Exploring the Applications of Quantum Computing
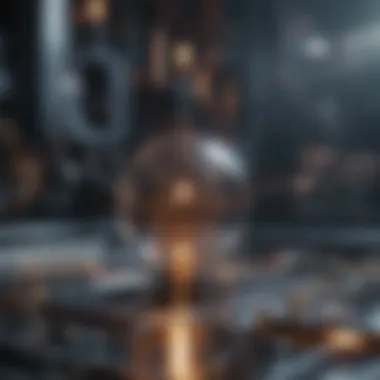
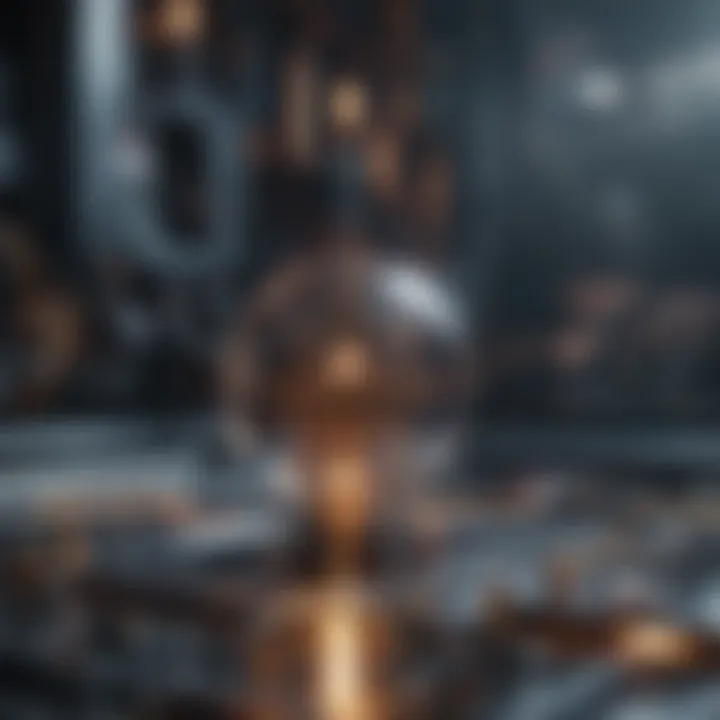
Overview of Research Topic
Quantum computing is not just a technological evolution; it represents a significant shift in the way we process information. The essence of quantum computing lies in its ability to leverage the principles of quantum mechanics to perform calculations much faster than traditional computers. This advancement has opened doors to numerous applications across a variety of fields.
Brief Background and Context
The advent of quantum computing began with the theoretical formulations by pioneers such as Richard Feynman and David Deutsch in the 1980s. They recognized that classical computers could struggle with certain complex problems and proposed a new paradigm. Over recent decades, significant progress has been made, leading to practical applications that challenge conventional computations.
Importance in Current Scientific Landscape
In today’s context, quantum computing is emerging as a crucial player in multiple sectors. Its potential applications extend beyond pure computation to areas such as cryptography, where it may redefine security standards, optimization problems in logistics, new methodologies in drug discovery, and advancements in artificial intelligence. The implications are vast and affect not only technology but also economics and societal norms.
Methodology
Research Design and Approach
A qualitative approach was utilized to analyze existing literature and case studies, providing insights into realized applications of quantum computing. The focus was on academic journals, industry reports, and expert opinions, allowing for a well-rounded understanding of the developments in this field.
Data Collection Techniques
Data was collected through both primary and secondary sources. Primary data included interviews with industry professionals and insights gathered from conferences and seminars related to quantum technologies. Secondary data involved reviewing articles, books, online resources, and databases that discuss various applications and their potential impact.
In essence, this structured approach ensures a comprehensive examination of how quantum computing can intersect with different domains, further driving the research necessary for this evolving field.
Prolusion to Quantum Computing
Quantum computing is fundamentally changing how we understand computational processes and their potential. The emergence of quantum computing signifies a pivotal moment in technology, offering advantages that go beyond what traditional computing systems can achieve.
This section serves as an introduction to the essential elements of quantum computing, laying the groundwork for the detailed discussions to follow. The growing interest in this field can be attributed to its capabilities in handling complex problems much faster than classical computers. For example, quantum computing offers an unprecedented ability to tackle simulations, optimizations, and cryptographic challenges that were previously deemed impractical.
Moreover, quantum computing's impact is felt across various sectors, including finance, healthcare, and artificial intelligence. By harnessing the principles of quantum mechanics, these systems will enhance data processing methodologies and redefine approaches to problem-solving.
