Biomass: Exploring Its Role and Future Potential
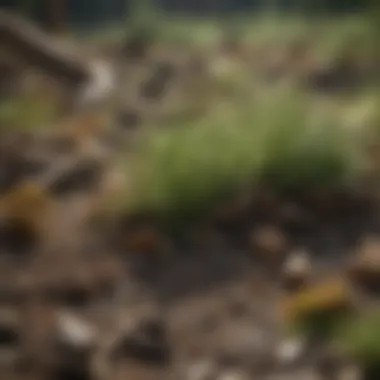
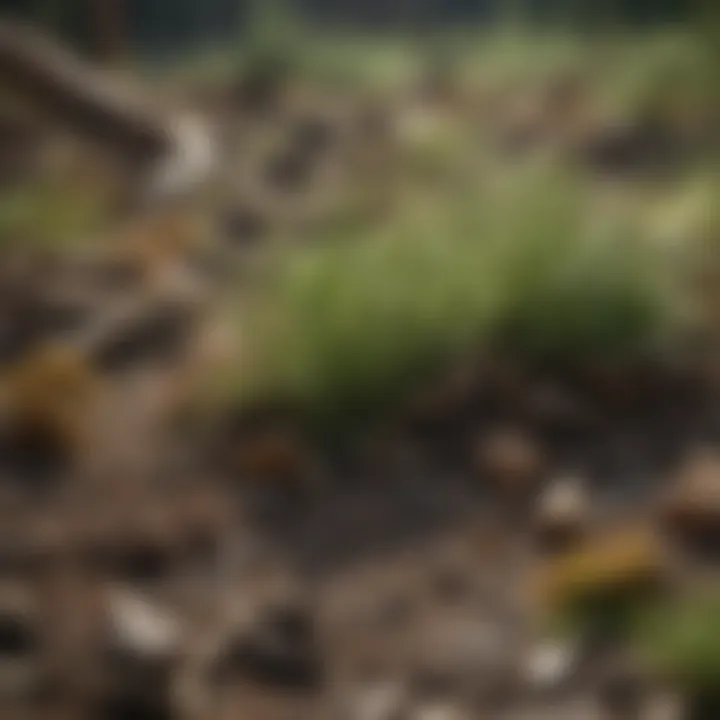
Intro
Biomass energy has carved out a significant niche within the realm of renewable energy, beckoning attention from researchers, policymakers, and industrial players alike. Characterized by a diverse array of organic materials, biomass is often defined as any material derived from living or recently living organisms. This can range from wood and crop waste to even some types of municipal waste. As the world wrestles with the ambitious task of transitioning to more sustainable energy systems, understanding biomass becomes not just useful but essential.
This comprehensive exploration aims to delve into the multifaceted nature of biomass, unearthing its vast potential not only in energy generation but also in contributing to environmental sustainability. The spotlight will shine on various sources, processing methods, and environmental impacts of biomass, while also casting a keen eye on the technological innovations that can increase its scalability.
Many researchers assert that harnessing biomass could mitigate some adverse effects of climate change, presenting opportunities for a greener future. To put the matter in clearer context, many nations are increasingly recognizing the dual benefits—energy security and carbon reduction—offered by this renewable resource.
The narrative will unfold in subsequent sections, aiming to provide readers with a well-rounded understanding, helping them to not only grasp the complexities behind biomass but also appreciate its potential role in the global energy landscape.
Overview of Research Topic
Brief Background and Context
Historically, biomass has been used for millennia, but modern interest surged in light of global climate concerns and energy demands. In simple terms, as fossil fuels face scrutiny due to their emissions and finite nature, biomass stands as a viable alternative. The unique characteristic of biomass lies in its ability to sequester carbon dioxide, potentially balancing out emissions when sourced responsibly.
Importance in Current Scientific Landscape
In the scientific arena, discussions surrounding biomass intersect with domains like environmental science, energy policy, and agricultural practices. Researchers are exploring various strategies to optimize biomass utilization, while also considering its impact on food security and land usage. Skills in these interconnected fields are becoming increasingly vital as professionals seek to navigate the challenges and opportunities presented by biomass.
Those involved in the realms of renewable energy will find that biomass is not just a one-trick pony; it encompasses several technologies and applications, from biofuels to heat generation. Hence, understanding biomass comprehensively could yield economic and ecological benefits that ripple through various sectors.
"Biomass is more than just an energy source; it's an intricate part of the ecosystem that has the potential to forge new paths toward sustainability."
Methodology
Research Design and Approach
The groundwork for understanding biomass is laid through diverse research methodologies. For an exhaustive examination, a combination of qualitative and quantitative analyses is often employed. Case studies illuminate practical applications of biomass, while statistical analysis can provide hard data supporting its viability.
