Understanding the Complexities of Tumor Development
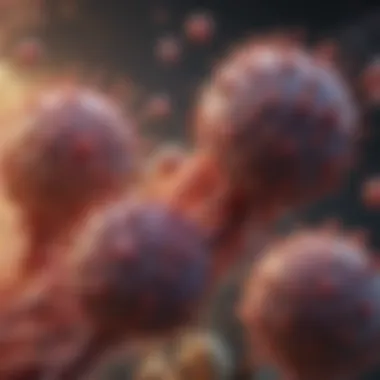
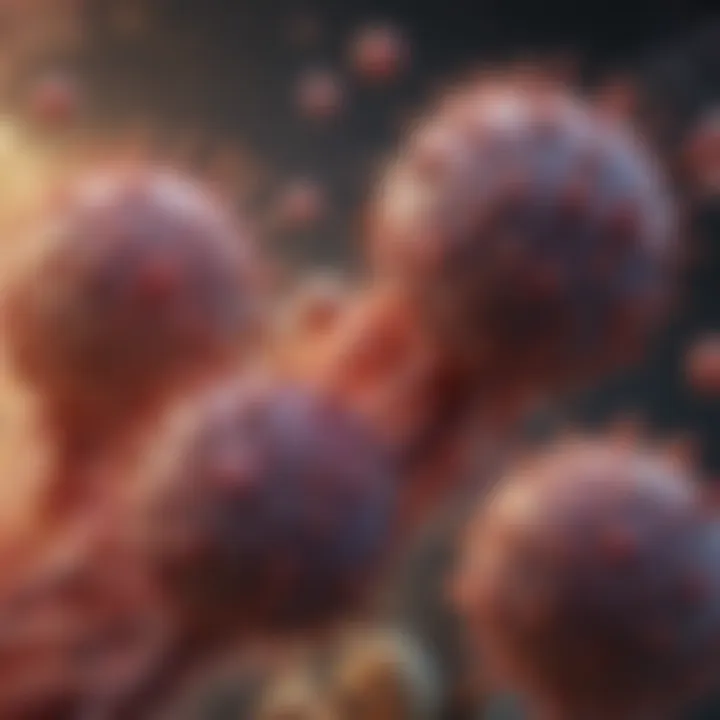
Overview of Research Topic
Brief Background and Context
Tumor development is a complex biological instance that encapsulates a myriad of processes, varying from genetic mutations to environmental factors. Understanding these mechanisms requires a thorough exploration into the intricate interactions between cells and their microenvironment. On a basic level, tumors can be classified into benign and malignant forms, but this bifurcation belies the underlying chaos that fuels cellular proliferation. The formation of tumors encompasses not just genetic changes but also influences from external agents like carcinogens, which can twist the molecular pathways toward uncontrolled growth.
Importance in Current Scientific Landscape
The significance of studying tumor development cannot be overstated. With the global burden of cancer steadily rising, pinpointing the mechanisms that underlie malignancy is essential. Researchers today strive to inform better preventive measures and therapeutic strategies, aiming for more targeted treatments. Clarity around these processes is critical; it lays the groundwork for advancing cancer detection, enhancing personalized medicine, and ultimately improving patient outcomes.
Methodology
Research Design and Approach
A diverse array of methodologies exists within cancer research. Generally, studies may use a combination of laboratory-based experiments, clinical trials, and computational biology. The lures of genomic sequencing technologies have tremendously escalated our understanding of cancer, providing rich data sets upon which to model tumor behavior. Various scientific approaches, such as proteomics and transcriptomics, contribute to offering a 360-degree view of tumor biology, showing how mutations result in altered functions at the protein and gene expression levels.
Data Collection Techniques
Data collection for understanding tumor mechanisms typically involves:
- Tissue biopsies: Examining histopathological samples to identify tumor markers.
- Genetic screening: Identifying mutations, epigenetic changes, and mRNA expression profiles.
- In vivo studies: Using animal models to observe tumor growth patterns and responses to therapies.
- Bioinformatics: Analyzing large-scale datasets to uncover hidden patterns and correlations.
Each technique serves to enhance our grasp of how tumors develop and evolve, forming a cohesive narrative that incorporates both biological and environmental aspects.
"Understanding the mechanisms behind tumor development is not merely an academic exercise but a crucial pursuit that can shape future therapeutic avenues."
As we dive deeper into the complexities of tumor biology, we will delineate how cells navigate these intricate pathways, laying the foundation for a robust discussion on genetic mutations, the impact of the tumor microenvironment, and the broader implications for cancer treatment.
End
Grasping the machinations of tumor development is paramount in the fight against cancer. By stitching together insights from genetics and environment, along with cutting-edge research methodologies, we can better equip ourselves to tackle this multifaceted disease head-on.
Understanding Tumors
Understanding tumors is a cornerstone of cancer biology, providing a window into the intricate processes that dictate cellular growth and proliferation. This knowledge is essential for anyone involved in research, diagnosis, or treatment of cancer. Tumors represent the manifestation of cellular dysregulation, serving as both a symptom and a contributing factor to the disease's progression. By studying tumors, we can discern the underlying mechanisms that lead to their formation and ultimately develop strategies for intervention.