As we explore the nuanced landscape of quantum computing, it is important to consider not only its potential applications but also the challenges and limitations that accompany its adoption. The interplay between theory and practical implementation remains a crucial aspect for researchers and industries alike.
Definition and Fundamentals
At its core, quantum computing is based on quantum bits, or qubits. Unlike classical bits, which represent either a 0 or a 1, qubits can exist in a state of superposition. This means that they can represent both 0 and 1 simultaneously. This property allows quantum computers to process a vast amount of information in parallel, leading to significant performance improvements in specific tasks.
Additionally, qubits are entangled, a phenomenon where the state of one qubit is dependent on the state of another, regardless of the distance between them. This entanglement is crucial for the power of quantum computation, allowing for complex correlations that classical systems cannot duplicate.
The fundamental operations performed on qubits are realized through quantum gates, which manipulate the states of qubits to perform calculations. By combining these gates in various configurations, quantum algorithms can be executed, leading to solutions that may outpace classical algorithms.
Historical Context
The concept of quantum computing began to take shape in the early 1980s. Pioneering work by physicist Richard Feynman and computer scientist David Deutsch highlighted the limitations of classical computers for simulating quantum systems. Their insights laid the foundation for what would later evolve into the field of quantum computing.
Throughout the 1990s, researchers such as Peter Shor and Lov Grover introduced groundbreaking algorithms that demonstrated how quantum computers could outperform their classical counterparts in specific applications, such as factoring large numbers and database searching.
The turn of the millennium saw the advent of experimental quantum computing, with various research institutions and companies, such as IBM and Google, beginning to build tangible quantum systems. These advancements paved the way for the present state of the field, characterized by rapid development and interest.
Despite its nascent status, quantum computing is anticipated to revolutionize computing paradigms in the years to come. As we further investigate specific applications, it becomes clear how this technology can address challenges across multiple domains.
Quantum Computing vs Classical Computing
The distinction between quantum computing and classical computing is fundamental to understanding the transformative capabilities of these technologies. Quantum computing represents a significant departure from how we traditionally think about computation. Classical computers use bits as the smallest unit of data, which can either be 0 or 1. In contrast, quantum computers utilize qubits, which can exist in multiple states at once, thanks to a principle called superposition. This allows quantum computers to perform certain calculations much faster than classical computers.
Computational Differences
The computational differences between quantum and classical computing are profound. Classical algorithms often process information sequentially, meaning they handle one operation at a time. This limits the speed and efficiency of calculations, especially as the problem size scales.
Quantum algorithms, such as Shor's algorithm and Grover's algorithm, exploit the unique properties of qubits to perform complex operations simultaneously. For example, Shor's algorithm can factor large integers exponentially faster than the best-known classical algorithms. This capacity shows potential for applications in cryptography, where breaking codes becomes significantly easier with quantum computing.
In essence, while classical computers solve problems in a linear fashion, quantum computers can approach challenges from multiple angles at once, leading to potentially revolutionary advancements in computation.
Speed and Efficiency
The speed and efficiency of quantum computing are not just theoretical concepts; they have real-world implications. Quantum computers can handle complex tasks much quicker than classical systems due to their ability to perform many calculations simultaneously. This is particularly evident in specific fields such as optimization and logistics.
For example, a classical computer might take years to compute the optimal route for delivery trucks across a major city. A quantum computer could theoretically perform this computation in significantly less time, potentially allowing businesses to enhance their operations dramatically. This capability also opens avenues for breakthroughs in scientific research and technology development, driving progress across various industries.
To summarize, the advantages of quantum computing over classical computing lie in its ability to solve problems at an unprecedented scale and speed. This evolution in computing capabilities is not just a mere increment; it represents a leap forward that could redefine how we approach problem-solving across multiple fields.
Key Applications in Cryptography
Cryptography plays a critical role in securing communication and protecting sensitive information in today's digital age. With the rise of quantum computing, the landscape of cryptography is undergoing a significant transformation. Quantum computing can potentially break many of the conventional encryption methods currently in use. This highlights the urgent need for enhanced cryptographic solutions that can withstand quantum threats. The implications of these developments are profound, affecting everything from personal privacy to national security.