Data Collection Techniques
Data collection for biomass research may employ approaches like:
- Field studies: Gathering real-world data from biomass facilities and farms.
- Surveys and interviews: Engaging with stakeholders including farmers, researchers, and policymakers.
- Literature reviews: Exploring academic journals, governmental reports, and white papers.
This broad spectrum of methodologies enables a thorough and nuanced understanding of biomass, laying the necessary foundation for informed discussions about its future role in energy production and environmental sustainability.
Understanding Biomass
Grasping the concept of biomass is quite pivotal in the realm of renewable energy. By understanding biomass, one can appreciate its potential contributions toward sustainable energy solutions. Biomass refers to any organic material that comes from plants and animals, which can be converted into energy. This definition encompasses a broad array of materials, providing multiple avenues for research and application. With energy security growing into a paramount concern globally, biomass stands as a key player. It offers an alternative to fossil fuels and can help mitigate climate change impacts.
Definition of Biomass
Biomass is essentially biological material derived from living, or recently living organisms. Plants, wood, agricultural crops, and even organic waste from livestock all fall under this umbrella. This material can be utilized for energy production, either directly by burning biomass for heat or converting it into biofuels. The efficacy of biomass as a renewable energy resource hinges largely on its ability to reduce greenhouse gas emissions when compared to conventional fossil fuels. Moreover, because it's derived from sustainable sources, it reinforces the circular economy concept.
Types of Biomass
The classification of biomass into distinct categories allows for a clearer understanding of its sources and applications. Here are the key types:
Organic Waste
Organic waste refers to any biodegradable materials that can be broken down naturally through biological processes. This includes food scraps, yard waste, and other organic materials. One of its notable characteristics is its abundance; it is generated daily in vast quantities, making it a popular choice for biomass energy. Particularly, organic waste contributes significantly to reducing landfill waste, which is a major environmental concern. However, one downside is that effective collection and processing require infrastructure investment.
Agricultural Residues
Agricultural residues comprise byproducts from farming activities, such as stalks, leaves, and husks. These residues are typically left over after harvest and can be harnessed for energy production. A key trait of agricultural residues is their renewability; since they are generated every planting season, they offer a consistent supply for energy conversion processes. They are favored in biomass discussions for their low cost and availability. The challenge lies in seasonal production, which may cause supply fluctuations.
Forestry Products
Forestry products involve raw materials sourced from forests, including wood chips, sawdust, and tree branches. A noteworthy feature here is their direct link to the wood industry, making them easily accessible and economically viable. They are often processed into wood pellets or used in combined heat and power plants. Yet, one significant concern with forestry products revolves around sustainability. If not managed properly, harvesting can lead to deforestation, affecting biodiversity and ecosystem balance.
Energy Crops
Energy crops are specifically cultivated for energy production. Varieties such as switchgrass, miscanthus, and other high-yield plants are chosen not for food, but for their efficiency in biomass conversion methods. The prime advantage of energy crops intersects with their productivity; they can produce a significant output while requiring less water and nutrients than traditional crops. However, there are debates about land-use sustainability and food vs. fuel concerns when dedicating agricultural land to energy crops.
"Biomass not only offers an opportunity to produce energy but also plays a vital role in waste management and sustainability."
Understanding biomass involves acknowledging its various types and the distinct benefits and challenges associated with each. This insight lays the groundwork for exploring how biomass can contribute to a more sustainable energy future.
Sources of Biomass
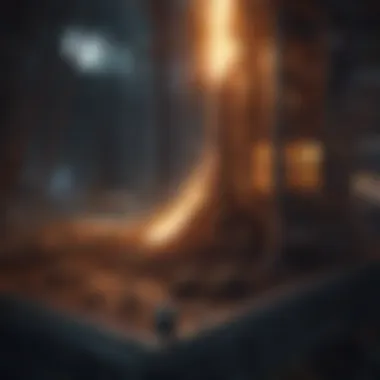
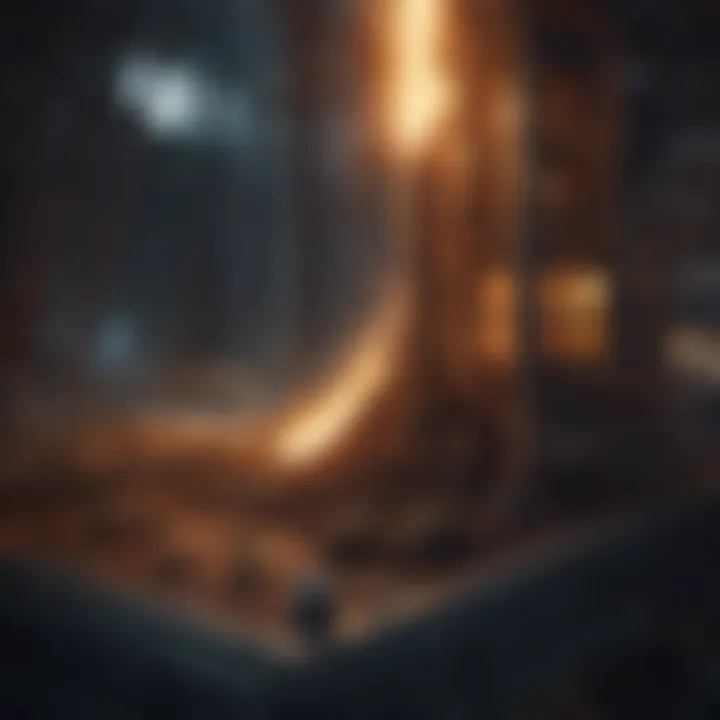
Biomass serves as a renewable resource that is essential in the quest for sustainable energy. Its sources are incredibly diverse, spanning across the agricultural, industrial, municipal, and algal realms. This section emphasizes the significance of understanding these sources as it presents the foundational elements that contribute to biomass production. The various categories not only help in identifying where biomass originates but also underscore the benefits and considerations related to each type, making them pivotal for policymakers, researchers, and industry professionals alike.