Definition of Tumors
A tumor is fundamentally an abnormal mass of tissue that arises when cells divide more than they should or do not die when they should. Tumors can be broadly classified into two categories: benign and malignant. Benign tumors do not invade nearby tissues or spread to other parts of the body. They tend to grow slowly and resemble normal cells much more closely than malignant tumors. In contrast, malignant tumors harbor the potential to invade surrounding tissues and metastasize to distant sites, often resulting in severe health consequences. Understanding these definitions sets the groundwork for further exploration of tumor behavior and the cellular intricacies involved in their development.
Types of Tumors
Benign Tumors
Benign tumors are often seen as the less troublesome cousins of malignant tumors. One of their key characteristics is that they do not spread beyond their point of origin, making them generally less dangerous. This quality allows for a positive outlook in clinical scenarios where benign tumors are diagnosed. Typically, these tumors can be removed surgically with little risk of recurrence, offering patients a chance of a clean bill of health. A unique feature of benign tumors is their encapsulation—many are surrounded by a fibrous layer that helps keep them contained.
However, they can still interact with surrounding tissues, causing complications depending on their location. For instance, a benign tumor in the brain can exert pressure on vital areas, leading to neurological issues, while one in the skin may remain asymptomatic. The notion that benign tumors are harmless can be misleading, as their management often requires a careful approach.
Malignant Tumors
Malignant tumors are a whole different ball game. They are notorious for their aggressive nature and ability to infiltrate surrounding tissues. The hallmark of malignant tumors is their genetic instability; they can mutate rapidly. This fast-paced change enables them to adapt and evade the body’s immune response, presenting significant challenges to treatment efforts. Typically, these tumors form from the uncontrolled division of abnormal cells and can lead to serious medical conditions, including cancer.
One distinctive feature of malignant tumors is their metastasis. They can shed cells that travel through the bloodstream or lymphatic system, establishing new tumors in various organs. This capability underscores the urgency of early detection and intervention, as the prognosis often worsens with delayed treatment. The complexities involved with malignant tumors not only deepen our understanding of tumor biology but also pose critical questions in therapeutic strategies.
Metastatic Tumors
Metastatic tumors are often viewed as the final stage of malignant growth—a grim testament to cancer's invasive nature. They represent the conquerors of the body’s landscape, having originated from another part of the body before establishing a foothold elsewhere. The characteristic feature of metastatic tumors is their undeniable connection to the primary cancer—they retain the properties of their origins, which aids in diagnostic procedures.
Understanding metastatic tumors is crucial in cancer research and treatment. Their presence often indicates advanced disease and a lower likelihood of successful outcomes. The mechanisms driving metastasis involve a complicated interplay of cellular, molecular, and environmental factors, highlighting the need for ongoing research to uncover pathways that could be targeted for therapeutic advantage.
"By comprehending the types of tumors and their distinctive features, medical professionals can develop more effective strategies tailored to each type's unique characteristics."
In summary, delving into the various tumor types reveals not only their fundamental differences but also their implications for health and disease management. Each tumor presents its challenges, emphasizing the significance of accurate diagnosis and understanding in the overarching narrative of cancer treatment and research.
Cellular Basis of Tumor Development
The cellular basis of tumor development serves as the cornerstone of understanding cancer biology. At its core, cancer disrupts the intricate dance of cellular processes, leading to abnormal growth and potentially dangerous consequences. By examining the cellular mechanisms that underlie tumor initiation and progression, we gain insight into the biological underpinnings of cancer, its mutations, and its capacity to invade adjacent and distant tissues.
In this context, grasping the intricacies of cellular dysregulation—particularly concerning the cell cycle and the pivotal roles played by oncogenes and tumor suppressor genes—becomes paramount. Armed with this knowledge, researchers, clinicians, and educators can pave the way for innovative strategies aimed at combating this insidious disease.
Cell Cycle Dysregulation
Cell cycle regulation is akin to an orchestra conductor who ensures that the musicians play in harmony. When this regulation falters, chaos ensues, leading to uncontrolled cell proliferation—a hallmark of cancer. The cell cycle consists of several phases: G1 (growth), S (DNA synthesis), G2 (preparation for mitosis), and M (mitosis). Each phase must be carefully monitored and regulated to maintain healthy cellular function.
When elements such as cyclins and cyclin-dependent kinases are disrupted, cells can slip into overdrive, ignoring the signals to stop multiplying. This dysregulation, often caused by genetic mutations or external environmental factors, can result in tumor formation. For instance, a malfunction in proteins that ensure checkpoints in the cell cycle can lead to the replication of damaged DNA, setting the stage for cancer development.
