient Complexation: EDTA can form complexes with essential micronutrients, which may reduce their bioavailability but also stabilize them in solution, preventing precipitation that might occur under certain conditions.
- pH Stability: The presence of EDTA can help maintain the pH levels of culture media by complexing with metal ions that otherwise might cause variations in pH, thus supporting a more controlled environment for cell growth.
However, there’s a caveat: the efficacy of EDTA in this role can also be a double-edged sword. If not monitored, excessive chelation of trace elements may lead to deficiencies that hinder cell proliferation and functionality.
"A precise balance is crucial; while EDTA offers certain advantages, it can also limit the very nutrients that cells depend on for optimal growth."
Synergistic Effects with Other Chelators
Beyond its individual role, EDTA often interacts synergistically with other chelating agents. Understanding these relationships can unlock innovative approaches to enhance cell culture conditions.
- Combining Chelators: When used alongside compounds like citrate or ascorbate, the combined effect may improve metal ion bioavailability more than EDTA alone. For instance, citrate may help maintain soluble fractions of certain metals, while EDTA secures their function by chelating unwanted metal ions that could be toxic or inhibit growth.
- Heterogeneous Interaction: Some research indicates that different chelators can target specific metal ions more effectively than EDTA. An environment with multiple chelators might reduce the prevalence of toxic heavy metals whilst ensuring that essential ions remain available to cells, effectively creating a protective and nurturing culture environment.
In summary, interactions between EDTA and other components of culture media paint a complex picture. Scientists and technicians must remain vigilant, as knowing how best to leverage these interactions can lead to more effective and predictable outcomes in cell culture practices.
Evaluating the Benefits of EDTA in Research
EDTA’s influence in the realm of cell culture is significant and multifaceted, making it vital to thoroughly evaluate its benefits in research settings. Understanding how EDTA interacts with biological systems can provide insights that push experimental design and application to new heights. The primary benefits of using EDTA in cell cultures are tied to its roles in standardizing experimental conditions and enhancing reproducibility in various experimental contexts.
Experimental Condition Standardization
When researchers dive into cell culture, they often find themselves faced with different cell types, growth factors, and media compositions. Standardizing experimental conditions is crucial to reduce variability, allowing for more accurate comparisons between tests. EDTA, due to its chelating properties, can help achieve this standardization by controlling metal ion concentrations, which can alter cell function, growth rates, and other behaviors.
By adding EDTA to culture media, researchers can effectively remove divalent cations like calcium and magnesium, which are known to influence cell adhesion and signaling pathways. Such a controlled environment ensures that results from different experiments remain comparable. This practice is especially fundamental when conducting trials that test new drugs or therapies across various cell lines—favoring an apples-to-apples comparison instead of apples and oranges.
For instance, in the culture of epithelial cells, the presence of calcium can lead to altered cell behavior, including differentiation and proliferation rates. Hence, using EDTA to maintain a consistent calcium concentration across experimental setups allows researchers to focus on the observed effects of their experimental treatments rather than the underlying variability due to differing ion concentrations.
Improving Reproducibility in Experiments
Reproducibility is the holy grail of scientific research; if results can’t be replicated, how reliable are they? EDTA stands out as a tool that can enhance reproducibility in cell culture research. By ensuring that every detail in the culture environment, especially those concerning metal ion concentration, remains constant, variability is reduced.
Notably, the role of EDTA in keeping metal ions at bay helps to eliminate artifacts that may arise due to unregulated changes in cellular environments. For instance, certain cell types may respond differently when exposed to fluctuations in growth media, which might include traces of ions unaccounted for in initial experiments. This incongruity can lead to confusion and misinterpretations of data, hindering scientific advancement.
To underscore this point, numerous studies have shown that the use of EDTA in cell culture not only provides a stable environment conducive to growth but also leads to more reliable data across multiple trials. For example, research involving the application of treatments on cellular models has demonstrated that EDTA-treated conditions yield more consistent results, ultimately making the findings robust and more applicable to real-world scenarios.
Limitations and Considerations of EDTA Use
When delving into the role of EDTA in cell culture, it's crucial to assess its limitations and the considerations that come with its use. While EDTA is invaluable in various applications, it also has set backs that researchers must be aware of. Recognizing these drawbacks enables informed decisions regarding its incorporation in cell cultures and experimentation.