Agricultural Biomass
When we talk about agricultural biomass, it encompasses the organic materials produced in the course of agriculture, including residues from crop production and livestock operations. Examples here could be straw from wheat fields, husks left over from corn processing, and even manure generated from livestock farms.
Utilizing agricultural biomass can offer significant benefits for both the environment and the economy. For one, it reduces waste that would otherwise contribute to landfills, promoting a circular economy mindset. This waste conversion can lead to various energy forms, whether through direct combustion or chemical processes such as anaerobic digestion.
However, it's not without challenges. The collection and processing of agricultural residues require specific infrastructure and consistent management. Seasonal variations also pose a hurdle, as the availability of raw materials can fluctuate. This necessitates careful planning in order to maintain a steady supply for energy production.
Industrial Biomass
Industrial biomass primarily refers to organic materials generated from manufacturing processes. This can include wood chips from lumber mills, sawdust from furniture production, or even food processing byproducts like vegetable peels.
The potential of industrial biomass is vast, as it can significantly reduce the environmental footprint of industrial operations. By integrating biomass as a feedstock, industries can enhance their sustainability while also tapping into new avenues for energy production. The transition to more biomass-based processes can lead to lower carbon emissions, contributing positively to climate goals.
Nonetheless, the feasibility of utilizing industrial biomass is dependent on several factors such as cost and logistical efficiency. Companies may face challenges in finding markets for their byproducts or may struggle with supply chain disruptions.
Municipal Biomass
Municipal biomass emerges from household waste and urban activities, including discarded food, yard waste, and paper products. This category stands out due to its role in waste management and energy production as it offers a double benefit: reducing landfill waste while generating energy.
Cities worldwide are searching for effective ways to manage municipal waste, and biomass can play a crucial role here. Instead of letting waste decompose in landfills and release methane—a potent greenhouse gas—converting it through compositing or energy recovery systems can create valuable bioenergy.
However, the collection and processing infrastructure must be robust enough to handle fluctuating waste volumes and different material compositions. This poses a significant challenge, particularly in rapidly growing urban areas.
Algal Biomass
Algal biomass is derived from algae, which flourish in water bodies. They hold immense potential due to their rapid growth rates and their ability to absorb carbon dioxide. Algae can be processed into biofuels, animal feed, and even bioplastics.
The merits of algal biomass are numerous. For example, they can be cultivated on non-arable land, thus not competing with food production. Their ability to sequester CO2 also makes them an ally in combating climate change, contributing to carbon capture and storage efforts.
However, leveraging algal biomass comes with its own set of hurdles. Cultivation techniques still require further innovation to enhance efficiency and cost-effectiveness. The technology to convert algae into usable forms is advancing, but it remains relatively nascent compared to other biomass sources.
"Biomass is a versatile resource that can bridge the gap between waste management and energy production, offering sustainable solutions to our pressing energy needs."
Biomass Conversion Processes
Biomass conversion processes are pivotal in transforming organic materials into renewable energy. This section delves into the different methods of conversion—thermochemical and biochemical—highlighting their importance and benefits. The conversion not only maximizes energy output but also contributes to reducing waste and utilizing biomass resources efficiently.
Thermochemical Conversion
Thermochemical conversion involves the decomposition of biomass through heat and chemical reactions. The beauty of this process lies in its ability to convert solid biomass into cleaner energy forms like gas or liquid fuels. It encompasses several methods, including pyrolysis, gasification, and combustion, each with its distinct characteristics and applications.
