Cellular Differentiation: Mechanisms and Implications
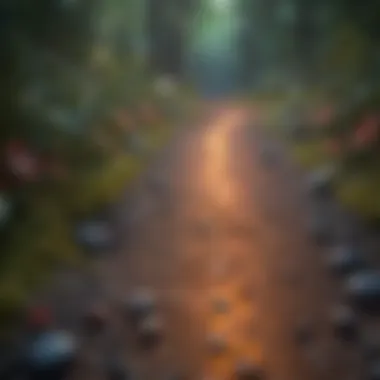
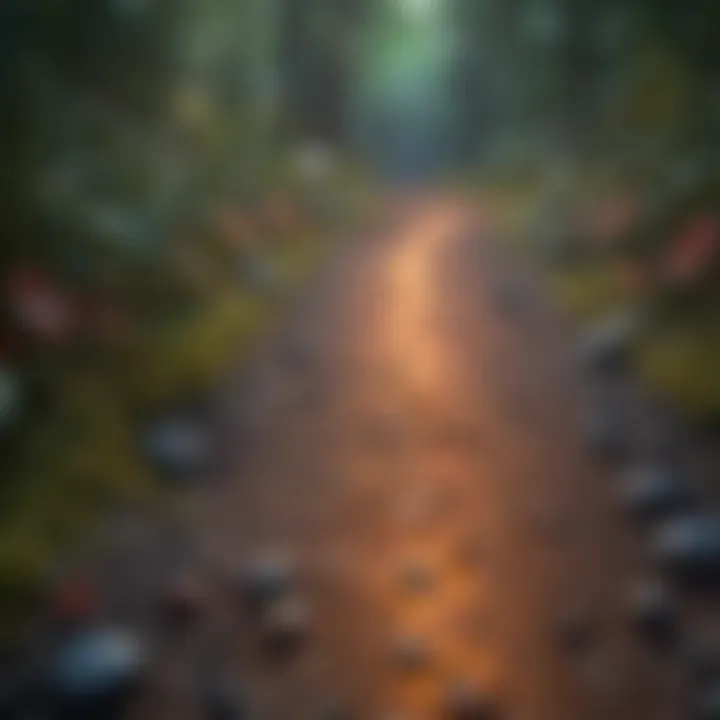
Overview of Research Topic
Cellular differentiation is a vital biological process where unspecialized cells become specialized with distinct functions. This transformation is crucial in the development of multi-cellular organisms and in sustaining various bodily functions. Understanding the mechanisms underlying cellular differentiation not only sheds light on developmental biology but also has profound implications for medical research and therapeutic interventions.
Brief Background and Context
Historically, scientists have been intrigued by how a single fertilized egg can give rise to diverse cell types, from neurons to muscle cells. Early studies focused on embryonic development, but advances in molecular biology now reveal that differentiation occurs throughout an organism's life. Various factors influence this process, including genetic signals and environmental cues.
Importance in Current Scientific Landscape
In today’s scientific environment, knowledge of cellular differentiation is indispensable. Researchers are uncovering how deviations in this process can lead to diseases, such as cancer or developmental disorders. Additionally, understanding differentiation is critical for fields like regenerative medicine, where scientists aim to harness stem cells for therapy. The complexity of cellular differentiation reflects various layers of regulation, making it a rich area for ongoing research and discovery.
Methodology
Research Design and Approach
To investigate the mechanisms of cellular differentiation, a multi-faceted approach is often employed. This includes both experimental and computational techniques. Researchers may conduct in vitro experiments, utilizing cell cultures to observe differentiation under controlled conditions. In vivo studies are also essential, where animal models help illustrate how cellular differentiation occurs in the context of living organisms.
Data Collection Techniques
Data collection in studies of cellular differentiation often involves several techniques:
- Gene expression analysis: This helps identify which genes are active during differentiation.
- Imaging methods: Techniques such as live-cell imaging allow researchers to visualize cellular changes in real-time.
- Biochemical assays: These provide insights into the signals and pathways active during differentiation.
Such a comprehensive methodology ensures a robust understanding of how cells differentiate and the potential implications for health and disease.
Understanding the mechanisms of cellular differentiation is crucial for advancements in regenerative medicine and cancer therapies.
