Insights into Carbon Capture Power Plants: Tech and Impact
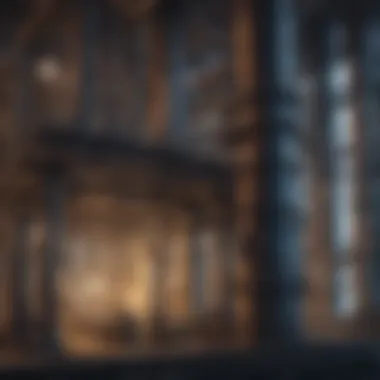
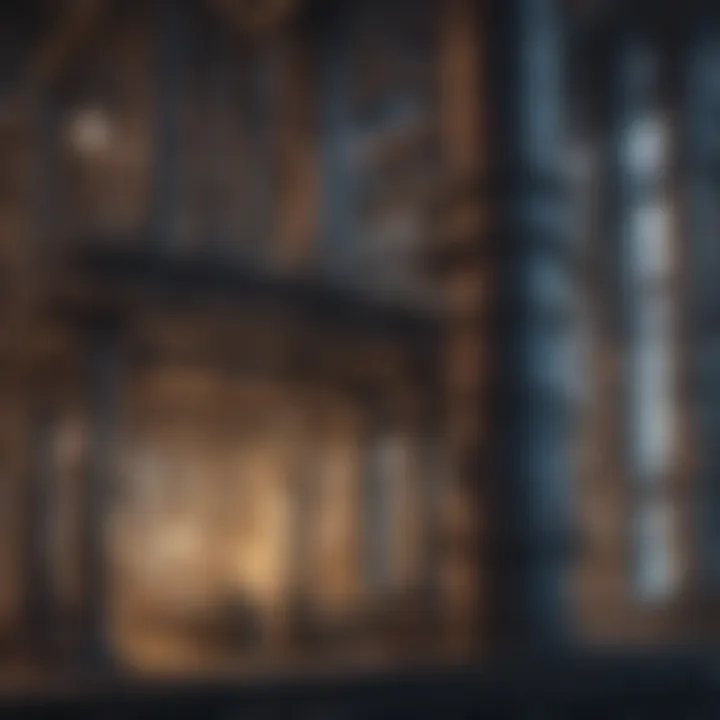
Intro
Carbon dioxide is the silent villain in the ongoing saga of climate change. As society continues to rely on fossil fuels, the need for innovative solutions to mitigate greenhouse gas emissions has never been more pressing. One of the most promising strategies has been the development of carbon capture power plants. These facilities aim to grab excess carbon dioxide from emissions before it can mingle with the atmosphere, creating a potentially game-changing pathway towards a more sustainable energy future.
From a technological standpoint, the realm of carbon capture is multifaceted. It involves a range of methods, from post-combustion capture to direct air capture, each with its own set of advantages and challenges. As global efforts ramp up to tackle climate change, understanding carbon capture's intricacies becomes vital for researchers, policymakers, and industry professionals alike. The stakes are high, and insights into this field could hold the key to balancing energy needs with environmental responsibility.
Understanding these power plants is crucial not only for their technological aspects but also for their economic viability and potential impact on the environment. By exploring how these systems work, the hurdles they face in broader implementation, and their overall potential to change the way we produce and consume energy, this article will provide a nuanced look at a pivotal topic in the transition to a low-carbon economy.
Prelims to Carbon Capture Technology
As the world grapples with the escalating consequences of climate change, the emergence of carbon capture technology has taken center stage. This approach to reducing greenhouse gas emissions is not merely a passing trend but a crucial strategy in our collective fight for a sustainable future. By capturing the carbon dioxide produced from various industrial activities and energy generation, this technology aims to mitigate the adverse impacts of climate change.
The relevance of carbon capture technology is underscored by its potential to significantly enhance the sustainability of traditional power generation methods. It serves as a bridge between current fossil fuel dependence and the eventual transition to renewable energy sources. In this section, we will delve into the definition and historical context of carbon capture, providing insight into how this technology has evolved and why it is essential today.
Definition and Overview
Carbon capture technology broadly refers to a set of processes designed to capture and store carbon dioxide emissions produced from industrial processes or power plants before they enter the atmosphere. This involves several methods and mechanisms, each with its own set of advantages and challenges. The ultimate goal is to prevent CO2, a major contributor to global warming, from contributing to the greenhouse effect.
Carbon capture can take place at various stages of energy production. For instance, it can be integrated into power plants that burn fossil fuels, capturing carbon emissions before they are released and ensuring that the carbon is either stored underground or reused in other industrial processes. This technology encompasses three primary phases: capturing the CO2 from the source, transporting it to a storage site, and finally, securely storing or utilizing it in ways that prevent it from re-entering the atmosphere.