Pyrolysis
Pyrolysis is the thermal decomposition of organic material in the absence of oxygen. This process generates solid (char), liquid (bio-oil), and gas products. It's especially noted for its efficiency in breaking down various biomass types, making it a valuable option in today's energy landscape. One key aspect of pyrolysis is its capability to produce bio-oil, which can be further refined into fuels similar to petroleum products.
A unique feature of pyrolysis is the flexibility it offers. It can utilize diverse feedstocks, from agricultural waste to forest residues. However, while it poses a clear benefit of converting biomass into energy-dense products, there are obstacles to consider, such as the management of biochar and potential environmental impacts if not handled responsibly.
Gasification
Gasification is another thermochemical route that converts biomass into synthesis gas, or syngas, which is rich in hydrogen and carbon monoxide. This method operates by partial oxidation at high temperatures, allowing greater efficiency in energy production. Gasification stands out for its potential to produce clean energy from a variety of organic materials, including municipal solid waste.
What makes gasification particularly advantageous is its adaptability to existing power generation systems. The syngas generated can be utilized in turbines and engines, providing an integrated solution for energy supply. However, challenges exist, particularly with the purification of syngas, which is essential for preventing contamination of downstream processes.
Combustion
Combustion, the most straightforward of the thermochemical processes, involves burning biomass to generate heat or electricity. Its simplicity is both its strength and weakness. While employing combustion methods offers a quick way to produce energy, they often lead to higher emissions compared to other conversion technologies.
The key characteristic of combustion is its widespread use in power plants and residential heating systems, making it a familiar option for many. Yet, efficiency can vary greatly depending on the technology used and feedstock quality. Efforts to improve combustion processes aim to minimize emissions and increase energy output, making it more environmentally friendly.
Biochemical Conversion
Biochemical conversion involves the use of biological processes, primarily by microorganisms, to break down biomass into usable energy forms. This method plays a crucial role in the production of biogas and bioethanol, contributing significantly to renewable energy solutions. The two main strategies falling under this category are anaerobic digestion and fermentation.
Anaerobic Digestion
Anaerobic digestion is the microbial breakdown of organic matter in the absence of oxygen, producing biogas that mainly contains methane, which is an excellent fuel source. This method stands out not only for producing energy but also for its effective waste management capabilities, as it can digest agricultural and municipal waste.
A unique feature of anaerobic digestion is its dual role in energy production and waste reduction. It significantly contributes to reducing landfill volume while recovering nutrients that can be used as fertilizers. Nevertheless, careful monitoring is needed to optimize the process and mitigate potential odors and nutrient management issues, ensuring it remains a sustainable alternative.
Fermentation
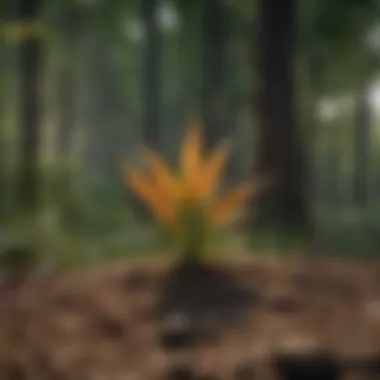
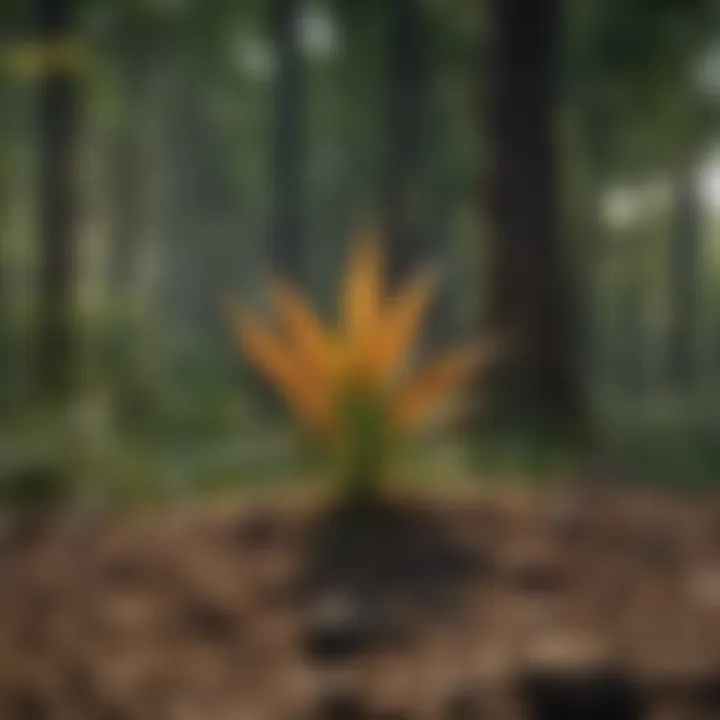
Fermentation is the conversion of sugars into alcohol (ethanol) through the action of microorganisms. This process has been historically important, particularly in the production of bioethanol, which can be blended with gasoline to reduce fossil fuel use.