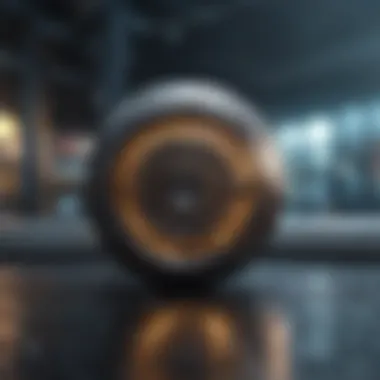
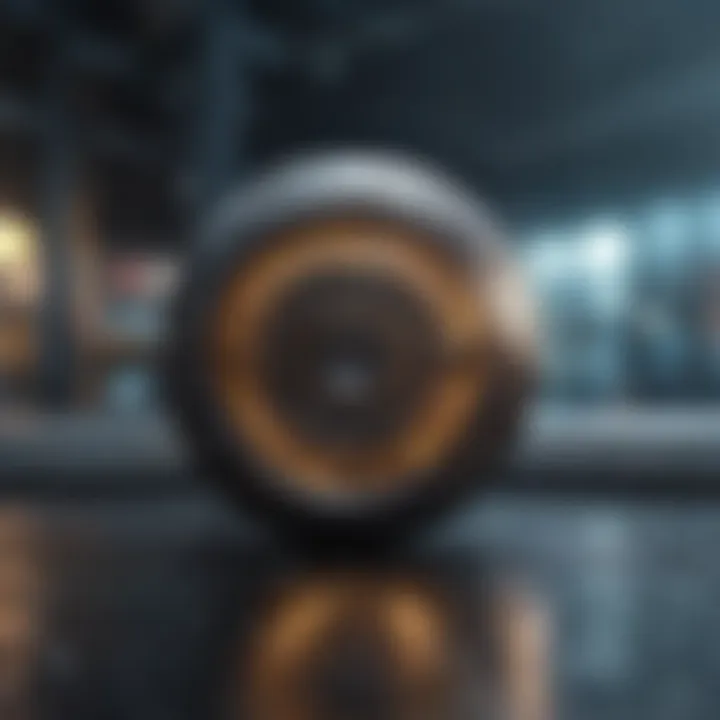
One of the primary benefits of understanding quantum cryptography is that it establishes a framework for fundamentally secure communication. As traditional cryptographic systems, such as RSA or ECC, rely on the computational difficulty of certain mathematical problems, they are vulnerable to attacks from sufficiently powerful quantum computers. Consequently, both researchers and practitioners must consider the unique challenges and advancements presented by quantum computing in the realm of cryptography.
In this section, we will explore two pivotal areas in quantum cryptographic applications: Quantum Key Distribution (QKD) and Post-Quantum Cryptography (PQC). These topics are not merely theoretical; they carry significant real-world implications for how we secure our data.
Quantum Key Distribution
Quantum Key Distribution represents one of the most promising applications of quantum computing in cryptography. It allows two parties to generate a shared, secret key using quantum mechanics principles. The principle behind QKD is simple yet powerful. Any attempt by an eavesdropper to intercept the key exchange will inevitably alter the quantum states being transmitted, thereby betraying their presence.
Some key aspects of Quantum Key Distribution are:
- Security Assurance: QKD provides a level of security that is not achievable with classical methods because it is based on the laws of quantum physics rather than computational assumptions.
- Protocols: Several protocols have been established for QKD, including the BB84 protocol and the E91 protocol. Each has unique advantages and is suitable for different applications.
- Implementation Challenges: While promising, the practical deployment of QKD also faces hurdles. These include transmission distance limitations and technological requirements for integrating QKD with existing networks.
QKD is already being integrated into telecommunications infrastructure in various countries, aiming to provide secured communication channels resistant to eavesdropping.
Post-Quantum Cryptography
Post-Quantum Cryptography refers to cryptographic algorithms that are designed to be secure against quantum attacks. As quantum computers advance, it is essential to develop encryption methods that remain effective even in a quantum computational environment.
Key points regarding Post-Quantum Cryptography include:
- Algorithm Development: Researchers are actively working on developing and standardizing new algorithms that are not susceptible to quantum attacks. Examples include lattice-based cryptography and code-based cryptography.
- Standardization Efforts: NIST is leading initiatives to standardize Post-Quantum Cryptography. These efforts are crucial for ensuring widespread adoption across industry and government sectors.
- Hybrid Approaches: A practical transition may involve hybrid systems that use both quantum-resistant algorithms and traditional algorithms concurrently, ensuring a buffer period as quantum technology matures.
Understanding Post-Quantum Cryptography is vital for organizations that handle sensitive information. It not only prepares them for future threats but also instills confidence in clients and stakeholders regarding data security.
"As we advance toward a quantum future, adapting our cryptographic approaches is not merely advisable; it is essential for safeguarding our digital landscape."
Optimization Problems
The field of optimization is crucial in various domains due to its applicability in making complex decisions efficiently. It involves finding the best solution from a set of possible choices, which can lead to significant cost savings or performance improvements. Quantum computing is well-suited for tackling optimization problems because of its ability to perform calculations on a massive scale and explore solutions simultaneously. This is especially valuable in situations where traditional computing methods struggle to find optimal solutions in a reasonable time.