In recent years, advancements in materials and processes have propelled the efficiency and cost-effectiveness of carbon capture technologies, making them more viable for widespread adoption. The implementation of these technologies can lead to significant reductions in emissions and push us toward a cleaner energy paradigm.
Historical Context
The concept of capturing carbon emissions is not entirely new. The roots of carbon capture can be traced back to the early 1970s when scientists began exploring methods for mitigating atmospheric CO2 levels. However, it wasn’t until the late 20th century that significant strides were made in the development of reliable technologies.
One of the pivotal moments came in the 1990s, with the establishment of pilot projects aimed at understanding the effectiveness of carbon capture processes. For instance, Norway's Sleipner project, launched in 1996, focused on capturing CO2 from natural gas production and injecting it into a saline aquifer beneath the North Sea. This marked a watershed moment in demonstrating that carbon capture was not only feasible but could be operationalized on an industrial scale.
Since then, various countries have ramped up efforts to implement these technologies under a variety of regulatory and economic contexts. The urgency surrounding climate change, paired with innovations in capture mechanisms, has spurred both private and public sector investments into research and development.
As we stand at this crossroads in climate action, the historical context of carbon capture technology reveals that, despite its origins, the real challenge lies ahead. We must focus on scaling these technologies effectively and integrating them into our existing energy systems to fulfill the promise they hold for our planet's future.
"Carbon capture technology represents a vital step toward reducing emissions and combating climate change, providing a pathway to sustainability we cannot afford to ignore." - Climate Scientist
The Mechanisms of Carbon Capture
Understanding the mechanisms of carbon capture is crucial to grasp how this technology functions and contributes to a greener future. These mechanisms involve various processes that either separate carbon dioxide from emissions or prevent it from entering the atmosphere in the first place. Each method possesses unique advantages and challenges, which makes them valuable in different contexts. By exploring these processes, we not only appreciate the intricacies of carbon capture but also identify the most efficient strategies for mitigating climate change.
Absorption Processes
Absorption processes present a popular method within carbon capture technology. This technique generally employs a solvent, often amine-based, that chemically reacts with carbon dioxide. As flue gases pass through this solvent, CO2 molecules are absorbed, leaving cleaner gas behind.
The benefit of absorption lies in its effectiveness for capturing a high percentage of carbon dioxide. Studies show that absorption can potentially remove up to 90% of emissions in a well-optimized system. Moreover, its established use in several plants demonstrates its operability on a large scale. However, it's not without challenges. The energy required to regenerate the solvent presents a significant economic consideration, as well as concerns about the long-term stability of the solvent itself.
Adsorption Techniques
In contrast to absorption, adsorption techniques utilize solid materials, called adsorbents, to capture carbon dioxide from gas streams. The basic principle revolves around the adherence of CO2 molecules to the surface of these solids. Typical adsorbents include activated carbon, zeolites, and metal-organic frameworks (MOFs).
One compelling advantage of adsorption is its reduced energy requirement during the regeneration phase. When properly designed, systems using adsorption can operate effectively at lower temperatures. Furthermore, the versatility of adsorbents allows customization based on the specific application and conditions, which can improve efficiency. However, the initial cost of adsorbents and the need for regular maintenance add layers of complexity that must be carefully considered.
Membrane Separation
Membrane separation constitutes a relatively recent innovation in carbon capture technology. It involves the use of selective membranes that allow carbon dioxide to pass through while blocking other gases. The effectiveness of this method largely hinges on the permeability and selectivity of the membrane material.
The primary advantage of membrane separation lies in energy efficiency and compact design. It doesn't require the heavy infrastructure associated with traditional technologies. Moreover, successful implementation can lead to significant reductions in operational costs, thus appealing to power plant operators. However, challenges remain regarding the long-term durability of the membranes and their susceptibility to fouling and other operational issues, which can hinder overall effectiveness.
Chemical Looping
Lastly, chemical looping presents an innovative approach, involving a two-reactor system where metal oxides facilitate the capture of carbon dioxide without the need for gas separation in the conventional sense. In this method, metal oxides are reduced with fuel materials, which generates a flue gas primarily composed of carbon dioxide. The captured CO2 can then be further purified for storage or reuse.
The major strength of chemical looping rests in its inherent process integration, minimizing energy loss during transitions and resulting in higher overall efficiency. Many proponents argue that it can leapfrog traditional approaches, blending excellent CO2 capture rates with power generation. On the flip side, there are still hurdles to jump, such as scaling this technology for widespread use and ensuring the consistent performance of the metal oxides used.
"The successful implementation of these mechanical processes in carbon capture power plants is paramount if we are to transition to a low-carbon economy."