One key characteristic of fermentation is the wealth of feedstock possibilities—from sugars extracted from crops like sugarcane and corn to cellulosic materials like straw and wood chips. Yet, while fermentation offers a viable pathway to biofuels, the competition for food crops raises sustainability issues. The development of lignocellulosic fermentation technology helps address this by optimizing the use of non-food biomass, reducing ethical concerns tied to food supply.
In sum, the exploration of biomass conversion processes reveals a landscape rich with potential and challenges. Each method presents unique opportunities for energy production while raising important considerations regarding sustainability and efficiency.
Environmental Impact of Biomass
The environmental impact of biomass is a crucial element that cannot be overlooked when assessing its potential as a renewable energy source. It involves examining how biomass production and utilization affect ecosystems, air and water quality, and overall biodiversity. Understanding these impacts allows practitioners, researchers, and policymakers to make informed decisions that balance energy demands with environmental sustainability. When used correctly, biomass can be a game-changer by reducing reliance on fossil fuels while offering multiple ecological benefits. However, mismanagement can lead to significant consequences, which must be navigated carefully.
Carbon Footprint
A significant advantage of biomass is its potential to reduce the overall carbon footprint compared to traditional fossil fuels. When biomass is burned to generate energy, it releases carbon dioxide (CO2). However, this CO2 is part of a natural cycle. The plants that are used as biomass sources absorb CO2 during their growth, effectively offsetting the emissions produced when they are utilized for energy.
Here's a better look at key factors:
- Closed Carbon Cycle: Biomass operates on the principle of a closed carbon cycle. It’s critical to note that if managed responsibly, the carbon released during bioenergy generation is largely balanced by the carbon absorbed during plant growth.
- Long-term Storage: Certain biomass types, when stored or converted into products like biochar, can sequester carbon for longer periods into the soil.
- Comparative Analysis: Studies show that biomass energy can offer a lower carbon intensity compared to coal or natural gas. But this varies based on type, source, and land-use practices.
In this light, while biomass lowers carbon emissions on paper, reality presents a more nuanced picture. Local forestry practices, land competition, and land-use change can alter these benefits significantly, leading to a complex calculation of its true impact.
Impact on Biodiversity
When tapping into biomass for energy, the impact on biodiversity can be a double-edged sword. On one hand, certain biomass practices can enhance ecological diversity, while on the other, mismanagement can lead to habitat destruction and loss of species.
Consider the following factors:
- Sustainable Practices: Utilizing agricultural residues and organic waste can sometimes help in promoting biodiversity, as these materials are often already part of the ecosystem.
- Land Use Change: Converting forests or natural landscapes into biomass plantations can dramatically disrupt local habitats, leading to species displacement.
- Invasive Species: The push for energy crops may lead to the introduction of non-native species into local ecosystems, which can outcompete indigenous plants, decreasing biodiversity.
To sum up, biomass has the potential to support biodiversity but requires careful management and strategic planning to ensure that energy needs do not come at the expense of ecological stability.
Soil Health and Erosion
In the context of biomass, soil health and erosion are paramount considerations. Proper land management practices can either enhance soil quality or contribute to its deterioration.
Here are some noteworthy points:
- Nutrient Cycling: The use of biomass and organic residues can contribute to nutrient cycling within the soil, improving fertility over time. As organic matter breaks down, it enriches the soil with nutrients.
- Erosion Control: Strategic planting of biomass crops can mitigate erosion, as their root systems stabilize soil. This is especially vital in areas prone to heavy rains.
- Mismanagement Risks: However, practices that strip the land of all biomass or fail to rotate crops can deplete soil health, leading to increased erosion and compaction.
Economic Aspects of Biomass
Understanding the economic aspects of biomass is pivotal when evaluating its potential as a renewable energy source. This section sheds light on the intricate relationship between biomass and the economy, encompassing market dynamics and cost-effectiveness. The transition towards renewable energy often hinges on not just the environmental benefits but also on the financial viability of these technologies. Biomass, being versatile, can significantly influence local economies, job creation, and energy independence.
Market Dynamics
The market for biomass is heavily influenced by various factors that can either drive or hinder its growth. Consumer demand for cleaner energy is on the rise, pushing industries and governments to adopt sustainable practices. This shift creates an interesting landscape where:
- Supply Chain Complexity: The haulers, processors, and end-users all add elements of cost and logistics. Efficient management of these factors can enhance market growth.
- Policy Influence: Government incentives and subsidies play a huge role in fostering the biomass market. These can range from credits for biofuel production to support for research initiatives in biomass conversion methods.