Traveling Salesman Problem
The Traveling Salesman Problem (TSP) is a classic example in optimization, where the goal is to determine the shortest possible route that visits a set of cities and returns to the origin city. The complexity of this problem grows factorially with the number of cities, making it infeasible for classical algorithms to compute solutions efficiently as the dataset size increases. Quantum computing offers a promising approach to resolve the TSP more efficiently.
Through quantum algorithms, such as the quantum approximate optimization algorithm (QAOA), it can potentially analyze multiple routes simultaneously. This parallel processing power could dramatically reduce the time taken to reach an optimal solution, thus making quantum computers an attractive alternative for solving TSP. Research is ongoing, and early results are promising, signaling a new avenue for logistical planning and route optimization.
Logistics and Supply Chain Management
Logistics and supply chain management involve coordinating the flow of goods from supplier to customer. Optimization problems within this field are numerous, including inventory management, distribution routing, and scheduling. The complexity inherent in supply chains can often lead to suboptimal decisions, where costs escalate and efficiency drops.
Quantum computing provides a pathway to navigate these complexities. By leveraging quantum algorithms, organizations can analyze vast datasets and uncover patterns that would be challenging for classical methods. This includes evaluating numerous supply chain factors—such as demand fluctuations, shipping routes, and resource allocation—simultaneously. The enhanced processing capability could lead to improved forecasting and resource optimization, resulting in cost reductions and higher customer satisfaction.
Quantum computing has the potential to transform logistics and supply chain management by providing insights that were previously difficult to obtain.
In summary, optimization problems are foundational to many industrial operations. With quantum computing's unique ability to handle complex calculations with greater speed and accuracy, it has the potential to fundamentally enhance optimization processes across various applications.
Drug Discovery and Molecular Simulation
The fields of drug discovery and molecular simulation are experiencing a notable transformation through quantum computing. This technology provides a unique capacity to tackle complex molecular phenomena that are traditionally difficult or impossible to model accurately. The integration of quantum mechanics into the simulation process allows researchers to predict molecular interactions with unprecedented precision. This capability can lead to groundbreaking advancements in the development of new therapies and medications.
Simulation of Molecular Systems
Simulating molecular systems accurately is essential for understanding biological processes and drug interactions. Quantum computing specializes in handling quantum states which represent atoms and their interactions. With this approach, scientists can create highly detailed models of how molecules behave under varying conditions.
Key advantages of quantum simulations include:
- Accuracy: Quantum simulations can predict the properties of molecules with much higher accuracy than classical methods, reducing the gap between theoretical predictions and experimental results.
- Complexity: They can efficiently simulate large systems. Classical computers struggle with larger molecules or intricate systems due to their computational limitations. Quantum processors handle this task more seamlessly.
- Optimization: This capability aids pharmaceutical scientists in optimizing drug candidates during the early development phases. They can explore more variables and potential interactions quicker.
Quantum simulations can elucidate reaction mechanisms, binding affinities, and the effects of structural changes on molecular behavior. This depth of insight can profoundly impact predictive modeling and screening efforts.
Accelerated Drug Development
The timeline from drug discovery to market can span years or even decades. Quantum computing has the potential to significantly reduce this timeframe. Two key areas where advancements can be observed include:
- Target Identification: By modeling biological systems and their interactions, quantum computing can facilitate the identification of new drug targets. This step is crucial for developing treatments for diseases that currently lack effective therapies.
- Drug Repurposing: Quantum algorithms can evaluate existing drugs and uncover novel uses for them in treating different diseases. This not only accelerates drug development but also leverages already known compounds, potentially reducing the costs involved.
The overall impact of these applications could redefine how pharmaceutical companies approach drug development, making it more efficient and less costly.
"By harnessing the power of quantum computing, we are entering a new era in drug discovery where speed and precision can lead to truly transformative therapies."
In summary, quantum computing promises to reshape drug discovery and molecular simulations. By improving accuracy in molecular modeling and accelerating various stages of drug development, this technology holds immense potential for medical science. As these techniques mature, they may open pathways to innovative treatments previously thought unattainable.
For further exploration, check resources like Wikipedia or Britannica for more detailed insights into these topics.