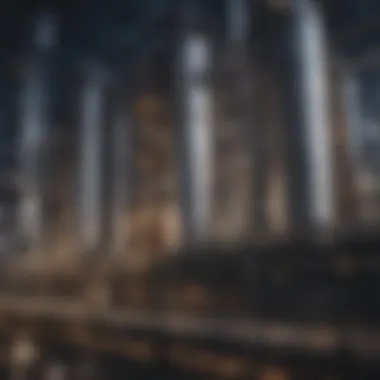
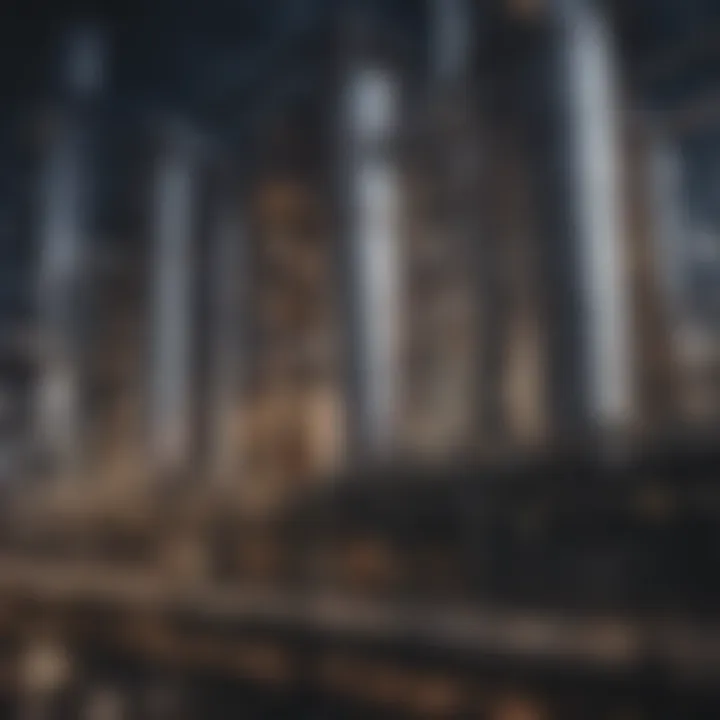
Through these varied mechanisms, the carbon capture landscape reveals a spectrum of techniques that cater to differing needs and conditions. Each option poses distinct advantages and drawbacks that shape their applications in real-world scenarios, making ongoing research and development crucial. It is this nuanced understanding of mechanisms in carbon capture that lays the groundwork for future advancements and innovations.
Types of Carbon Capture Power Plants
Carbon capture power plants are crucial in reducing greenhouse gas emissions and mitigating climate change. The different types of carbon capture technologies available each have unique characteristics, advantages, and potential drawbacks. Understanding these various approaches will help stakeholders in energy production gauge which methods align with their operational frameworks and environmental goals. It's about choosing the right tool for the job; each method can play a significant role depending on the specific context of power generation.
Post-combustion Capture
Post-combustion capture is perhaps the most familiar method among the three types of carbon capture. It refers to the process where carbon dioxide is captured after fossil fuels have been burned. This method employs techniques like solvent absorption, where chemical solutions absorb CO2 from flue gases.
One major benefit of post-combustion capturing includes its flexibility; it can be retrofitted to existing power plants without requiring major changes to the plant's structure. This can facilitate immediate reductions in emissions. On the downside, the energy demand for the capture process can be quite high, sometimes negating the benefits of emissions reductions.
"Post-combustion capture allows us to adapt existing infrastructures to new environmental standards without needing a complete overhaul."
Pre-combustion Capture
In the pre-combustion capture process, carbon is removed before the fuel is combusted. Mainly applicable to gasification processes, such as in integrated gasification combined cycle (IGCC) plants, this method converts fossil fuels into syngas, from which CO2 is removed before energy production.
This approach presents several advantages. For one, it can achieve higher purity levels of hydrogen, which can be utilized for various applications or further used in cleaner energy production. However, the initial investment for setting up such plants is generally higher compared to others. The long-term gain comes in the form of lower operational emissions.
Oxy-fuel Combustion
Oxy-fuel combustion is an approach that involves burning fuel in pure oxygen rather than air. This method increases the concentration of CO2 in the combustion gas, making it easier to capture. A key advantage of oxy-fuel technology lies in its effectiveness in generating a more concentrated stream of CO2 for capture, thus improving the efficiency of the capture process itself.
Nevertheless, it is necessary to point out that employing pure oxygen requires significant changes in the combustion system, which can lead to expensive retrofits in existing infrastructure. Additional research and development are ongoing to make this process more cost-effective and viable for widespread commercialization.