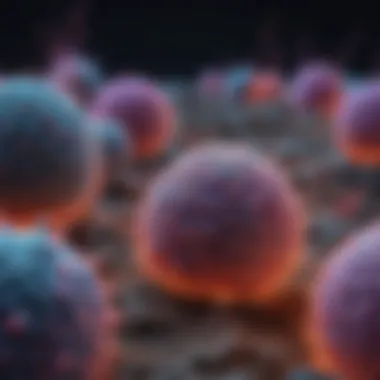
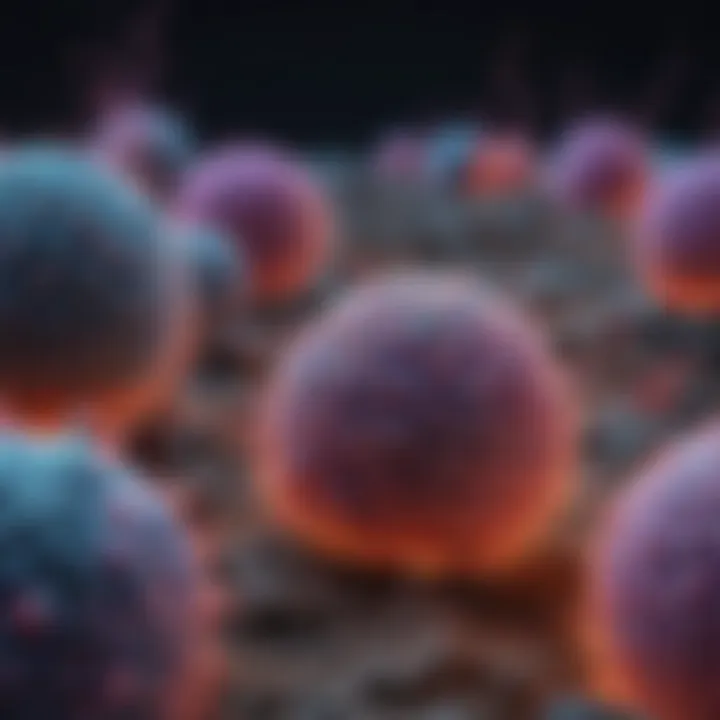
Role of Oncogenes and Tumor Suppressor Genes
Understanding the roles of oncogenes and tumor suppressor genes unfolds further depth in comprehending tumor development. Both types of genes contribute fundamentally to the regulation of cell growth and division, but they do so in starkly different ways.
Oncogenes: A Driver of Cancer
Oncogenes can be seen as the proverbial accelerators of cancer. Normally dormant or responsible for promoting cell growth, when these genes are mutated or overactive, they can push the cell beyond its natural limits. Common examples include the RAS gene family, which, when mutated, can lock a cell in a perpetual state of proliferation. This state poses a major question: how much is too much growth?
The unique feature of oncogenes is their potential to drive cells toward malignancy. The most significant advantage of discussing oncogenes in this article lies in their transformative potential. By understanding how these genes operate and cause cellular malfunction, researchers can work on targeted therapies that inhibit their functions, offering hope in the fight against cancer.
Functions of Tumor Suppressor Genes
Contrastingly, tumor suppressor genes act more like braking systems for cellular growth. Their primary job is to monitor cell division and repair DNA errors or prompt apoptosis (programmed cell death) when necessary. The most well-known tumor suppressor gene, TP53, encodes a protein responsible for maintaining the integrity of the genome. When this gene is compromised, cellular safeguards collapse, leading to unchecked cell division.
A key characteristic of tumor suppressor genes is their preventative nature. They serve as guardians that work to ensure cellular harmony, and this function is critically relevant for our understanding of tumorigenesis. By fortifying our appreciation of these genes, we can identify new avenues for treatments that could restore normal function to these vital controls. In short, both oncogenes and tumor suppressor genes play pivotal roles in cellular mechanisms, and their dysfunction contributes directly to tumor development.
"Understanding the interplay between oncogenes and tumor suppressor genes is crucial for deciphering the genetic languages of cancer."
In summary, the cellular basis of tumor development encapsulates a complex interplay of regulatory mechanisms governed by both oncogenes and tumor suppressor genes, alongside the regulation of the cell cycle. Recognizing the impacts of mutations and environmental influences enhances our overall understanding of cancer's mechanisms, guiding future research and treatment strategies.
Genetic Mutations and Alterations
Understanding genetic mutations and alterations provides a pivotal insight into the intricate world of tumor development. Genetic changes in cells can instigate a cascade of effects, influencing everything from cell function to the overall behavior of a tumor. Essentially, these mutations lead the way to tumor formation and progression, making it essential to delve into their types and characteristics. By unpacking the complexities of genetic mutations, one can appreciate how they foster tumorigenesis and inform treatment strategies.
Types of Genetic Mutations
Point Mutations
Point mutations, perhaps the most common type of genetic alteration, involve a change in a single base pair in the DNA sequence. They can come in various forms, including missense mutations, where one amino acid is replaced by another, resulting in a potentially dysfunctional protein. The key characteristic of point mutations lies in their simplicity; just a single nucleotide change can lead to significant biological consequences. They are beneficial to this discussion because their role in cancer development has been widely studied, showing clear links between specific point mutations and certain types of tumors.
An intriguing feature of point mutations is their frequency in oncogenes, where they can transform normal genes into cancer-promoting entities. This characteristic provides a double-edged sword: on one hand, they can provide insights into targeted therapies, while on the other, they underscore how easily a cell can be led astray with just a slight modification.