Machine Learning and Artificial Intelligence
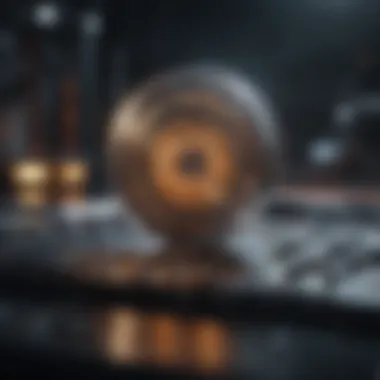
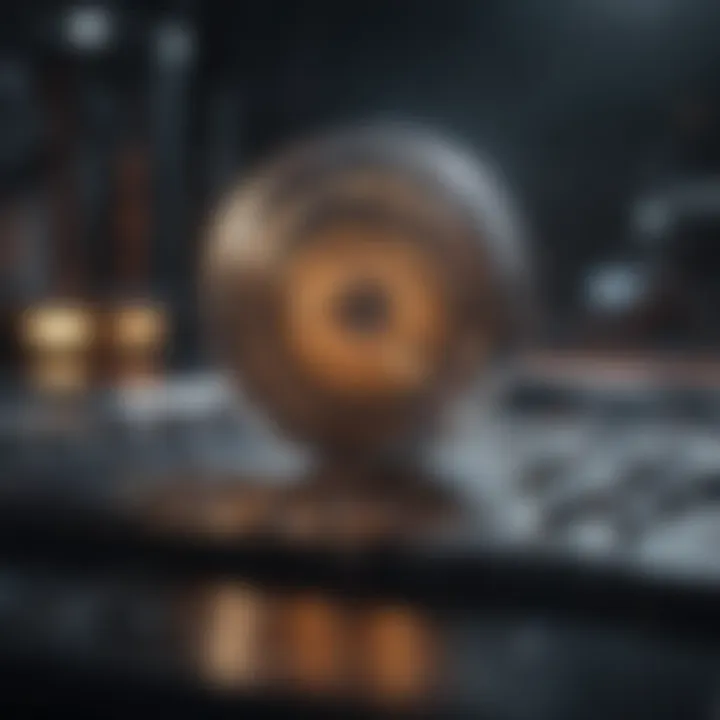
The integration of quantum computing into the domains of machine learning and artificial intelligence heralds a significant shift in computational capabilities. These fields demand processing power that far exceeds what classical computers can offer, especially with large datasets and complex algorithms. Quantum computing's unique properties, such as superposition and entanglement, can potentially accelerate machine learning techniques, leading to more accurate models and enhanced data processing. This section will explore quantum algorithms specifically designed for machine learning and discuss how these innovations can streamline workflows in artificial intelligence applications.
Quantum Algorithms for Machine Learning
Quantum algorithms can dramatically improve the efficiency of various machine learning tasks. Unlike classical algorithms, which process data sequentially, quantum algorithms leverage quantum bits (qubits) to perform many calculations at once. This parallelism opens new possibilities for building and training machine learning models faster than ever before.
A prominent example is the Quantum Support Vector Machine (QSVM), which can classify data in higher-dimensional spaces more efficiently than classical support vector machines. Similarly, quantum k-means clustering presents a quantum-enhanced method for group analysis, improving speed and scalability when processing voluminous datasets.
Some notable quantum algorithms include:
- Quantum Principal Component Analysis (QPCA): This algorithm aims to find the principal components of a dataset with reduced computational resources, making it useful for dimensionality reduction.
- Quantum Neural Networks (QNN): These networks utilize the principles of quantum mechanics to process information in ways that classical neural networks cannot.
"Quantum algorithms can offer exponential speedups in certain machine learning tasks, reshaping how models are trained and optimized."
As researchers continue to develop these algorithms, their practical applications in real-world scenarios will become clearer. The advancement of quantum algorithms provides insights into potential breakthroughs in machine learning, heralding an era of discovery and innovation.
Data Processing Enhancements
The efficiency of data processing is a critical factor in both machine learning and artificial intelligence. Classical data processing methods often struggle with huge datasets, resulting in prolonged processing times and increased cost. Quantum computing offers potential solutions to these challenges through enhanced data processing capabilities.
Quantum computers can tackle data-intensive tasks, like searching through large databases, with remarkable speed. Using Grover's algorithm, quantum computers can search unsorted databases in a time proportional to the square root of the size of the dataset, which is a significant improvement over classical methods.
Some specific data processing enhancements provided by quantum computing include:
- High-dimensional data analysis: Quantum computing allows for simultaneous evaluations across multiple dimensions, improving insights extracted from complex datasets.
- Real-time data processing: The speed of quantum algorithms permits the processing of streaming data in real-time, which is critical in applications like fraud detection and instant decision-making in financial markets.
- Reduction of processing errors: Quantum error correction techniques can help improve the accuracy of data processing tasks, leading to more reliable outcomes in machine learning models.
Integrating quantum computing with data processing workflows can significantly enhance the abilities of machine learning systems. By capitalizing on quantum advancements, researchers and professionals in the field can develop smarter, more efficient artificial intelligence solutions that respond to the ever-evolving challenges of modern data landscapes.