In the next sections, we will delve deeper into the specific mechanisms that drive cellular differentiation and their biological significance.
Preamble to Cellular Differentiation
Cellular differentiation is a vital process that drives the transition of unspecialized cells into distinct cell types with specific functions. This phenomenon is foundational not just in embryonic development but also in tissue maintenance, repair, and various physiological processes throughout life. Understanding cellular differentiation is crucial for multiple fields, including developmental biology, regenerative medicine, and cancer research. The implications of this process stretch across health, disease, and even therapeutic strategies, highlighting the need for a deeper exploration of the mechanisms involved.
Definition and Importance
Cellular differentiation refers to the process by which a less specialized cell becomes a more specialized cell type. This transformation involves a series of tightly regulated genetic, epigenetic, and environmental changes. The importance of this process cannot be overstated; it is essential for proper development, tissue homeostasis, and response to injury. For example, during embryonic development, stem cells differentiate into various cell types like muscle, nerve, and blood cells, allowing for the formation of complex tissues and organs.
Moreover, understanding cellular differentiation enhances our grasp of various diseases. For instance, abnormalities in differentiation processes are implicated in certain developmental disorders and cancers. Research in this area opens doors for developing targeted therapies that can induce or correct differentiation processes, making it a critical area of study in contemporary biology.
Historical Context
The concept of cellular differentiation has evolved significantly over the years. Early studies in the 19th century began to outline the principles of cellular development, primarily through the observations of biologists like Ernst Haeckel. However, it was not until the 20th century that significant breakthroughs occurred. Discoveries related to stem cells paved the way for understanding how these versatile cells could be directed toward specific lineages.
In the latter part of the 20th century, technologies such as in vitro differentiation became pivotal in studying stem cells. Advances in methods like gene editing and molecular biology further propelled this field forward, allowing scientists to dissect the complex mechanisms governing differentiation.
With a rich historical background, the study of cellular differentiation now represents a synthesis of many scientific disciplines, including genetics, molecular biology, and developmental biology.
"Cellular differentiation is not merely cell development; it encompasses the sustained interplay between genetic and environmental factors that defines the diverse cell types in an organism."
This understanding sets the stage for current investigations into the mechanisms of differentiation, its implications in health and disease, and its applications in innovative therapies.
Mechanisms of Cellular Differentiation
The mechanisms of cellular differentiation are vital to understanding how unspecialized cells transform into specialized types with distinct functions. These mechanisms encompass a range of biological processes that are influenced by genetic regulation, signaling pathways, and the cellular environment. Grasping these elements is crucial, as they layer the complexity of developmental biology and have far-reaching implications for health and disease.
Genetic Regulation
Genetic regulation serves as a cornerstone in the process of cellular differentiation. It encompasses the various ways genes are activated or silenced, shaping cell fate and function.
Role of Transcription Factors
Transcription factors are proteins that bind to specific DNA sequences, controlling the transfer of genetic information. They are essential for the regulation of gene expression during differentiation. The key characteristic of transcription factors is their ability to initiate or suppress the transcription of target genes. They offer a powerful mechanism for fine-tuning how cells respond to internal and external signals.
In this article, understanding the role of transcription factors is beneficial because it highlights how specific factors can orchestrate the pathway to specialized cell phenotypes. The unique feature of transcription factors is that they often act in networks, collaborating with other proteins to regulate complex gene interactions.
However, there are challenges associated with transcription factors, such as potential misregulation leading to diseases. For instance, mutations in transcription factors can contribute to developmental disorders or cancers.