- Competition with Fossil Fuels: Traditional energy sources like coal and natural gas often enjoy price advantages due to well-established supply chains, which makes it crucial for biomass to demonstrate its economic viability through competitive pricing.
Keeping an eye on these dynamics can help stakeholders navigate the industry. The growth potential in emerging markets, particularly in regions like Southeast Asia and Africa, indicates a shift towards biomass utilization—provided there’s an equilibrium between cost and efficiency.
Cost-Effectiveness
When it comes to cost-effectiveness, biomass stands out for several reasons, making it a noteworthy contender in today’s energy market. Here’s what to consider:
- Feedstock Variety: Biomass can be derived from numerous sources including agricultural waste, forestry products, and even municipal solid waste. This diversity often enhances its resilience against fluctuating prices in any particular sector.
- Life Cycle Costs: It’s vital to evaluate not just the initial investments, but also the long-term operational costs. For instance, biomass power plants might require significant capital but can yield lower annual operating expenses compared to fossil fuel plants over time.
- Job Creation: The biomass sector can create jobs not only in production but also in collection, transport, and processing. It often requires local labor and thus can significantly boost community economies.
- Energy Independence: By investing in local biomass resources, countries can decrease their dependence on imported fossil fuels, providing a significant economic buffer against global market volatility.
The effectiveness of biomass as a renewable energy source is ultimately tethered to its economic feasibility and the ability to capture emerging market opportunities.
In summary, addressing the economic aspects of biomass is crucial to recognizing its multiplicative benefits. From influencing job markets and energy independence to competing efficiently in diverse markets, biomass has the potential, when properly managed, to be a cornerstone of sustainable energy policies.
Policy and Regulatory Framework
When discussing biomass, the policy and regulatory framework can’t be overlooked. It plays a crucial role in shaping the landscape for biomass energy production and utilization. This framework encompasses the myriad of laws, regulations, and initiatives set forth by governments and global bodies that regulate biomass use. These policies are vital for encouraging investment, promoting sustainable practices, and ensuring that biomass contributes positively to energy needs and environmental goals.
The significance of policies surrounding biomass becomes apparent when you acknowledge their influence in ensuring that biomass resources are developed sustainably and cost-effectively. Effective policies can incentivize innovation, guide research and development, and create viable markets for biomass energy. Without a supportive regulatory environment, advancements in biomass technology and infrastructure may stagnate.
Global Initiatives
Numerous global initiatives have emerged to bolster the biomass sector. These initiatives promote best practices and support sustainable biomass technologies worldwide. The United Nations Framework Convention on Climate Change (UNFCCC) has acknowledged biomass as a potential tool for reducing greenhouse gas emissions while encouraging sustainable land use practices.
Several collaborations, such as the Global Bioenergy Partnership, have been established to enhance the exchange of knowledge and experience among countries. This platform allows nations to share their successes and challenges in expanding their biomass sectors. Additionally, various international funding mechanisms are available to nations looking to invest in biomass technologies. These funds are often accompanied by guidelines to ensure that projects adhere to environmental and social standards.
For instance, the BioFuture Platform aims to consolidate global efforts to promote sustainable bioenergy, encouraging nations to commit to specific targets concerning biofuel production and utilization.
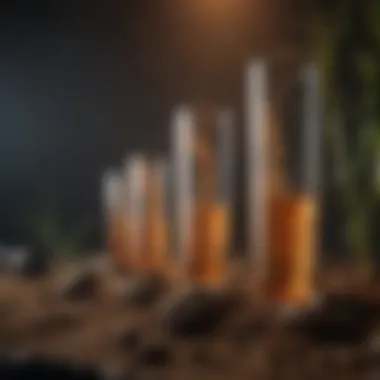
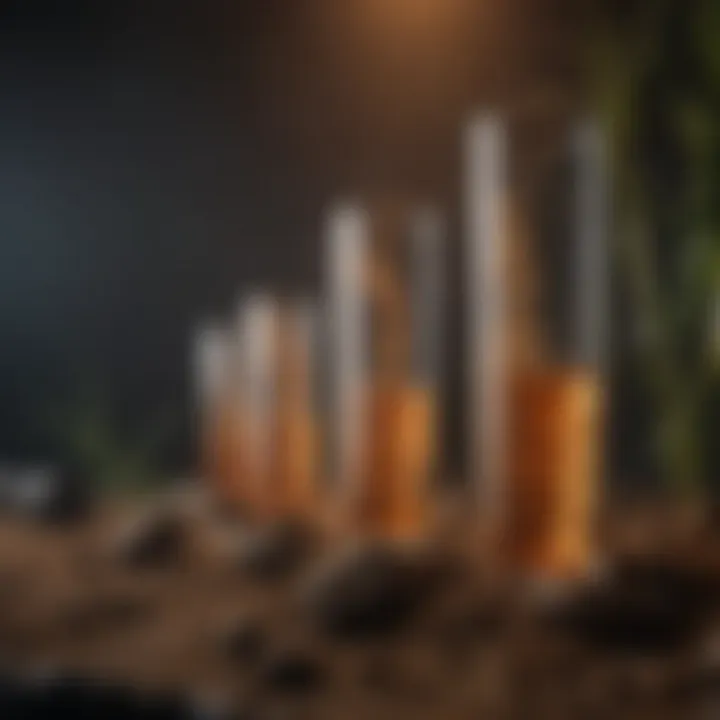
"A coordinated global effort is necessary for the sustainable expansion of biomass, considering its potential risks and benefits."