Economic Considerations
The field of carbon capture technology is not just an environmental or scientific phenomenon; it’s also a complex web of economic factors that have significant implications for its growth and viability. Understanding these economic considerations is crucial because they influence the development, deployment, and overall effectiveness of carbon capture power plants. The financial landscape includes costs, funding opportunities, and government incentives that can make or break the success of such technologies. When evaluating the journey towards a sustainable energy system, these elements serve as critical building blocks.
Cost Analysis
Delving into cost analysis is essential, as it provides clarity on both the initial and ongoing expenditures associated with carbon capture power plants. The costs in this realm can be broadly divided into two categories: capital expenditure and operational expenditure. Capital expenditure includes the investment in technology, plant construction, and the integration of carbon capture systems into existing infrastructures. Operational expenditure, on the other hand, concerns maintenance, staff, and energy required for the capture process.
A shifting paradigm is emerging in which the cost of carbon capture technologies is steadily declining, driven by advances in engineering and economics. For instance, the cost per ton of CO2 captured has fallen significantly in the last decade, making it more competitive with traditional energy production methods. Various economic models have suggested that the right adoption strategies could lead to carbon capture becoming a financially feasible option for energy companies. Evaluating these expenses not only informs investment decisions but also shapes the broader economic feasibility of adopting carbon capture solutions.
Funding Opportunities
With rising interest in combating climate change, there are an increasing number of funding opportunities available for carbon capture initiatives. Financial backing can come from both private and public sectors, which can vary from research grants to large-scale financing deals. For instance, many organizations such as the Global CCS Institute and various non-profits actively promote financing opportunities to encourage the development of carbon capture technologies.
Additionally, investors are recognizing the potential returns associated with sustainable solutions, prompting venture capital and private equity firms to allocate resources to promising carbon capture projects. Universities and research institutions are also key players in securing funds, often collaborating with industry partners to bring ideas from the lab to reality. By tapping into diverse funding sources, carbon capture projects can gain momentum and sustainability in a landscape that’s traditionally fraught with financial limitations.
Government Incentives
Government incentives can drastically affect the trajectory of carbon capture projects. Many nations have implemented policies aimed at reducing carbon emissions, sometimes offering subsidies or tax benefits to companies investing in carbon Capture. These incentives can dramatically alter the financial landscape, making it more appealing for businesses to incorporate carbon capture technologies into their operations.
For example, some countries offer a tax credit for each ton of carbon dioxide captured, creating a direct financial incentive for industries that adopt these technologies. Moreover, government initiatives might also include funding for research and development that focuses specifically on improving carbon capture methods. The notion is that by fostering an environment that supports innovation and adoption through incentives, the market for carbon capture power plants can become robust and competitive.
"The economic viability of carbon capture technologies is dependent on a confluence of factors, including cost, funding, and supportive policy frameworks."
In sum, as carbon capture technologies continue to evolve, the intertwined elements of cost analysis, funding opportunities, and government incentives will not only dictate the development pace, but they will also pivot the future of energy towards cleaner, more sustainable practices. The economic perspectives not only shape the practicalities of implementation but also contribute to how societies view and accept these technologies as viable paths to address the carbon crisis.
Operational Challenges
The implementation of carbon capture technology is not without its fair share of complications. This section will delve into the operational challenges that these power plants encounter, emphasizing why the discussion of this topic is crucial for understanding the broader impacts of carbon capture on our environment and economy.
Technical Limitations
While carbon capture processes have shown promise, several technical limitations can hinder their efficiency. For one, capture technology often struggles with energy consumption. The energy required to capture, compress, and transport CO2 can sometimes rival or exceed the energy generated by the plants themselves. When plants utilize technologies such as post-combustion capture, the need for additional energy can lead to reduced overall efficiency in power production.
Moreover, the capture methods employed may be more effective for certain types of emissions sources over others. For instance, post-combustion capture systems are generally better suited for natural gas plants but can face hurdles in capturing emissions from heavy industries like cement or steel production. Furthermore, the efficiency of separation processes—through adsorption or membrane separation—can vary markedly depending on the gas mixtures involved.
Another layer of complexity arises in the maintenance of these systems. High operational temperatures and the corrosive nature of captured gases can adversely affect the durability of equipment used in carbon capture. As the technology stands, it still presents significant obstacles that require ongoing research and development.