Financial Modeling and Risk Analysis
Financial modeling and risk analysis are critical areas where quantum computing can demonstrate significant advantages. Traditional financial models often struggle with the complexities and vast data that drive financial decision-making in today ’s dynamic environments. Quantum computing can enhance the speed and accuracy of these models, leading to improved predictions and more strategic decisions.
Portfolio Optimization
Portfolio optimization is the process of selecting the best mix of assets to maximize returns while minimizing risk. Classical methods can be computationally intensive, especially with large datasets and numerous variables. Quantum algorithms, such as the Quantum Approximate Optimization Algorithm (QAOA), present an opportunity to enhance this process by evaluating multiple optimal combinations simultaneously.
Benefits of Quantum Portfolio Optimization:
- Speed: Quantum processors can perform calculations at unprecedented speeds. This accelerates the simulation of various market scenarios and investment strategies.
- Precision: Quantum computing can manage complex correlations between various assets more effectively than classical systems. This leads to more precise risk assessments.
- Scalability: The capability to handle larger datasets means that portfolios can be optimized using a more comprehensive set of variables rather than relying on limited historical data.
Risk Assessment Models
Risk assessment models are essential in quantifying the potential losses associated with different investment strategies. Quantum computing enables the analysis of multiple risk factors and scenarios more holistically, making it easier for financial institutions to mitigate risks.
Key Elements of Quantum Risk Assessment:
- Enhanced Simulations: Quantum systems can run simulations of various market conditions faster, leading to more reliable risk predictions.
- Complex Configurations: They can process intricate risk factors and dependencies between assets, which are often too complex for classical computers to handle efficiently.
- Adaptive Learning: As quantum machine learning technology advances, these models can adapt and evolve based on new data, improving accuracy over time.
"Quantum computing possesses the potential to transform the way financial models are built and assessed, making the future of finance smarter and more resilient."
Materials Science and Engineering
The intersection of quantum computing and materials science is a domain of significant interest. This synergy provides the potential to revolutionize how materials are developed, tested, and utilized across various industries. As the demands for material efficiency and performance increase, so does the need for innovative approaches to understanding material properties and behaviors. Quantum computing offers unique advantages here, such as heightened simulation capabilities and the ability to manage complex interactions within materials on a quantum level.
Researchers in materials science are often tasked with predicting how materials will respond to different conditions. Traditional methods can be time-consuming and sometimes inaccurate. With quantum computing, scientists can simulate and analyze molecular interactions much more accurately and quickly. This allows for a more profound understanding of material characteristics, enabling the development of customized materials tailored for specific applications.
Quantum Simulation in Material Design
Quantum simulation refers to the use of quantum computing to model and analyze the properties of materials with extreme precision. This simulation can yield valuable insights into the electronic structure of materials. By modeling these properties accurately, quantum computing facilitates the development of new materials with desirable characteristics, such as increased strength, reduced weight, or enhanced conductivity.
For instance, researchers may need to design superconductors that work at higher temperatures. Traditional approaches to material design involve substantial trial and error. Quantum simulations can significantly streamline this process by allowing researchers to predict which materials might exhibit superconducting properties under specified conditions. This results in faster breakthroughs in material design and application.
Novel Material Discoveries
The potential for quantum computing extends beyond simulation. It also opens the door to discovering entirely new materials that were previously unimaginable. By employing quantum computing techniques, researchers can explore vast material space efficiently, uncovering combinations and structures not yet synthesized in labs.
These discoveries can lead to breakthroughs across a variety of sectors. For example, in renewable energy, finding new materials for solar panels that have better absorption characteristics can lead to more efficient energy solutions. Additionally, in medicine, new biomaterials that integrate better with human tissue can advance biomedical applications.
"The quantum revolution in materials science could unlock novel solutions to many societal challenges, from clean energy to advanced health care."
In summary, the integration of quantum computing into materials science and engineering plays a pivotal role in enhancing our approaches to material design and discovery. As researchers continue to explore the quantum realm, the implications for various industries can lead to significant advancements and innovations.
Telecommunications Advancements
In the realm of quantum computing, telecommunications advancements emerge as a crucial area. The potential for improved communication systems rests on the unique properties of quantum mechanics. These advancements represent not just an upgrade in technology but a shift in the fundamental way data can be transmitted securely and efficiently.