National Policies
On the national level, policies can significantly differ. Governments often tailor their biomass policies to fit their specific resources, economic structures, and environmental goals. Many countries have devised legislation that establishes mandates for renewable energy sources, including biomass. Such mandates encourage utilities to include a certain percentage of bioenergy in their energy mix.
For example, in the United States, the Renewable Fuel Standard requires a specific volume of renewable fuel to replace or reduce the quantity of petroleum-based transportation fuel, heating oil, or jet fuel. This policy has stimulated the production of biofuels derived from various biomass sources.
Similarly, countries within the European Union have established the EU Renewable Energy Directive, which sets legally binding targets for renewables, including biomass. This directive not only supports market mechanisms but also promotes transparency and sustainability in biomass supply chains.
Technological Advances in Biomass
The landscape of biomass utilization is changing, thanks largely to advances in technology. In this section, we will explore how these innovations are reshaping the biomass industry, optimizing its potential for energy production, and addressing environmental challenges.
Innovative Conversion Technologies
Conversion of biomass into usable energy involves various methods, with several innovative technologies emerging recently. These technologies aim to maximize energy output while minimizing waste. For instance, gasification allows biomass to be converted into synthetic gas, which can be further transformed into electricity or fuels. This process has gained traction because it can handle a variety of feedstocks, something that traditional combustion methods struggle with.
Another noteworthy technology is pyrolysis. This method heats biomass in the absence of oxygen, breaking it down into bio-oil, biochar, and syngas. Each of these byproducts can be used for various applications, such as fertilizers or energy carriers. While the syngas can be burned for heat or converted into chemicals, the resulting biochar can improve soil health, making it a dual-purpose solution.
As efficiency improves and costs go down, biomass conversion processes are becoming more viable options in both developed and developing nations. These advancements make biomass not just a supplementary energy source but a fundamental pillar in achieving renewable energy targets.
Biomass to Biofuel Technologies
The journey from biomass to biofuel is a critical one. Technologies in this area have been evolving to enhance conversion efficiency and reduce production costs. Fermentation is a prime example, where microorganisms break down carbohydrates in biomass to produce ethanol, a widely used biofuel. This method has expanded beyond corn and sugar cane to include agricultural residues such as straw and husks.
Moreover, researchers are also turning their attention to transesterification, a process that converts oils and fats into biodiesel. The growth of oilseed crops like canola and soybeans has opened up new avenues for biodiesel production. The formidable flexibility in feedstock choice plays an essential role in developing robust biofuel markets.
To sum up, the advances in biomass conversion and biofuel technologies are pivotal for sustainable development. They not only provide renewable energy solutions but also contribute to environmental conservation and soil health. The ongoing innovations promise to make biomass a central player in addressing global energy demands while considering the environmental footprint.
"Technological advances in biomass not only open doors to renewable energy but also offer pathways to sustainability that can benefit future generations."
Challenges in Biomass Utilization
Biomass energy, while a key player in the renewable energy sector, faces several hurdles that must be addressed to realize its full potential. Understanding these challenges is crucial as they directly influence the efficiency, affordability, and acceptance of biomass as a viable energy alternative. An open discourse on these complications not only highlights the areas needing attention but also presents opportunities for innovating and improving the way biomass is utilized.
Sustainability Concerns
Biomass is often hailed as a sustainable energy source since it predominantly relies on renewable materials. However, sustainability in biomass utilization introduces a paradox. The sourcing of biomass can sometimes lead to contentious practices like deforestation and land degradation. For instance, consider the cultivation of dedicated energy crops; if practiced on a large scale without careful management, this can transform ecological habitats and disrupt biodiversity.
In a broader sense, sustainability concerns also extend to the energy inputs required for biomass production and processing. Fertilizers, water usage, and fossil fuel consumption during cultivation and transportation can offset the ecological benefits. Thus, adopting a regenerative approach is pivotal. Integrating techniques such as agroforestry or perennial cropping systems might alleviate some of these pressures by promoting healthier ecosystems and enhancing carbon sequestration.