Potential Toxicity to Cells
The application of EDTA is sometimes shadowed by its toxic effects on some cell types. This toxicity is primarily dose-dependent; with higher concentrations yielding increased risks of detrimental outcomes. For example, fibroblast cells have shown reduced growth rates when exposed to elevated EDTA levels. In some scenarios, even prolonged contact with lower concentrations might generate cytotoxic effects. Therefore, it becomes vital to carefully calibrate EDTA concentrations based on the specific cell culture being utilized.
Additionally, certain cell lines, particularly those originating from sensitive tissues, can react unfavorably to EDTA. Toxicity can manifest in various forms: morphological changes, altered growth patterns, or even cell death. This prompts a thorough evaluation of the specific cellular context when integrating EDTA into culture media.
Key points to consider:
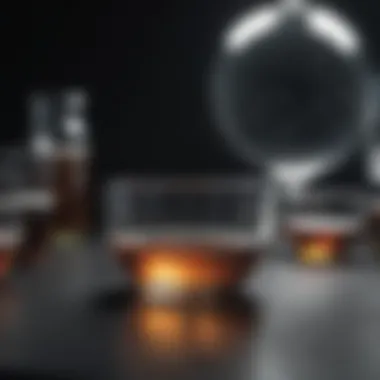
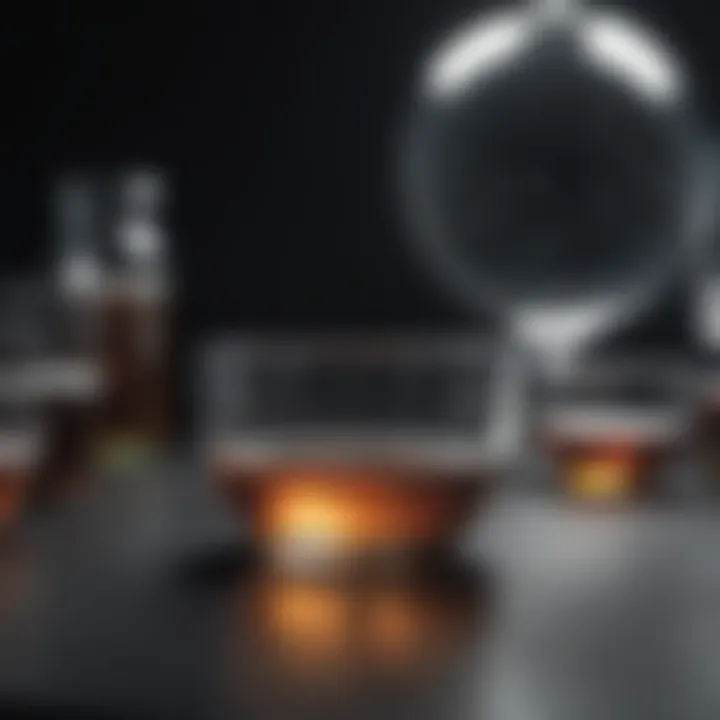
- Cell Line Sensitivity: Different strains might experience varying levels of toxicity, necessitating preliminary tests with each new culture.
- Concentration Control: Monitoring the concentrations in use is elemental; slight adjustments can often mitigate adverse reactions.
- Experimental Designs: Where feasible, preliminary experiments can identify thresholds of EDTA tolerance specific to your cell type.
Interference with Biological Assays
Another aspect to consider is the interaction of EDTA with biological assays which could compromise experimental outcomes. EDTA's chelating properties can disrupt metal-dependent enzymatic activities, thus affecting enzymatic assays that rely on specific metal ions like zinc or magnesium for activity. This occurrence can lead to misleading results and misinterpretation of cellular behavior.
For instance, in assays measuring the functionality of metalloproteins, the presence of EDTA can lead to reduced activity, thereby rendering results questionable. This necessitates caution in experimental designs that evaluate metal ions or require the presence of certain proteins dependent on those ions.
Key points regarding interference:
- Metal Ion Utilization: For assays reliant on metal ions, rule out EDTA to enable accurate results.
- Redundancy in Assays: Consider alternative assay methods that do not involve metal ion dependency if working within an EDTA context.
- Complementary Materials: Some researchers use other agents to counterbalance the effects EDTA may have on substrate availability.
In summary, while EDTA stands as a significant component in cell culture practices, it is not without its challenges. Toxicity potential and interference with assays emphasize the importance of meticulous planning and assessment when adopting EDTA in any experimental framework.