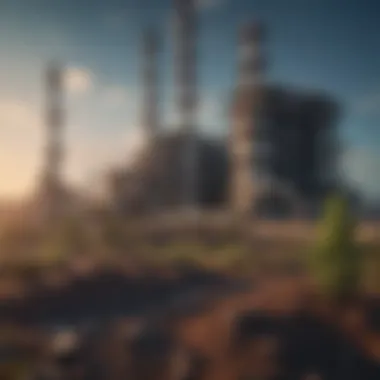
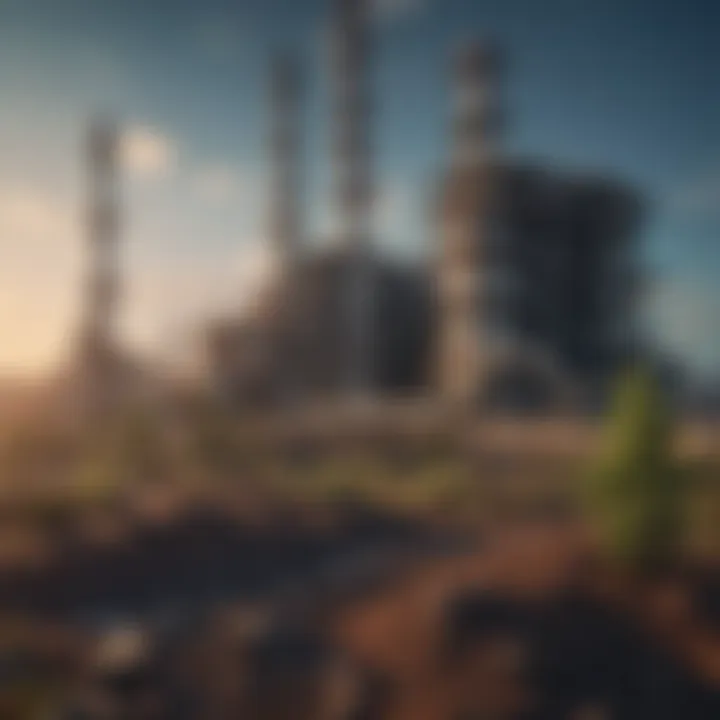
Maintenance and Reliability
The reliability of carbon capture systems is paramount, but maintenance presents its own set of challenges. Regular upkeep is necessary to ensure that the components remain functional and effective. With technologies requiring intricate machinery, such as absorption towers or compression units, failure in any part of the process can lead to significant downtimes.
Moreover, maintenance should not just be reactive but must also account for predictive measures. Staff need to be trained in identifying wear and tear before it impacts operations. Innovations like predictive analytics can be useful here, leveraging data to anticipate mechanical failures based on historical performance.
The operations of a carbon capture plant can also be influenced by external factors, such as fluctuations in the supply of the raw materials used for capture processes—like solvents or membranes—affecting reliability. Moreover, integration with existing infrastructure raises questions about smooth operational transitions and potential disruptions caused by modifications or upgrades to legacy systems.
"In the race against climate change, overcoming operational challenges in carbon capture is not just an obstacle; it's an opportunity for innovation."
The challenges mentioned here underscore the complexity behind carbon capture technology. By addressing these operational hurdles, stakeholders can better navigate the nuances of deploying this technology in the transition to a more sustainable energy landscape.
Environmental Impact
In the conversation around climate change and sustainability, the environmental impact of carbon capture power plants represents a pivotal focus. Understanding how these plants can influence our ecosystem offers insights that are not just theoretical but practical, essential for the ongoing debates about climate strategies. Essentially, carbon capture technology serves as a crucial pathway in mitigating the dire consequences of elevated atmospheric CO2 levels, thus ideally shifting the needle toward a more sustainable future.
The importance of evaluating environmental impact lies in its multifaceted nature, considering both the potential benefits and possible drawbacks. Carbon capture power plants are designed to reduce emissions, and what that means is crucial. On one hand, they could significantly diminish greenhouse gases responsible for global warming. Yet, on the other hand, assessing how they affect local ecosystems, water resources, and human health becomes imperative. Hence, such evaluations must be approached with a holistic lens, where both benefits and downsides are examined closely.
Reduction of Carbon Emissions
Carbon capture power plants have emerged as powerful tools in reducing carbon emissions from industrial processes. By capturing up to 90% of the CO2 produced from power generation, these plants could potentially convert significant amounts of harmful emissions into reusable forms.
- Mechanism of Action: The way these plants work is fascinating. They use various technologies like absorption and adsorption to separate CO2 from other gases. This enables the CO2 to be stored underground or utilized in other processes, like making synthetic fuels.
- Scope of Impact: It’s estimated that widespread implementation could prevent millions of tons of CO2 from entering the atmosphere annually, helping to slow climate change. In contrast, without such measures, emissions from traditional power generation could soar uncontrollably.
- Regulatory Compliance: Many nations are moving towards stricter regulations around emissions. By incorporating carbon capture technology, companies can not only remain compliant but also play their part in global conservation efforts.
These power plants serve as a bridge, allowing us to transition to a cleaner energy landscape while still relying on fossil fuels.