Scalability Issues
Scaling up biomass utilization is another significant challenge. The transition from small, localized facilities to larger, more integrated systems often encounters logistical hurdles. For instance, the need for consistent and reliable biomass feedstock demands an advanced supply chain that can manage fluctuations in availability and quality.
To illustrate, a biomass facility depends heavily on nearby agricultural activities. If a farmer decides to switch crops or experiences a poor yield due to weather conditions, the power generation facility may be left scrambling for new sources. Furthermore, investment in infrastructure—like transport systems and processing plants—can be daunting, both financially and politically. Technological innovation, along with government support, can help bridge this gap, ensuring that the expansion of biomass utilization is feasible and effective.
Public Perception and Acceptance
Public perception of biomass energy is a double-edged sword. On one hand, many view it favorably as a greener alternative to fossil fuels; on the other, there remain significant apprehensions about its environmental safety and health impacts. Misunderstandings surrounding biomass burning often lead to resistance. For example, critics point out that smoke emissions from biomass can rival that of coal combustion, raising concerns about air quality and public health.
Social acceptance of biomass projects also varies greatly among communities. Factors such as local environmental policies, historical context, and prior experiences with energy projects can shape opinions. Engaging communities through transparent communication and educational initiatives is essential for fostering trust and acceptance. Moreover, collaborating with local stakeholders to tailor biomass solutions according to specific community needs and expectations can enhance public engagement.
"Addressing the challenges in biomass utilization not only enhances its role in the energy landscape but sets the stage for a more sustainable and accepted energy future."
Conclusively, the challenges encompassing biomass utilization—ranging from sustainability concerns, scalability issues to public perception—demand a multifaceted approach to ensure effective and responsible energy generation. By navigating these complexities, biomass can become an integral part of the renewable energy mix, contributing significantly to a cleaner and more sustainable future.
Future Outlook for Biomass
The significance of the future outlook for biomass cannot be overstated, especially as the world grapples with the urgent need for sustainable energy alternatives. The role of biomass in our energy portfolio has evolved tremendously, and it is increasingly recognized as a pivotal player in achieving energy security and reducing greenhouse gas emissions. Looking forward, the prospects for biomass are tied closely to advances in technology, shifts in policy, and the evolving landscape of climate science.
Biomass has the potential to save not only our environment but also bolster local economies, presenting opportunities in rural areas where agricultural and forestry residues are plentiful. This section delves into various elements surrounding the future of biomass, touching on emerging trends, innovative developments, and the challenges it faces in a rapidly changing world.
Trends in Research and Development
Research and development (R&D) in biomass technology has been on a significant uptick, spurred by the need for cleaner and more efficient energy sources. Several key trends are reshaping the narrative around biomass:
- Advanced Conversion Techniques: Innovations in thermochemical and biochemical conversion processes are making it feasible to produce a wider variety of fuels and chemicals from biomass. For instance, the adoption of hydrothermal liquefaction offers a new frontier in producing biofuels from wet biomass sources, which were often deemed unsuitable for traditional methods.
- Genetic Engineering Advances: Techniques like CRISPR-Cas9 are being utilized to tailor energy crops for higher yields and better conversion rates. Factors like stress tolerance can be engineered so these crops thrive even in less than optimal conditions, which helps ensure consistent production.
- Waste Valorization: Research is increasingly focused on turning waste streams from agriculture and forestry into valuable energy sources. This not only solves disposal issues but also adds value to what was previously considered useless.
Essentially, the research landscape is making strides toward making biomass an integral part of a circular economy.
Biomass and Climate Change Mitigation
The role of biomass in mitigating climate change is intricately linked to its potential as a renewable energy source. While the combustion of fossil fuels releases a slew of greenhouse gases into the atmosphere, biomass can serve as a more balanced alternative. Here are some of the critical elements influencing how biomass addresses climate-related concerns:
- Carbon Neutrality Debate: As plants grow, they absorb carbon dioxide—which can offset the emissions released upon burning biomass, creating a closed carbon cycle. However, the debate around its carbon neutrality hinges on sustainable sourcing practices and land-use changes. If biomass is not produced sustainably, the benefits can be undermined.
- Integration with Other Renewables: Biomass can complement other renewable technologies such as solar and wind. For instance, biomass can be converted into biofuels, serving not only as energy for vehicles but also as a way to store energy for later use, enhancing the reliability of renewable energy systems.
- Policy Support and Incentives: The greatest strides in biomass for climate change mitigation will rely heavily on policy frameworks that incentivize its adoption. National governments and international bodies are more frequently acknowledging biomass in climate action plans, which could reinforce its role as a viable energy source long-term.
In summary, the future of biomass is characterized by innovative practices that can transform it from mere waste into powerful energy solutions, playing a crucial role in our fight against climate change.