Epi-genetic Modifications
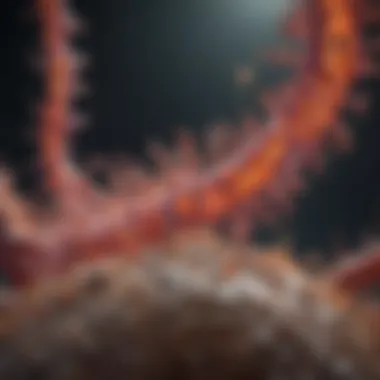
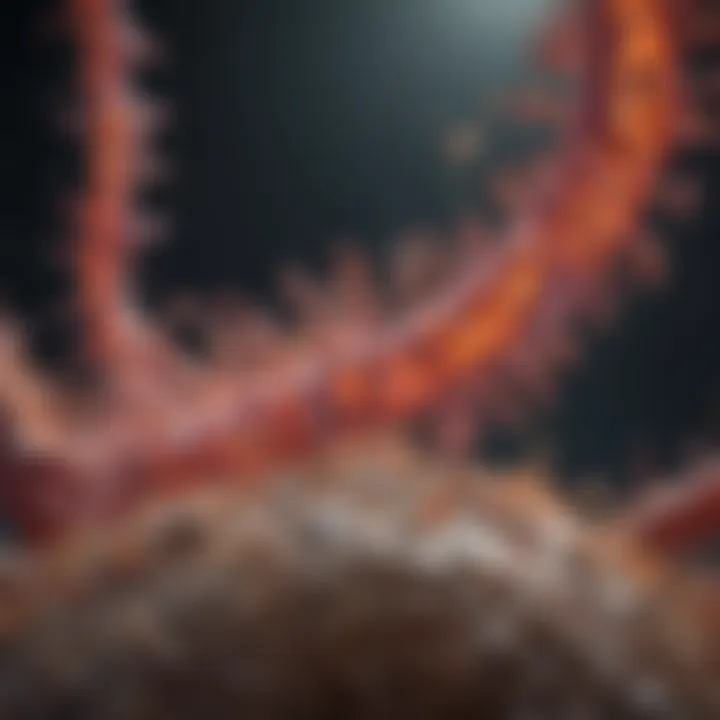
Epi-genetic modifications involve changes to gene activity without altering the underlying DNA sequence. These modifications include DNA methylation and histone modification, which can enhance or repress gene expression. Epigenetics offers flexibility in gene regulation, allowing cells to respond to environmental changes rapidly.
The key characteristic of epigenetic modifications is their reversible nature, providing a dynamic way for cells to adapt during differentiation. This flexibility makes it a beneficial area of focus in this article, especially because it plays a significant role in how stem cells differentiate.
One unique feature of epigenetic modifications is the potential for long-term changes in gene expression patterns. This has advantages in fields such as regenerative medicine, where inducing specific epigenetic changes can guide stem cells towards desired cell types. However, they also have disadvantages; for example, the complex regulatory networks involved can lead to unintended consequences if manipulated in therapeutic settings.
Signaling Pathways
Signaling pathways are crucial for mediating communication within and between cells during differentiation processes. Different pathways can trigger specific responses based on the needs of the developing organism.
Wnt Signaling
Wnt signaling plays an essential role in regulating cell fate decisions. It influences processes like embryonic development and adult tissue homeostasis. The key characteristic of Wnt signaling is its ability to transmit signals from the cell surface to the nucleus, facilitating various developmental paths.
This pathway is particularly relevant for this article as it underscores how external cues can direct internal cellular events. One unique feature of Wnt signaling is its involvement in both normal and tumorigenic processes. Its advantages include governing embryonic development and stem cell maintenance; however, the risk of aberrant activation leading to cancers is a notable disadvantage.
Notch Pathway
The Notch pathway is another vital signaling mechanism in cellular differentiation. This pathway is known for its role in lateral inhibition, where communicating cells influence the fate of adjacent cells. The key characteristic of the Notch pathway is its direct interaction among cells, requiring physical contact to relay signals.
Focusing on the Notch pathway is beneficial, as it emphasizes the importance of cell communication in differentiation. Its unique feature is the context-dependent signaling; different outcomes depend on the relative levels of Notch activation. This can be advantageous in maintaining tissue homeostasis but also presents challenges, as dysregulation can cause developmental abnormalities.
MAPK/ERK Pathway
The MAPK/ERK pathway is well-known for its roles in cell proliferation and differentiation. It transmits signals from cell surface receptors to the nucleus. The key characteristic of this pathway is its ability to integrate multiple signals, allowing for a coordinated response in differentiation processes.
This pathway is significant to this article as it further elucidates how different cellular environments influence development. A unique aspect of the MAPK/ERK pathway is its versatility; it can promote both cell survival and apoptosis depending on the context. Its advantages include promoting rapid responses to external stimuli, but its disadvantages lie in the potential for inappropriate activation leading to diseases like cancer.