Life Cycle Assessment
Another critical component in understanding the environmental impact of carbon capture power plants is conducting a life cycle assessment (LCA). This evaluation tracks the total environmental footprint of a power plant from its inception to decommissioning, highlighting key areas where impacts can be mitigated.
- Cradle-to-Grave Evaluation: The LCA considers several stages, including raw material extraction, manufacturing, operation, and eventual disposal or recycling. For carbon capture plants, it's not just about capturing CO2; it’s about determining how these processes affect resources like water and land use over time.
- Energy Input vs. Output: One of the main concerns is the energy requirement for operating capture technologies. It’s crucial that the energy used is substantially less than the energy savings gained through emissions reduction. Otherwise, the whole endeavor may become a costly exercise in futility.
- Innovation Opportunities: The findings from LCA studies can often lead to innovation by identifying stages where efficiency can be improved, costs reduced, or emissions further curtailed. For instance, enhancing materials used in construction or optimizing capture processes can contribute to a shrinking overall footprint.
Ultimately, a thorough life cycle assessment fosters greater awareness and responsibility in developing carbon capture power plants.
Future Prospects and Innovations
The evolution of carbon capture power plants is a cornerstone in the battle against climate change. The importance of exploring future prospects and innovations in this space cannot be overstated. As the global focus shifts toward sustainable energy solutions, emerging technologies in carbon capture are paving the way for a greener future. These innovations promise not only to enhance efficiency in reducing carbon emissions but also to integrate seamlessly with existing and emerging energy systems.
Advancements in carbon capture technologies can hold significant potential for capturing greater amounts of CO₂ with reduced costs. By examining the trends and predictions in this field, stakeholders from various sectors—be it science, industry, or policy—can make informed decisions that align with sustainability goals while addressing energy demands.
Emerging Technologies
In the ever-evolving landscape of carbon capture, several emerging technologies show great promise. Here are a few noteworthy ones:
- Direct Air Capture (DAC): This innovative method captures CO₂ directly from the atmosphere. Companies, such as Climeworks, are deploying facilities that utilize fans and chemical processes to extract carbon, making it less reliant on concentrated emission sources.
- Bioenergy with Carbon Capture and Storage (BECCS): This dual approach combines biomass energy generation with carbon capture processes. By using natural decay processes to absorb CO₂ during plant growth, BECCS can create a cycle that effectively reduces atmospheric carbon levels when properly managed.
- Nanotechnology and Advanced Materials: The utilization of advanced materials, such as metal-organic frameworks (MOFs), enhances absorption efficiency. By increasing surface area and optimizing structural properties, these materials can effectively capture CO₂ from gas streams.
The adaptation of these technologies depends largely on economic feasibility and efficient implementation within existing frameworks. The potential of these innovations is significant, offering solutions that could reshape energy industries while mitigating climate impacts.
Integration with Renewable Energy Sources
Another critical aspect of future prospects is how carbon capture technologies integrate with renewable energy sources. This integration is key to a holistic transition toward sustainable energy. Consider the following points:
- Synergistic Benefits: Carbon capture systems powered by renewable energy can operate with lower emissions and higher efficiency. The combination not only curtails CO₂ output further but also promotes the growth of renewable technologies such as solar and wind.
- Energy Storage Solutions: As the intermittency of renewable sources is a challenge, integrating carbon capture with energy storage solutions can smooth energy deployment. Captured CO₂ could be utilized to produce synthetic fuels, enabling a circular economy that bridges conventional systems with renewables.
- Grid Flexibility and Reliability: The integration enhances the overall reliability and flexibility of the energy grid. By employing carbon capture technologies, traditional combustion power plants can adapt to fluctuating renewable output, generating a steadier supply while cutting carbon footprints.
Integrating carbon capture with renewable energy sources can ultimately help in achieving net-zero targets while concurrently boosting energy diversity and security.
To summarize, the path forward for carbon capture power plants is marked by technological innovation and a strategic alignment with renewable energy solutions. The comprehensive exploration of such synergies is crucial, as they represent not just the future of energy but the potential path to a more sustainable and resilient planet.
Case Studies of Carbon Capture Power Plants
Examining detailed case studies of carbon capture power plants provides invaluable insights into real-world implementation of the technology. These examples illuminate not only the successes achieved, but also the challenges faced in operationalizing these systems. Understanding specific scenarios offers key lessons that can guide future projects, ensuring strategies are refined and enhanced based on tangible evidence.