Insertions and Deletions
Insertions and deletions, collectively termed indels, represent another layer of genetic mutations. These changes occur when extra base pairs are added to or removed from the DNA sequence. They are unique in that they can lead to frameshift mutations, altering the reading frame of the gene and ultimately impacting the resultant protein significantly. This unpredictability poses challenges; while precise alterations (like point mutations) might lead to specific outcomes, indels can have sweeping implications for gene function.
The conceptual intricacy of insertions and deletions makes them a notable choice in discussions surrounding tumor development. Their potential for causing large-scale disruptions in genetic coding means that they can contribute to the tumorigenic process in ways that are less straightforward than other mutations. However, understanding their role remains a priority—grasping how they factor into genetic instability is vital in comprehending the broader context of cancer biology.
Chromosomal Abnormalities
Diving deeper, chromosomal abnormalities broaden our perspective on genetic mutations. Involving changes to the structure or number of chromosomes, these abnormalities can lead to significant dysregulation of gene expression. A hallmark of many cancers, these alterations can manifest as duplications, deletions, or even whole-genome duplications. Their capacity to disrupt entire genetic landscapes makes them compelling but also raises complexities in their analysis.
A unique feature of chromosomal abnormalities is their broad impact on tumor cell behavior. They can create an environment conducive to tumor progression, stirring up a melting pot of genetic chaos. Despite being considered a challenge in treatment due to their heterogeneity, these chromosomal changes also open new avenues for targeted therapies. Acknowledging their importance is key; their role in oncogenesis cannot be overstated, reminding us that genetic alterations arise at various levels of complexity.
Inherited Genetic Factors
The implications of inherited genetic factors cannot be overlooked as they provide crucial context in tumor development. These factors pertain to hereditary mutations passed down through generations, which can significantly elevate cancer risk. Familiarity with inherited factors allows for better risk assessment and the potential development of preventative strategies. Understanding how certain genetic traits predispose individuals to cancer broadens the scope of cancer research and highlights the necessity for individualized approaches in patient care.
"Genetic understanding lays the groundwork for preventative measures that can save lives."
Recognizing the interplay between genetic mutations and inherited traits ultimately underlines the multifaceted nature of tumor development. It steers research efforts in a direction that considers both hereditary risks and environmental factors, thereby enriching our comprehension of cancer as a whole.
Environmental and Lifestyle Influences
The factors that surround us and the choices we make in our daily lives significantly impact tumor development. A growing body of research underscores the connections between environmental exposures, lifestyle choices, and cancer risk. Understanding these influences offers valuable insights into how we might mitigate risks and promote better health outcomes.
Various elements play into this, including exposure to carcinogens, dietary habits, and levels of physical activity. Carcinogens like tobacco smoke, certain chemicals, and even some viruses can initiate or exacerbate tumor formation. Meanwhile, what we eat—or don’t eat—can either fortify our defense against cancer or lay the groundwork for its development. Physical activity emerges as another key player. Inactive lifestyles can lead to obesity, which is itself linked to various cancers.
Taking these factors together, the landscape of cancer prevention starts to take shape, framing an intricate web of prevention strategies based on our environments and choices.
Carcinogens and Their Mechanisms
Carcinogens are substances that are known to cause cancer. They can be found in a myriad of everyday items, from household cleaning products to industrial pollutants. Their mechanisms often involve altering cellular DNA, leading to mutations that can drive tumorigenesis.
Common types of carcinogens include:
- Chemical Carcinogens: These are typically found in tobacco smoke, certain pesticides, and even processed foods. For instance, benzene, a solvent used in many industrial processes, has been linked to blood cancers.
- Physical Carcinogens: Exposure to certain types of radiation, such as ultraviolet (UV) rays from the sun, can lead to skin cancer.
- Biological Carcinogens: Certain viruses and bacteria, like Human Papillomavirus (HPV) and Hepatitis B, are known to influence the development of specific cancers.
The mechanisms through which these carcinogens operate often involve the formation of reactive oxygen species (ROS) and the disruption of normal cellular functions. Understanding these mechanisms gives valuable clues to developing targeted interventions.
Impact of Diet and Nutrition
Diet plays a fundamental role in shaping our body’s resilience or susceptibility to cancer. A well-nourished body is better equipped to combat diseases, while poor nutrition can lead to deficiencies that weaken the immune system and promote tumor growth.