Cellular Environment
The cellular environment significantly influences differentiation outcomes. This includes both extracellular components and intercellular interactions that shape developmental pathways.
Extracellular Matrix Influence
The extracellular matrix (ECM) provides structural and biochemical support to surrounding cells. Its composition directly affects cellular behavior during differentiation. The key characteristic of ECM influence is its role in providing physical cues that determine cell shape and function.
This discussion is pertinent to our article as it highlights how the ECM can guide stem cells into specific lineages. The unique aspect of ECM is its dynamic nature; it can change in response to cellular activity. This quality provides benefits in applications like tissue engineering but presents challenges, as variations in ECM can affect differentiation outcomes unpredictably.
Cell-Cell Interactions
Cell-cell interactions are vital for coordinating differentiation among neighboring cells. Communication via signaling molecules allows cells to make context-informed decisions about their fate. The key characteristic of cell-cell interactions is their capacity to maintain tissue organization and function.
Inclusion of cell-cell interactions in this article is crucial as it serves to demonstrate how multi-cellular context is essential for proper differentiation. One unique feature is that these interactions can lead to heterogeneous populations within a tissue, which can be advantageous for functionality. However, if not regulated, they can result in disruptive signals that lead to abnormal development.
Types of Cellular Differentiation
Cellular differentiation refers to the process through which a less specialized cell becomes a more specialized cell type. This transformation is crucial in biological systems, influencing everything from developmental processes to repair mechanisms after injury. Understanding the different types of cellular differentiation can provide insights into various health conditions and diseases. This section focuses on embryonic and adult stem cells, showcasing their unique properties and roles.
Embryonic Stem Cells
Potential and Plasticity
Embryonic stem cells are known for their remarkable potential and plasticity. These cells can differentiate into virtually any cell type in the body, making them incredibly valuable in both research and therapeutic contexts. Their ability to transform into different cell lineages allows for extensive applications in developmental biology and regenerative medicine. A primary characteristic of embryonic stem cells is their pluripotency, which means they can give rise to any cell type derived from the three germ layers: ectoderm, mesoderm, and endoderm.
This quality is beneficial because it opens avenues for repairing damaged tissues and organs. However, there are ethical considerations associated with the use of embryonic stem cells, especially regarding sourcing and the implications of using human embryos in research. Despite these concerns, their unique feature of unlimited differentiation potential makes them pivotal in current scientific inquiry.
Applications in Regenerative Medicine
The applications of embryonic stem cells in regenerative medicine have been a focal point of exploration. Their unique characteristic lies in their ability to differentiate into specialized cells, which can be used to replace damaged or diseased tissues. For instance, researchers are investigating their use in creating insulin-producing cells for diabetes treatment or neurons for neurodegenerative diseases.
The advantages include their adaptability and ability to provide a source of cells that can theoretically address multiple medical challenges. The challenges often revolve around immunological rejection and ethical issues surrounding stem cell procurement. Nevertheless, their role in regenerative medicine is significant and continues to evolve as techniques advance.
Adult Stem Cells
Characteristics and Limitations
Adult stem cells, also known as somatic or tissue-specific stem cells, have a more restricted differentiation potential compared to embryonic stem cells. They primarily give rise to cell types within the specific tissues they reside in, typically exhibiting multipotency. For instance, hematopoietic stem cells can differentiate into various types of blood cells.
A key characteristic of adult stem cells is their ability to maintain tissue homeostasis and repair damaged tissue. However, their limitations are considerable. They are not as versatile as embryonic stem cells and typically have a reduced capacity for self-renewal. This restricted plasticity can hinder their effectiveness in some therapeutic contexts, making research into their capabilities essential for optimizing their use.