Future Directions for EDTA Research
Exploring the future of EDTA in cell culture reveals a dynamic landscape ripe with potential innovations. As researchers strive to push the boundaries of scientific understanding, the implications of EDTA usage in varied biotechnological fields cannot be overstated. The significance of this topic lies not only in advancing theoretical knowledge but also in practical applications that can improve methodologies employed in current research.
EDTA isn't just a tool; it's a key player in evolving cellular study, and its future directions can unlock many doors, literally and figuratively. The focus on innovative applications and modifications to enhance effectiveness will shape how we use this chelating agent moving forward.
Innovative Applications in Tissue Engineering
The integration of EDTA in tissue engineering is capturing attention like never before. By this, I mean scientists are realizing its potential to create scaffolds that better support cell attachment and growth. Imagine a scaffold embedded with specific metal ions that enable precise cellular responses! By maintaining an optimal ion balance, EDTA can enhance cellular interactions, fostering the engineering of complex tissues.
One prominent consideration is how EDTA might be utilized to modify the properties of these scaffolds. For instance:
- Hydrophilicity Enhancements: EDTA can help improve the water retention capacity of materials, facilitating better nutrient diffusion.
- Ion Release Profiles: The controlled release of metal ions can direct stem cell differentiation, a major advancement in regenerative medicine.
The potential to create vascularized tissues could benefit immensely from EDTA applications. Its chelating properties might also help protect growth factors from degradation, thereby prolonging their activity in engineered tissues. This approach opens new avenues for personalized medicine, where tissues can be customized for specific patient needs.
Potential Modifications of EDTA for Enhanced Effectiveness
When it comes to improving EDTA’s function, researchers are becoming increasingly inventive. The concept of modifying EDTA into derivatives that might be more effective or specific poses exciting possibilities. Consider the implications of tailoring EDTA structures to create targeted chelators that could interact with specific metal ions or cellular pathways.
Some areas for potential modifications include:
- Biologically Compatible Chelators: Adjusting the EDTA structure to enhance its biocompatibility could reduce cytotoxicity, making it more favorable for use in sensitive cell types.
- Targeted Delivery Systems: Developing formulations that allow for localized delivery of EDTA to specific tissues or cells can maximize its effectiveness while minimizing side-effects.
- Polymer Conjugates: Attaching EDTA to polymer backbones could facilitate controlled release and create multifunctional materials for use in manipulative laboratory settings.
In summary, the future of EDTA research looks promising with innovative applications in tissue engineering and modifications honing its capabilities. By delving into these explorations, scientists can ensure they are not merely following trends but rather paving the way for applicable advancements in their fields.
"Innovation distinguishes between a leader and a follower." – Steve Jobs
As the discussion progresses on future directions for EDTA, it's crucial for researchers to remain open to the unexpected contributions this compound may yield. Each step forward not only enhances our understanding of cellular systems but also improves the tools at our disposal, leading to more robust and effective cell culture practices.
Epilogue
The conclusion of this article on EDTA's role in cell culture serves as a crucial synthesis of the various factors discussed throughout this comprehensive exploration. It not only emphasizes the importance of understanding EDTA's biochemical properties and mechanisms but also highlights its significant contributions to enhancing cell viability and ensuring consistency in experimental results. Researchers and practitioners in the field must grasp these nuances to optimize their cell culture practices.
Summary of Key Insights
Throughout our discussion, several key insights about EDTA emerged:
- Metal Ion Complexation: One of EDTA's primary functions in cell culture is its ability to chelate metal ions, which can be detrimental to cell growth if left unchecked. By binding these ions, EDTA helps to prevent toxic effects and promotes a healthier cellular environment.
- Support of Cell Proliferation: The additive properties of EDTA facilitate a boost in cell viability and proliferation, vital for experiments requiring robust cellular responses.
- Reduction of Contaminants: Incorporating EDTA into culture media reduces the risk of microbial contamination, which can compromise experimental integrity.
- Standardization of Conditions: The use of EDTA contributes to the standardization of experimental conditions, an essential factor when aiming for reproducibility across multiple trials.
These elements converge to demonstrate how EDTA functions as more than just a preservative. It is a versatile compound central to the nuances of cellular development and experimentation.
Final Thoughts on EDTA's Role in Cell Culture
EDTA's dynamic interactions within cell culture media signify its worth in advancing biological research. As this essential compound continues to evolve with scientific inquiry, we can anticipate greater efficiencies and innovations in cell culture methodologies.
"Understanding the role of additives like EDTA is essential for ensuring the quality and reliability of experimental results in cell biology."