Certain dietary patterns have been associated with cancer risk. For example:
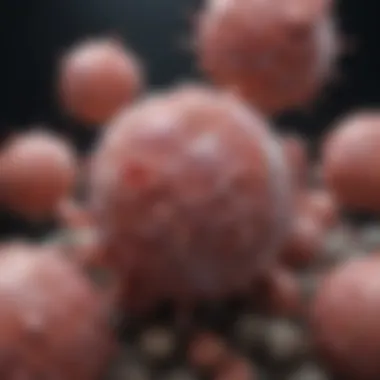
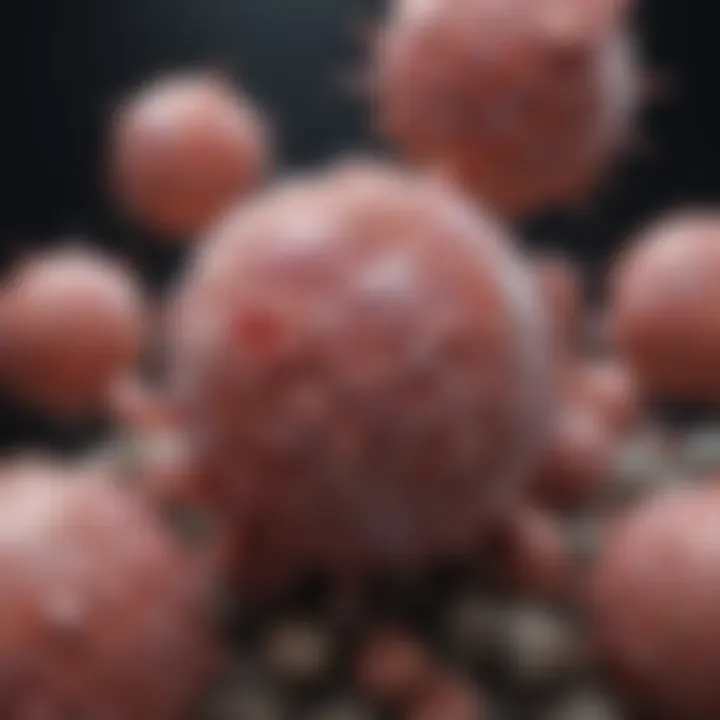
- High-Fat Diets: Diets rich in saturated fats can lead to obesity, a known risk factor for several kinds of cancer.
- Processed Foods: These often contain additives and preservatives that may carry carcinogenic potential.
- Antioxidant-Rich Foods: Fruits and vegetables, especially those high in antioxidants, can neutralize free radicals, thus reducing cellular damage.
The food pyramid is gradually changing, with a focus on incorporating more plant-based foods while minimizing processed options. What you eat doesn’t just impact your weight; it can also alter your body’s biochemical environment, providing a potential catalyst for tumor development or prevention.
Physical Activity and Cancer Risk
Regular physical activity is another cornerstone of health that can influence cancer risk. Research suggests that an active lifestyle can reduce the risk of several cancers, particularly breast and colon cancer. Exercise is thought to help regulate hormones, improve immune function, and maintain a healthy weight—all of which are crucial for lowering cancer risk.
Consider the benefits:
- Weight Management: Keeping a healthy weight can reduce the likelihood of developing cancer.
- Hormonal Balance: Exercise helps balance hormones that can affect tumor growth.
- Immune Function: Physical activity can enhance immune surveillance, allowing the body to respond more effectively to abnormal cells.
Importantly, it’s not a matter of intense workouts alone; even moderate activities, like walking or cycling, can yield beneficial outcomes. Simple lifestyle changes, like taking the stairs instead of the elevator or engaging in social sports, can create a substantial impact on cancer risk over time.
"Active living is not just a trend; it’s a long-term investment in health and well-being, pivotal for lowering cancer risk."
Each of these elements—carcinogens, diet, and physical activity—intersect through intricate pathways that collectively influence tumor development. Recognizing their roles is key to devising more effective prevention strategies. By making informed decisions about our environments and lifestyles, we take a crucial step not just towards cancer prevention, but towards overall health.
The Tumor Microenvironment
The tumor microenvironment encompasses the cellular environment surrounding a tumor, which includes various cell types, signaling molecules, and extracellular components. This environment plays a crucial role in tumor behavior, influencing how tumors develop, progress, and respond to treatment. The interplay between the tumor cells and their microenvironment is indeed a two-way street; tumor cells can alter their surroundings to promote survival and growth, while the microenvironment can drive tumor evolution through dynamic interactions. Therefore, understanding the tumor microenvironment is vital for advancing cancer research and improving therapeutic strategies.
Components of the Microenvironment
Stroma
Stroma is the supportive tissue that provides a structural framework for the tumor. It comprises a mix of connective tissues, blood vessels, and immune cells. A key characteristic of stroma is its ability to influence tumor progression through various pathways. While the stroma can provide necessary nutrients and support for tumor growth, it can also harbor immune cells that may either suppress or enhance tumor activities.
One unique feature of stroma is its dynamic nature; it changes its composition and characteristics as the tumor evolves. This fluidity presents advantages, such as aiding tumor resilience, but it can also be a disadvantage when it leads to having an overtly permissive environment that allows aggressive tumor behavior to thrive.
Immune Cells
Immune cells are pivotal actors in the tumor microenvironment, influencing both the immune responses and the progression of cancer. Different immune cell types, including T cells, macrophages, and dendritic cells, can either protect against tumor growth or facilitate it. One of the key aspects of immune cells is their plasticity; they can adapt their function based on the signals they receive from the tumor and the surrounding microenvironment.
This adaptability can lead to the recruitment of regulatory T cells that suppress anti-tumor activity, allowing the tumor to avoid immune detection. Furthermore, immune cell infiltration can create a conducive environment for tumor metastasis, thus illustrating the dual role immune cells can play.
Extracellular Matrix
The extracellular matrix (ECM) is a complex network of proteins and carbohydrates that fills the spaces between cells in tissues. In the context of tumors, the ECM is not just a passive scaffold but actively participates in signaling pathways that regulate tumor behavior. A notable characteristic of the ECM is its ability to modulate cellular responses through mechanical and biochemical signals.