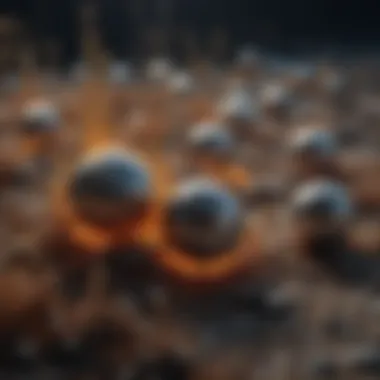
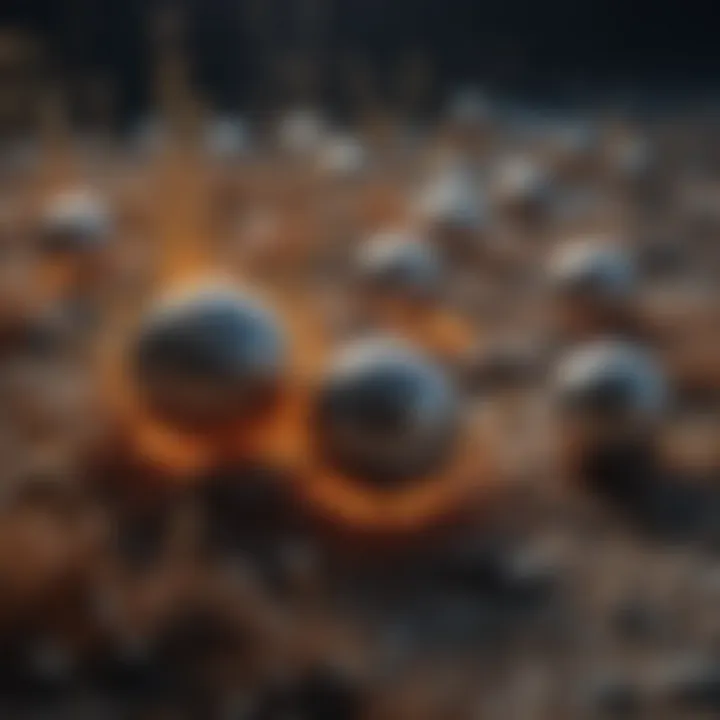
Examples in Tissues
Adult stem cells are found in various tissues including bone marrow, skin, and the intestinal lining. For instance, hematopoietic stem cells from bone marrow are used in treatments for blood cancers and other hematological disorders. Another example is the mesenchymal stem cells found in connective tissues, which are considered for applications in tissue repair.
The primary advantage of adult stem cells is their lower ethical concerns and potential for autologous transplantation, which minimizes risks of rejection. However, their limitation in versatility compared to embryonic stem cells can restrict their applications. Understanding the specific roles of these adult stem cells can guide future research, especially regarding their potential in treating degenerative diseases and injuries.
"Recognizing the different types of cellular differentiation allows us to appreciate the intricate balance of biology, which plays a crucial role in development, healing, and the understanding of diseases."
The exploration of both embryonic and adult stem cells establishes a foundational understanding of cellular differentiation, essential for advancing medical applications and ethical considerations in research.
Impact of Cellular Differentiation on Development
Cellular differentiation plays a crucial role in biological development. It is the process where unspecialized cells become specialized. This affects how organisms grow and function. Differentiation ensures that a variety of cell types are available to form tissues and organs. These specialized cells have specific roles which are vital for survival. The impacts of cellular differentiation are comprehensive, influencing not just development but also the capacity to heal and maintain homeostasis in adult organisms.
Embryonic Development
Stages of Development
The stages of embryonic development represent a sequence of events from fertilization to the formation of a fully functional organism. This process begins with the zygote, which undergoes division and differentiation. Following cleavage, a blastocyst is formed which then implants into the uterine wall. Each stage has its significance. For instance, the formation of the three germ layers — ectoderm, mesoderm, and endoderm — establishes the blueprint for all body structures.
The distinctive characteristic of these stages is the rapid proliferation of cells. This characteristic is essential for creating various tissues and organs. Understanding these stages benefits researchers exploring developmental biology and regenerative medicine.
However, the complexity of these stages can lead to potential issues. Irregularities in any of these stages can result in developmental disorders or abnormalities.
Role in Organogenesis
Organogenesis is the phase where specific organs develop from the embryonic germ layers. This phase is instrumental in shaping the final anatomy of an organism. During organogenesis, signaling pathways guide cells to differentiate and assemble into functional organs. The process includes intricate interactions among cells, crucial for communication and coordination.
A key point of organogenesis is the precise timing of cellular differentiation. Any disruption can result in malformations or functional impairments. This is a popular topic due to ongoing research into how understanding organogenesis can improve strategies for congenital anomalies and transplant medicine. The unique aspect of organogenesis is its reliance on external signals and the local environment. This characteristic underlines the importance of cellular context in determining cell fate. Yet, intervention during this phase must be approached cautiously, as inappropriate manipulation can lead to adverse outcomes.