Successful Implementations
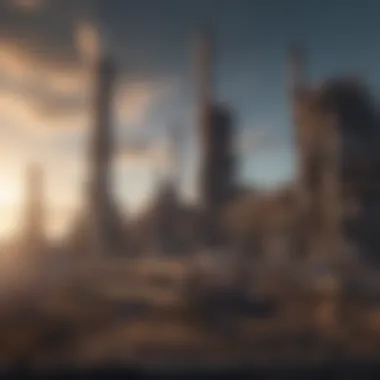
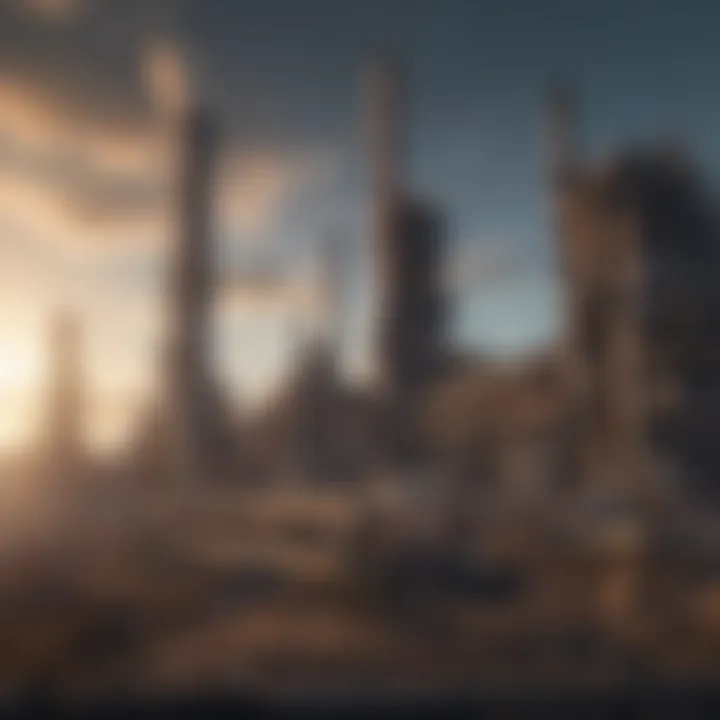
One standout case in the context of successful carbon capture is the Boundary Dam project in Saskatchewan, Canada. This facility is noted for being one of the first large-scale power plants to incorporate carbon capture and storage technology. With its post-combustion capture system, Boundary Dam has reduced carbon emissions by over 1 million metric tons annually.
Moreover, another prominent example is the Petra Nova project in Texas, USA. This project utilizes similar technology to capture over 90% of emissions from a coal-fired power plant. The captured CO2 is repurposed for enhanced oil recovery, showcasing a profitable application of the technology. Case studies like these affirm that the integration of carbon capture in existing infrastructures can lead to significant reductions in greenhouse gas emissions.
Key benefits of these successful implementations include:
- Proven Technology: Demonstrates that carbon capture can be effectively integrated into energy production without significant disruption.
- Economic Viability: Many projects have found ways to make carbon capture financially beneficial through alternative applications of CO2.
- Policy and Incentives: Success stories can further advocate for supportive legislation and incentives focused on reducing emissions.
Despite their success, it’s pivotal to critically analyze outcomes to gather insights into practical challenges.
Lessons from Failures
Contrastingly, not all attempts at implementing carbon capture technology have yielded positive results. The Kemper County Energy Facility in Mississippi stands as a cautionary tale. Originally designed to capture and store CO2, the project faced escalating costs and technical issues, which led to a significant scaling back of carbon capture efforts. Eventually, the facility shifted primarily to natural gas, abandoning its original carbon capture intentions.
This highlights a crucial lesson:
- Feasibility Assessment: A thorough feasibility study, encompassing both technological capabilities and economic factors, is fundamental before embarking on such projects. The Kemper project underlines the importance of realistically assessing resources and technology before investment.
Another example includes the ICAP project in Australia, which aimed to demonstrate innovative carbon capture processes. Despite initial promise, the project encountered operational inefficiencies and was deemed unviable, resulting in its closure.
The lessons derived from these failures underline several considerations:
- Adaptability Is Key: Projects must be adaptable to changing conditions, whether in technology or market dynamics, ensuring a proactive approach to potential obstacles.
- Stakeholder Involvement: Engaging a diverse range of stakeholders from the get-go can help identify vital concerns and expectations, ultimately leading to a smoother project execution.
- Financial Planning: Well-structured financial models must account for unpredictability and the potential for cost overruns.
Regulatory Framework and Policy
The landscape of carbon capture power plants is heavily influenced by regulatory frameworks and policies at both national and international levels. These regulations are critical because they dictate how technology is developed, implemented, and evaluated. Without a robust framework, the innovation in carbon capture can either stall or be implemented without adequate oversight, which could lead to inefficiencies or environmental hazards.