The ECM’s composition can vary greatly across different tumors, impacting their stiffness and adhesive properties. This variability is a double-edged sword; while a stiff ECM can promote tumor progression by enhancing invasion, it can also inhibit effective treatment delivery. Therefore, understanding the ECM's role in the tumor microenvironment is critical for developing therapeutic strategies that aim to disrupt the supportive framework that tumors utilize for growth and spread.
Interactions Between Tumor Cells and the Microenvironment
The interactions between tumor cells and their microenvironment are complex and multifaceted. Tumor cells can secrete factors that alter the composition of the microenvironment, creating a niche that supports their growth. For instance, tumor cells may release signals that attract immune cells to the site, which can either enhance immune suppression or cause inflammation that can aid tumor growth.
This interplay is not just about direct signaling but also involves feedback loops. As the microenvironment changes in response to the tumor, the tumor cells adapt, leading to a continuous cycle of interaction. Such dynamics complicate therapeutic approaches, as targeting specific components of the microenvironment may inadvertently affect tumor growth or survival. This underscores the necessity for a holistic approach when considering interventions aimed at the tumor microenvironment.
Tumor Progression and Metastasis
Tumor progression and metastasis are central themes in understanding the overall mechanics of cancer development. These processes not only shed light on how tumors grow and invade new territories within the body but also inform treatment strategies and prognostic evaluations. Recognizing the dynamics of tumor behavior provides a foundational knowledge for anyone involved in cancer research, clinical practice, or education. Moreover, it enhances awareness regarding how certain tumors can defy local limitations, spreading rapidly and making treatment significantly more challenging.
The progression of a tumor can often be likened to a marathon, rather than a sprint. It involves several stages, each characterized by different biological behaviors and cellular adaptations. Similarly, metastasis can be seen as the final, dangerous act of this marathon, where tumor cells break free from their primary site, travel through the bodily systems and establish secondary growths in distant organs. The investigation of these processes opens doors to innovations in cancer management that could change patient outcomes effectively.
Stages of Tumor Progression
Understanding the stages of tumor progression is a little like peeling an onion; each layer reveals complexities and nuances pivotal to the overall narrative of tumor development. Generally, tumor progression is categorized into several stages:
- Initiation: This is when genetic mutations occur, triggered by internal or external factors. Cells begin to lose their normal regulatory mechanisms, setting the scene for possible tumor formation.
- Promotion: During this stage, clinically observable changes happen as mutated cells proliferate. Environmental factors can amplify this phase as certain lifestyle or dietary influences may exacerbate growth.
- Progression: Here, the tumor's heterogeneity emerges, marked by a diverse cellular population with varying characteristics. This diversity allows cells to adapt, survive, and resist therapeutic efforts.
- Metastasis: The culmination of tumor progression lies in its ability to spread. Tumor cells enter circulation, invade new tissues, and establish colonies, complicating treatment protocols and potentially leading to patient deterioration.
These stages illustrate how tumors evolve over time and emphasize the cumulative nature of the changes that fuel their growth and spread. Each point in this sequence represents a potential intervention target for treatments aimed at halting cancer in its tracks.
Mechanisms of Metastasis
Invasion
Invasion marks the first major leap in the metastasis journey, wherein cancer cells break through the boundaries of surrounding tissues. This process is a real game-changer, as it determines the tumor's potential to spread far beyond its initial niche. One key characteristic of invasion is the ability of tumor cells to degrade the extracellular matrix, allowing them to squeeze through the tissue barriers. This capability is not simply about moving; it represents a biological adaptability that changes the landscape of local tissues.
The unique feature of invasion is its reliance on a cocktail of enzymes, notably matrix metalloproteinases, which remodel tissues around the tumor. This enzymatic activity has its upsides and downsides. On the one hand, it facilitates the movement of cancer cells; on the other hand, it can also trigger host immune responses that could either hinder or promote tumor growth. Hence, understanding the invasiveness of tumors is crucial—it might offer pathways to develop therapies designed to obstruct this initial escape route.
Circulation and Colonization
Once tumor cells have invaded their immediate surroundings, they embark on their next challenge: circulation and colonization. This process involves the migration of cancer cells through the bloodstream or lymphatic system, making their way to distant sites where they can form new tumors. A key characteristic of this stage is the ability of tumor cells to survive the hostile conditions they encounter in the circulation, such as immune attacks and shear stress from flowing blood.