Tissue Homeostasis
Regenerative Capacities
Regenerative capacities refer to the ability of tissues to repair and regenerate after injury or loss. This relies heavily on the activity of stem and progenitor cells, which can differentiate into required cell types. The regenerative process is pivotal in maintaining the integrity of tissues. In organisms like salamanders, regeneration can restore limb functionality, showcasing impressive regenerative abilities.
A notable aspect of regenerative capacities is their potential in therapy. Understanding these mechanisms can pave the way for innovative treatments in medicine. For example, utilizing stem cells for tissue engineering is an area of active research. Despite its promise, regeneration has limitations. Not all tissues possess equivalent regenerative abilities, and factors such as age and underlying health can influence outcomes.
Response to Injury
The response to injury involves a series of biological processes. When an injury occurs, the body activates specific pathways that facilitate healing. Cellular differentiation plays a key role in this response. Specialized cells are recruited to the injury site. They secrete signaling molecules that guide the healing process. Inflammation and tissue repair must be carefully balanced; acute responses are necessary, however, prolonged inflammation can result in chronic issues.
A key characteristic of this process is the dynamic nature of cellular response. Cells must adapt to varying conditions, thus showcasing plasticity. This high adaptability makes responses to injury a critical area of study. Understanding this feature could enhance methods for treating wounds and injuries. Nevertheless, the complexity of responses to injury presents challenges. Some tissues might not heal effectively due to scarring or improper regeneration.
"Cellular differentiation is fundamental for not just development but also healing processes, highlighting its significance in biology."
In summary, cellular differentiation is central to both the embryonic development stages and the maintenance of tissue homeostasis. Its implications are far-reaching in understanding both normal growth and responses to injury.
Cellular Differentiation and Disease
Cellular differentiation plays a significant role in understanding the relationship between cellular processes and disease. This subject highlights how alterations in differentiation can lead to various pathologies, most notably in cancer and inherited disorders. Insights gained from this field inform both medical research and therapeutic approaches.
Cancer Biology
Differentiation Loss in Tumors
Differentiation loss in tumors describes a key characteristic observed in cancer progression. As tumors develop, they frequently exhibit a shift from specialized cells to more undifferentiated forms. This phenomenon contributes to tumor aggressiveness and the disease's overall complexity. Tumor cells that lose differentiation often gain a higher capacity for growth and invasiveness, making them difficult to treat. The fundamental aspect here is that undifferentiated cells tend to ignore the usual regulatory signals present in normal tissues.
The significance of this loss in differentiation is clear when considering cancer therapies. Tumors that maintain some level of differentiation can often respond better to treatments. Therefore, understanding how differentiation is lost helps in identifying potential therapeutic targets. The unique feature of differentiation loss makes it a focal point for developing new strategies in oncology. The challenge remains, however, in finding effective methods to induce differentiation in malignant cells.
Stem Cell Models in Cancer Research
Stem cell models in cancer research provide innovative insights into cancer biology. These models, derived from pluripotent stem cells, mimic the varying states of cell differentiation observed in tumors. They are a beneficial approach as they allow researchers to study the mechanistic pathways of cancer development. By understanding how stem cells differentiate, scientists can explore how similar processes may fail in cancer.
One key characteristic of stem cell models is their ability to simulate tumor microenvironments. This can lead to a better understanding of the interactions between different cell types in tumors. These models are popular because they not only aid in understanding malignancies but also help test new therapeutic strategies in a controlled setting. However, the unique feature they offer comes with challenges, such as their complexity and the difficulty in replicating all aspects of human tumors.