Understanding and integrating such policies is essential not just for compliance but also for fostering a culture of accountability and diligence. Regulation serves as the backbone, guiding the path toward viable carbon capture solutions that could play a significant role in mitigating climate change effects.
International Agreements
International agreements on carbon emissions play a crucial role in shaping the policies behind carbon capture technology. One significant agreement that comes to mind is the Paris Agreement, where nations committed to limiting global warming to below 2 degrees Celsius. Such agreements set the stage for countries to adopt more sustainable practices, including the development and funding of carbon capture power plants.
These global accords underscore several important elements:
- Emission Reduction Targets: Nations are inspired to reduce greenhouse gas emissions, which directly boosts the need for carbon capture technologies.
- Funding and Resources: International cooperation can lead to pooled resources, enhancing research and development of more effective carbon capture mechanisms.
- Standards and Accountability: These agreements force countries to adhere to specific standards, creating a clearer path for implementing carbon capture.
Countries that actively engage with these international commitments tend to see a better alignment of national policies with carbon capture goals. Emerging economies, in particular, may find opportunities for technological transfer and funding through international partnerships. This network of cooperation can lead to innovations that perhaps wouldn’t materialize in isolation.
National Regulations
On the flip side, national regulations provide a framework tailored to each country’s specific needs, considering geographical, economic, and social facets. These regulations often address:
- Permitting Requirements: Getting the necessary permits is crucial for establishing any carbon capture facility. Vague or overly strict regulations could deter investment.
- Incentives for Implementation: National policies can offer tax credits, subsidies, or grants to stimulate interest and funding for technology adoption.
- Monitoring and Reporting: Regular reporting requirements help ensure that the carbon capture technologies perform as claimed, fostering public trust and ensuring that the goals set in international agreements are met.
A prominent example of national regulation is the Clean Air Act in the United States, which has been instrumental in promoting emissions reduction technologies.
The effective move towards a decarbonized future requires a cohesive approach, where international and national policies operate hand in glove. Public awareness and scientific evidence show that ignoring this essential partnership can lead to delays and failures in implementing technologies that have proven their worth in addressing climate challenges. Each stakeholder must work in harmony to secure a sustainable energy future.
“Policies are the lifeblood of technological advancements; without them, we risk stagnation.”
Public Perception and Awareness
Understanding public perception and awareness surrounding carbon capture power plants is crucial for several reasons. Primarily, this technology plays a significant role in addressing climate change. If the public understands how these plants can mitigate emissions and foster a cleaner environment, it fosters support and engagement for such initiatives. Without widespread knowledge, skepticism and resistance may arise, hampering progress in the field.
Public perception is often shaped by various factors, including media representation and personal experiences with energy consumption and pollution. Consequently, educating the populace about the benefits and workings of carbon capture technology can help alleviate fears and misconceptions. A well-informed community is more likely to advocate for and accept the changes that carbon capture power plants bring.
Community Engagement Strategies
Engaging with the community is vital for fostering positive public perception. Strategies for effective engagement can include:
- Open Forums and Dialogues: Hosting public forums allows stakeholders, experts, and citizens to converse directly. Open discussions can clarify misconceptions and highlight the importance of carbon capture in tackling climate issues.
- Partnerships with Local Organizations: Collaborating with local non-profits and environmental groups can foster trust. These organizations often have established credibility within communities and can help disseminate accurate information about carbon capture technology.
- Transparency in Operations: Keeping the community informed about how these plants operate and their safety measures is key. Regular updates and reports on plant performance can also ensure that the public feels included in the process.
- Demonstrations and Tours: Inviting the community to tour existing facilities can offer firsthand insight into the technology. Experiencing the processes firsthand can demystify carbon capture and highlight its benefits.
Educational Initiatives
Education is another cornerstone in shaping public perception. Initiatives aimed at educating diverse populations can have significant impacts. Possible educational measures include:
- School Programs: Introducing curriculum modules on climate change, sustainability, and carbon capture technology in schools can raise awareness from a young age. This ensures that future generations are more informed and engaged.
- Workshops for Adults: Hosting community workshops that provide detailed information about how carbon capture works and its role in a sustainable future can empower adults. These sessions can tackle specific topics, such as the technology's efficiency or its environmental benefits.
- Digital Resources: Leveraging online platforms for educational content can broaden reach. Use of infographics, video documentaries, and podcasts can present information in accessible ways, catering to those who prefer digital learning methods.
- Social Media Campaigns: Utilizing platforms like Facebook and Reddit to disseminate information can create discussions. These campaigns can engage the public in a dialogue, allowing them to voice their concerns and ask questions about carbon capture technology.
"Public engagement is the linchpin for carbon capture technologies. Without understanding, support dwindles, and resistance may grow."