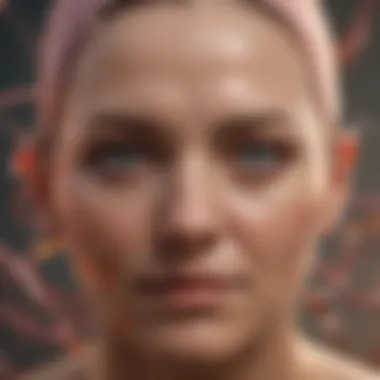
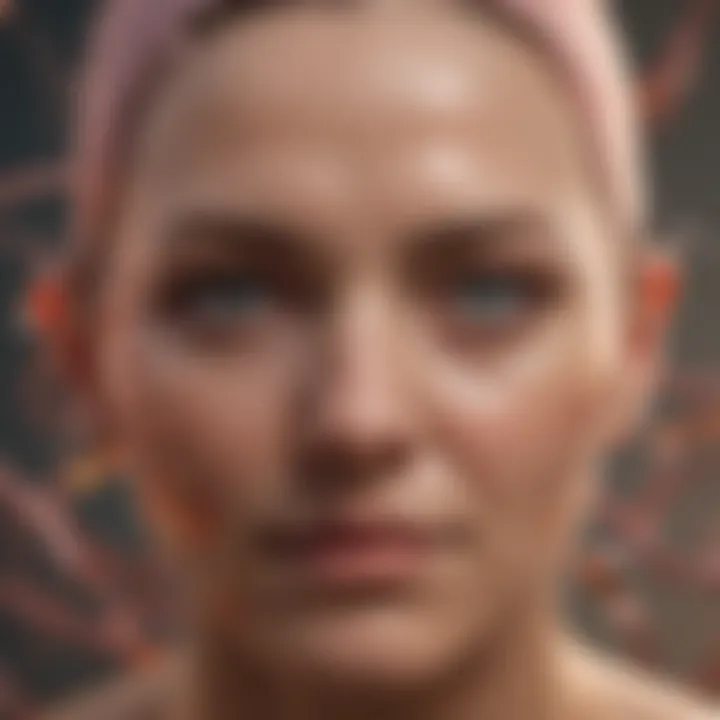
Circulation manages to broaden the potential impact of any tumor remarkably. However, the odds are largely stacked against these wandering cells. Very few survive the journey, but those that succeed are equipped with specific traits allowing them to lodge into new environments successfully. This facet offers a unique angle for researchers; if we can comprehend how certain cells manage to colonize successfully, we might devise strategies for hindering metastatic spread altogether.
Tumor Heterogeneity
Tumor heterogeneity is a crucial concept in understanding cancer. It's about recognizing that tumors are not uniform entities but instead exhibit a wide range of variations. This variability has implications for diagnosis, treatment, and prognosis in oncology. Cancer’s ability to adapt and change means that no two tumors are exactly alike, even if they originate in the same organ. This notion underscores the complexity of cancer biology and the need for personalized treatment approaches.
Another angle to appreciate is how heterogeneity affects tumor behavior. It plays a major role in how tumors respond to therapies, often leading to treatment resistance. As such, getting a handle on tumor heterogeneity gives insights not just into tumor growth, but also how to combat it effectively.
Definition and Importance
The term tumor heterogeneity refers to the genetic and phenotypic diversity found within a tumor and between tumors of the same site. This variation stems from several factors, such as genetic mutations, environmental influences, and selective pressures within the tumor microenvironment. Acknowledging this diversity is important for several reasons. It can inform treatment decisions, enhance our understanding of disease progression, and direct future research avenues. Without a clear grasp of heterogeneity, therapies might miss the mark, failing to address the specific characteristics of the tumor that could dictate its behavior.
Types of Heterogeneity
Within the realm of tumor heterogeneity, two primary categories emerge: genetic heterogeneity and phenotypic heterogeneity. Each of these offers unique insights into the mechanisms that drive tumor development and persistence.
Genetic Heterogeneity
Genetic heterogeneity involves the presence of a variety of genetic mutations within a single tumor. Each subset of tumor cells can possess distinct genetic alterations, which can fuel their growth and resistance to treatment. The key characteristic of genetic heterogeneity is its role in cancer evolution, allowing parts of the tumor to adapt to various environmental stresses, including chemotherapy. This adaptation can lead to tumor recurrence after treatment, making it a critical aspect to study in oncology.
From a research standpoint, understanding genetic heterogeneity opens avenues for targeted therapies. Since different mutations can drive different characteristics of cancer, treatments that are tailored to these mutations show better outcomes. However, this genetic diversity also presents challenges, as it complicates diagnosis and can hinder the development of effective therapies if not carefully considered.
Phenotypic Heterogeneity
On the other hand, phenotypic heterogeneity refers to the observable physical differences between tumor cells that arise from similar genetic backgrounds. This can include variations in size, shape, and behavior of tumor cells. The key characteristic here is that it reflects how different parts of a tumor may carry out distinct functions, potentially influencing their capacity to invade and metastasize.
The unique feature of phenotypic heterogeneity is seen in how it may affect treatment responses. Some cells might be more aggressive than others, which can lead to inconsistent responses to therapies. This aspect is a double-edged sword; while it complicates treatment, it also provides a rich area for research on how to effectively target different tumor characteristics.
"Understanding tumor heterogeneity is key not just for a comprehensive view of how cancers behave, but also for designing effective treatment strategies that can adapt as tumors evolve."
In summary, both genetic and phenotypic heterogeneity are critical components in painting a full picture of tumor behavior. They contribute to the complexities observed in treatment responses and disease progression, emphasizing the need for personalized approaches in cancer therapy.
Implications for Cancer Treatment
The implications for cancer treatment are profound and multifaceted. Understanding how tumors develop opens several doors in the realm of therapy, offering insights that can drastically change patient outcomes. With cancers becoming a leading cause of death globally, the urgent need for effective treatment strategies cannot be overstated. Insights into tumor biology lead to targeted interventions, potentially reducing side effects and improving efficacy.