Inherited Disorders
Genetic Mutations Affecting Differentiation
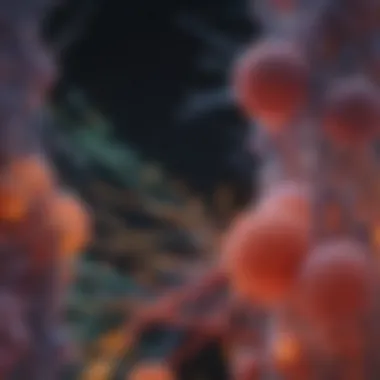
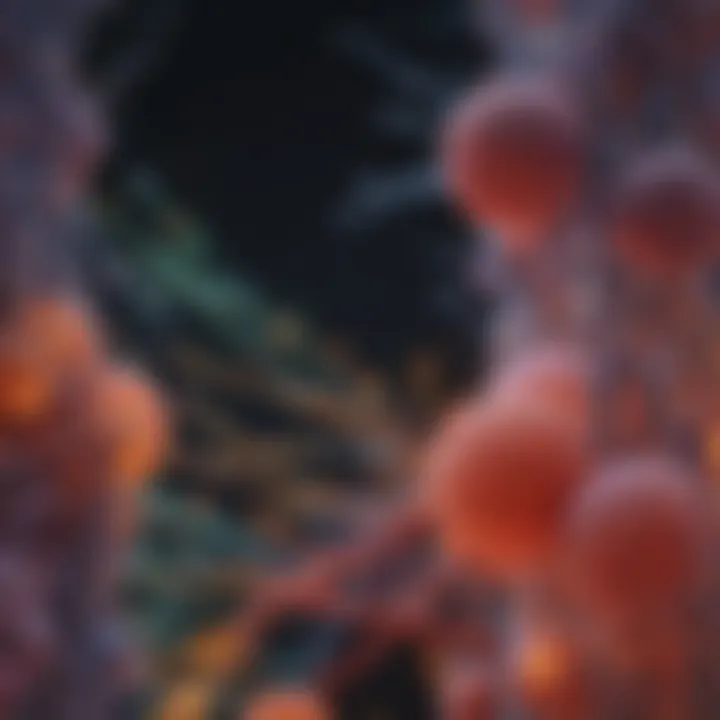
Genetic mutations affecting differentiation play a crucial role in several inherited disorders. These mutations disrupt normal differentiation pathways, leading to improper development of tissues. One powerful aspect of these genetic changes is that they often lead to a wide range of symptoms and severities in affected individuals.
Understanding how these mutations impact cellular differentiation provides valuable insights into possible interventions. This topic is beneficial because it informs genetic counseling and potential gene therapies. The unique feature of these mutations is that they can pinpoint specific cellular defects, guiding research towards tailored treatments. However, challenges remain in effectively correcting these genetic defects in patients.
Therapeutic Approaches
Therapeutic approaches in the context of inherited disorders focus on restoring or compensating for the loss of normal differentiation. These methods can include gene therapy, where normal genes are introduced to correct mutations. Another avenue is the development of drugs that can modulate differentiation pathways. This approach is beneficial as it opens up possibilities for treating multiple disorders with similar underlying mechanisms.
The unique aspect of these therapeutic strategies is their focus on reactivating or bypassing disrupted pathways. This can be a more targeted method than conventional treatments, which may not address the root cause of the disorder. However, some limitations exist, such as the need for precise delivery methods and the potential for off-target effects.
Through understanding cellular differentiation in diseases, significant progress can be made in developing more effective and targeted therapies.
Recent Advances in Research
Recent developments in cellular differentiation have expanded our understanding and opened doors for innovative applications. These advances highlight how technology can transform research in biology and medicine. By focusing on cutting-edge tools like CRISPR and single-cell RNA sequencing, we are seeing new possibilities in understanding cellular behaviors and responses.
Technological Innovations
CRISPR Applications
CRISPR technology has emerged as a powerful tool in genetics. Its specific aspect of gene editing allows for precise modifications to DNA sequences. This feature greatly enhances studies on cellular differentiation, allowing researchers to investigate how specific genes impact the differentiation process.
One key characteristic of CRISPR is its versatility. Researchers can use it to knock out genes or introduce new genetic material. This makes it a beneficial choice for exploring how genetic changes affect cell fate. The ease of designing CRISPR constructs makes it particularly popular in laboratories around the world.
However, the unique feature of CRISPR also comes with consideration of potential off-target effects. Unintended alterations could complicate interpretations of results. Balancing its advantages, like efficiency and accuracy, against these potential drawbacks is crucial in research.
Single-cell RNA Sequencing
Single-cell RNA sequencing allows researchers to examine gene expression at the single-cell level. This method provides deeper insights into cellular differentiation by enabling the study of diverse cell populations within a sample. The ability to analyze individual cells sheds light on how cells respond to various stimuli and differentiate in real-time.