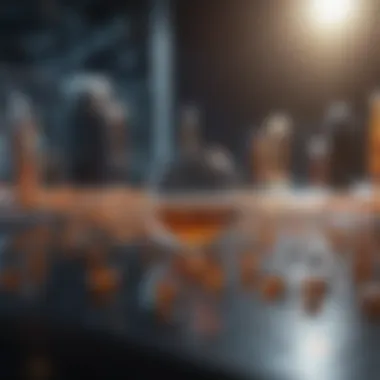
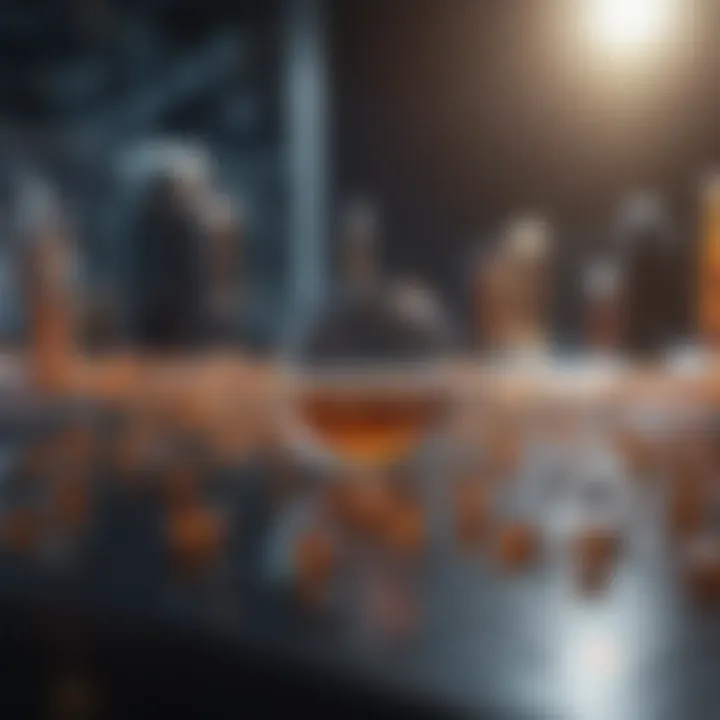
Quantum Communication Networks
Quantum communication networks leverage the principles of quantum mechanics to create highly secure methods for transmitting information. One of the most significant benefits of these networks is their ability to use quantum key distribution (QKD). QKD allows users to share encryption keys securely. Any attempt to eavesdrop disrupts the quantum states of the transmitted particles, thus alerting the communicating parties to potential interception.
This technology ensures that the transmitted data remains private and secure even against powerful adversaries. Aside from security, quantum networks promise increased data transmission speeds over longer distances compared to classical methods. Furthermore, they can facilitate new types of applications that were previously unattainable with classical physics. The development of these networks is critical in a world where data breaches are increasingly common.
"Quantum communication networks could redefine the landscape of secure communications, ensuring confidentiality and integrity in data transmission."
Secure Data Transmission
The challenge of secure data transmission is not new, but quantum computing offers groundbreaking solutions. Traditional encryption methods, while effective, can potentially be broken with the advent of powerful classical computers. Quantum secure transmission addresses these concerns by employing the laws of quantum mechanics.
By utilizing quantum entanglement and superposition, data can be encrypted in such a way that it is practically immune to unauthorized access. Statistical assurances given by quantum physics guarantee the authenticity of messages. Thus, even with the most advanced computational techniques available today, it remains extremely challenging for an unauthorized party to decipher quantum-encrypted data.
Moreover, the integration of quantum secure transmission into existing telecommunications infrastructures is gaining traction. Companies are exploring practical implementations that involve minimal disruption to current systems. This integration could lead to an overall increase in data integrity and trustworthiness across communication platforms.
Challenges and Limitations
The application of quantum computing carries remarkable promise, yet it does not come without its challenges and limitations. Understanding these obstacles is crucial for stakeholders in industry and research. Identifying these hurdles can facilitate strategic planning and resource allocation, ultimately guiding the development of more effective quantum computing solutions. This article section highlights two critical aspects: technical hurdles and scalability issues.
Technical Hurdles
Technical challenges in quantum computing revolve around the maintenance of quantum states and the coherence of qubits. Quantum systems are exceedingly sensitive to their environment, which makes them prone to errors. As a result, achieving a reliable and stable quantum computation often requires complex error correction techniques.
Key aspects of technical hurdles include:
- Decoherence: Quantum information can be lost due to interaction with the environment. This necessitates the development of methods for error correction and fault tolerance.
- Gate Fidelity: The precision with which quantum operations are executed directly affects the accuracy of computations. Improving gate fidelity is vital for practical applications.
- Hardware Limitations: Many quantum devices are still in their infancy. Current quantum processors may have relatively low qubit counts and high error rates, hindering their ability to run large-scale applications.
Efforts to address these technical issues are ongoing and require interdisciplinary collaboration between physicists, engineers, and computer scientists.