Key Aspects:
- Personalized Medicine: Knowing how tumors adapt and respond to treatments lets healthcare providers tailor therapies.
- Early Detection: Understanding tumor markers can foster methods for earlier diagnosis, improving survival rates.
- Long-Term Management: Insight into tumor behavior assists in developing strategies for long-term surveillance and intervention.
Targeted Therapy
Targeted therapy represents a surge of innovation, fundamentally changing the way we approach cancer treatment. Unlike traditional chemotherapy—which indiscriminately attacks all rapidly dividing cells—targeted therapies hone in on specific molecular targets within tumors. This specificity aims to spare healthy cells and focuses solely on malignant processes.
- Mechanism of Action: Targeted therapies often involve small molecules that interfere with specific pathways and processes crucial for tumor growth and survival.
- Examples: Common targeted therapies include trastuzumab for HER2-positive breast cancer and imatinib for chronic myeloid leukemia.
- Benefits: Patients often experience fewer side effects and improved survival rates when treated with targeted therapies.
Immunotherapy Approaches
Immunotherapy has emerged as a revolutionary concept in treating cancers that harnesses the body’s immune system to fight tumors. This is akin to unlocking a secret weapon that the body already possesses.
- Types of Immunotherapy:
- Success Stories: Noteworthy successes include treatment for melanoma and certain leukemias, where immunotherapies have led to durable remission in patients who previously had poor prognoses.
- Continued Research: As scientists learn more about the immune response, new combinations and treatment protocols are being developed to maximize this approach.
- Checkpoint Inhibitors: Drugs like pembrolizumab block proteins that inhibit immune responses, allowing T-cells to recognize and destroy cancer cells more effectively.
- CAR T-Cell Therapy: Involves modifying a patient’s T-cells to better recognize and kill cancer cells.
Challenges in Treatment
Despite the promising avenues available through advanced therapy options, cancer treatment retains a basket of hurdles. These challenges must be navigated with care.
- Tumor Heterogeneity: Tumors can vary markedly even within the same patient, leading to unpredictable responses to therapies.
- Resistance Mechanisms: Cancers often develop resistance to targeted therapies, necessitating ongoing adjustments in treatment plans.
- Side Effects: While targeted therapies and immunotherapy generally offer reduced side effects compared to traditional methods, they are not without risks. Autoimmune reactions, for instance, can occur in immunotherapy.
- Cost and Accessibility: New therapies often come with high costs, creating inequity in treatment access among different populations.
"Understanding the unique aspects of tumor biology is critical in navigating the road towards effective cancer treatment. Each patient presents a different puzzle to solve."
Future Directions in Tumor Research
Cancer research is a vast and ever-evolving field. It’s crucial that we continually seek innovative approaches to understand and combat tumor development. As we untangle the complex mechanisms behind tumors, new avenues emerge that offer promise for more effective treatments and preventative measures. This section highlights the importance of exploring future directions in tumor research. By doing so, we can harness cutting-edge technology and findings for improved patient outcomes and enhanced knowledge in the realm of oncology.
Emerging Technologies
The advent of new technologies has substantially changed the landscape of cancer research. Tools such as next-generation sequencing (NGS), artificial intelligence (AI), and CRISPR gene editing are at the forefront of these advancements. NGS enables researchers to analyze genetic mutations in a more comprehensive way than ever before, allowing for the identification of unique genetic signatures associated with specific tumor types.
- Artificial Intelligence: AI can sift through massive datasets to spot patterns that might be missed by human analysis. It assists in predicting patient responses to therapies based on their genetic profiles, thus personalizing treatment plans.
- CRISPR: This groundbreaking technology allows for precise editing of DNA. Researchers are exploring its potential not only to correct mutations but also to enhance immune responses against tumors.
These technologies offer the chance to identify novel therapeutic targets and develop personalized treatments that can adapt to the unique characteristics of each patient’s tumor.
"The future of tumor research lies in our ability to incorporate technological advancements into clinical applications, ultimately bridging the gap between laboratory discoveries and patient care."
Preventive Strategies
Effective cancer prevention strategies are vital for reducing incidence rates and improving public health outcomes. Future directions in preventive research involve several key considerations that can make a significant difference:
- Screening Methods: Development of improved screening techniques that can detect tumors at an earlier stage could drastically change prognosis. Liquid biopsies, which analyze biomarkers from a blood sample, are a promising area that researchers are actively pursuing.
- Lifestyle Interventions: Education on lifestyle factors such as diet, exercise, and smoking cessation can play a major role in preventing tumor development. Research is delving into how specific diets or supplements can modulate cancer risk.
- Vaccination: For certain cancers, like cervical cancer and liver cancer, vaccines have shown effectiveness in prevention. Exploring the development of more targeted vaccines could be a game-changer in reducing the burden of cancer in at-risk populations.
To sum up, the interplay between emerging technologies and preventive strategies could pave the way for a new era in cancer research, enabling us to not just treat tumors but to prevent them from forming in the first place. Exciting possibilities await in the quest to outsmart cancer.