A key characteristic of this technology is its high resolution. Single-cell RNA sequencing reveals heterogeneity in cell responses, which is often missed in bulk sequencing approaches. This reflects its value in advancing our understanding of cell behavior during differentiation.
The unique advantage of single-cell RNA sequencing is its capacity to elucidate the complexity of cell populations. Yet, it can also present challenges, such as data analysis and interpretation, due to the large amounts of data generated. Care in validating results is necessary for meaningful conclusions.
Future Directions
Research in cellular differentiation is now at a pivotal point, with potential for new discoveries and applications on the horizon. By exploring avenues such as disease modeling and regenerative therapeutics, we can anticipate a transformative impact on medicine.
Potential for Disease Modeling
The potential for disease modeling in cellular differentiation research is substantial. This approach enables researchers to create models that mimic disease states by manipulating the differentiation process. Such models allow for the examination of disease mechanisms in a controlled system, which can enhance our understanding of pathologies.
One key advantage of this method is its ability to provide insights into specific cellular behaviors tied to diseases. This makes it a beneficial option for advancing therapeutic strategies. By studying disease mechanisms directly in human cells, researchers can identify targets for intervention and test potential treatments.
Uniquely, the capacity to recreate various disease scenarios from patient-derived cells offers personalized insights, leading to tailored approaches in treatment. However, challenges exist in ensuring that these models accurately represent the complexities of actual diseases. Continuous refinement is necessary for achieving reliable results.
Regenerative Therapeutics
Regenerative therapeutics is an exciting field that focuses on restoring functions of damaged tissues or organs using insights from cellular differentiation. This area leverages knowledge gained from stem cell biology and differentiation pathways to develop treatments for diverse disorders.
The key characteristic of regenerative therapeutics is its potential to heal or replace damaged tissues, which makes it a particularly attractive field in modern medicine. This characteristic highlights its promise in applications ranging from wound healing to organ transplantation.
However, this area also presents unique challenges. The complexities of maintaining stem cell properties and directing their differentiation need careful control. Moreover, ensuring that regenerated cells integrate seamlessly into existing tissues poses additional hurdles. Despite these challenges, the pursuit of regenerative therapeutics holds incredible promise for the future of medicine.
Closure
In this article, we have delved into the intricate world of cellular differentiation, emphasizing its vital role in biology and medicine. The complexity of this process is not only foundational to development but also critical in understanding health and disease. The various mechanisms involved—such as genetic regulation, signaling pathways, and cellular environments—underline its multifaceted nature.
Key benefits of understanding cellular differentiation include:
- Enhanced comprehension of developmental biology.
- Insights into the etiology of diseases, particularly cancers.
- Potential advancements in regenerative medicine through the applications of stem cell research.
Summary of Key Points
Throughout this exploration, several key points have emerged:
- Genetic Regulation: The influence of transcription factors and epigenetic modifications shapes cell fate.
- Signaling Pathways: Pathways such as Wnt, Notch, and MAPK/ERK are crucial in guiding differentiation processes.
- Cellular Environment: The extracellular matrix and cell-cell interactions play essential roles in establishing differentiation pathways.
- Types of Stem Cells: Distinctions between embryonic and adult stem cells shed light on their unique characteristics and applications in medicine.
- Impact on Health: Differentiation is central to understanding various diseases, particularly how differentiation loss relates to cancer pathology.
- Recent Advances: Innovations in tools like CRISPR and single-cell RNA sequencing are paving the way for new discoveries in cellular differentiation.
Implications for Future Research
The vast implications of cellular differentiation extend towards future research avenues:
- Disease Modeling: Advances in cellular differentiation can lead to better models for studying genetic disorders and cancer, enabling precise treatments.
- Regenerative Therapies: The potential of stem cells can revolutionize therapeutic strategies for tissue repair and organ replacement.
- Personalized Medicine: Understanding differentiation may support personalized treatment plans, especially in oncology, tailored to individual genetic profiles.
- Ethical Considerations: As research progresses, ethical considerations regarding stem cell use and genetic manipulation will require careful deliberation.
Overall, cellular differentiation remains a key focus in biological research, necessitating continued investigation to unlock its full potential in medicine and beyond.