Scalability Issues
Scalability represents another significant challenge. Quantum computers, to be truly effective, need to operate on a larger scale than current prototypes. However, scaling up quantum systems presents unique difficulties.
Consider the following factors related to scalability:
- Qubit Interconnectivity: As the number of qubits increases, so does the complexity of controlling and entangling them efficiently. This might lead to increased error rates and computational inaccuracy.
- Resource Requirements: Increased numbers of qubits necessitate more sophisticated supporting infrastructure, which can be expensive and complex to manage.
- Physical Limitations: Current technologies may not be able to support the cooling and isolation requirements needed as qubit counts grow.
The pathway to scalable quantum computing is not straightforward. A successful strategy must involve overcoming these hurdles while also ensuring that the quantum processors can operate reliably at larger scales.
"The road to effective quantum computing is laden with scientific and engineering challenges that must be surmounted to realize its full potential."
Understanding these challenges can inform upcoming research initiatives and development efforts. As researchers tackle these obstacles, the future landscape of quantum computing will gradually become clearer.
Future Prospects of Quantum Computing
The future prospects of quantum computing are not only intriguing but also pivotal for a range of industries and academic disciplines. As quantum computing continues to advance, it promises to redefine problem-solving and data processing techniques. With its ability to manage complex computations efficiently, this technology can transform how we approach various fields, from artificial intelligence to cryptography. The significance of these prospects lies in their potential to unlock capabilities that traditional computing cannot achieve.
Emerging Trends
Emerging trends in quantum computing reflect the rapid evolution of this sector. Several key themes are surfacing which may play a critical role in shaping the future landscape:
- Quantum Hardware Development: Innovations in quantum processors are accelerating. Companies like IBM and Google are leading research to build more stable and scalable quantum systems.
- Cloud-Based Quantum Computing: Accessibility to quantum resources is increasing. Platforms like IBM Q Experience enable users to explore quantum algorithms without owning quantum hardware.
- Integration with Classical Systems: The interoperation of quantum and classical computing environments is gaining traction, enabling hybrid approaches that maximize computational efficiency.
- Standardization and Protocol Development: Efforts to establish standards in quantum algorithms and protocols are essential for widespread adoption, ensuring compatibility and security across platforms.
These trends underline a steady progress toward making quantum computing more practical and accessible.
Potential Industrial Transformations
The industrial applications of quantum computing hold promise for significant transformations across various sectors. The expected impact is profound and multifaceted:
- Pharmaceutical Industry: Quantum computing can revolutionize drug design processes by simulating molecular interactions much faster than conventional methods. This can drastically shorten the timeline for drug development, potentially saving lives and resources.
- Finance Sector: Financial modeling may evolve with quantum algorithms improving risk analysis and asset management. Portfolio optimization could become more accurate, leading to better investment strategies.
- Supply Chain Management: Quantum optimization can enhance logistics by providing solutions to complex routing problems swiftly. This would improve efficiency and reduce costs operationally.
- Telecommunications: The development of quantum communication networks and protocols can lead to unprecedented levels of secure data transmission, crucial for preserving user privacy and protecting sensitive information.
"Quantum computer's ability to solve certain problems exponentially faster has implications that extend beyond theoretical applications; they offer transformative potential for critical industries."
Culmination
In this article, the Conclusion serves as an essential final component that encapsulates the transformative implications of quantum computing across multiple sectors. Understanding the applications of quantum computing is crucial, as it highlights the potential benefits and sets the stage for further advancements in technology.
Summary of Key Insights
The insights presented throughout this article underscore the vast potential of quantum computing. From its capabilities in enhancing cryptographic security through Quantum Key Distribution to the accelerations observed in drug discovery, the landscape of computational problems is evolving. Notably, quantum computing showcases unique advantages in optimization problems, including logistics and financial modeling. This technology, although still developing, promises to revolutionize how we approach complex challenges.
A few key points include:
- Quantum computing provides substantial speed advantages over classical computing for certain tasks.
- Real-world applications are emerging in various fields such as pharmaceuticals, materials science, and finance.
- The potential for future innovation continues to grow, suggesting a must-invest attitude toward research and development in this area.
Call to Action for Research and Development
With the momentum building around quantum computing, it becomes imperative for stakeholders in academia, industry, and government to prioritize research and development. Investments should focus on not just the technology itself but also on its applications in solving real-world problems.
- Encourage collaboration among researchers and institutions.
- Support interdisciplinary studies that link quantum computing with other fields such as artificial intelligence and materials science.
- Advocate for educational programs that prepare the next generation of scientists and engineers to harness quantum technology effectively.
This concerted effort will ensure that society fully realizes the benefits of quantum computing and addresses the challenges that lie ahead.