Understanding T7 RNA Polymerase Processivity
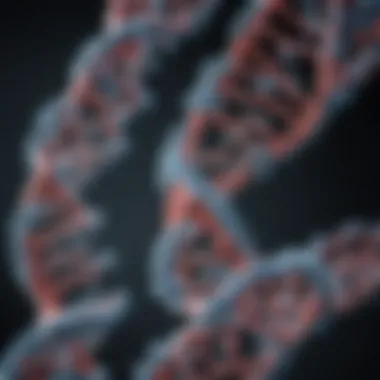
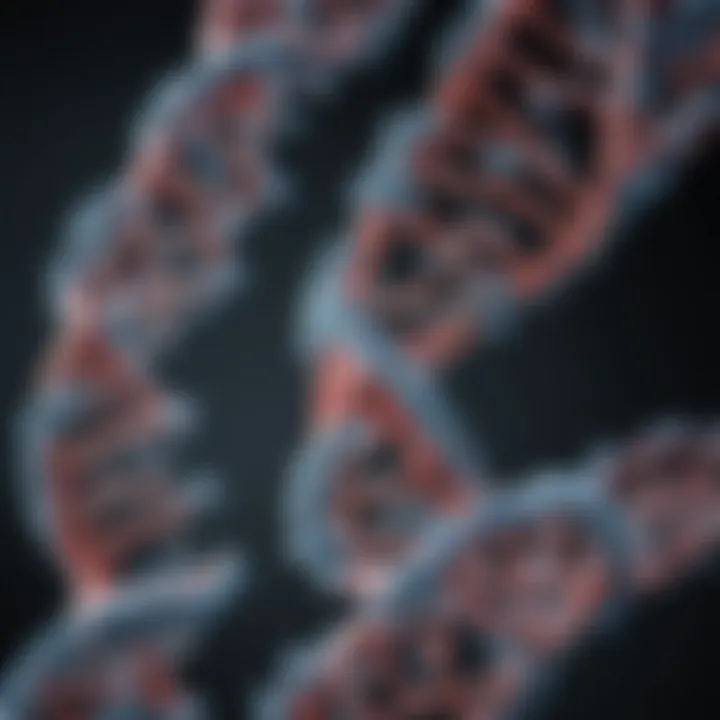
Intro
T7 RNA polymerase (T7 RNAP) has attracted the attention of scientists due to its remarkable characteristics, particularly its high processivity during transcription. This enzyme, derived from the bacteriophage T7, holds a prominent place in molecular biology and biotechnology, serving as a key player in RNA synthesis. As we navigate through this article, we will unpack the molecular intricacies that empower T7 RNAP's efficiency and accuracy, while highlighting its significant applications.
Overview of Research Topic
Brief Background and Context
Understanding how T7 RNA polymerase functions goes beyond mere curiosity; it's a fundamental piece of the puzzle in genetic engineering and therapeutic development. Historically, T7 RNAP was recognized for its ability to initiate transcription with minimal need for additional factors. This inherent property has made it an exceptionally useful tool in crafting synthetic RNA. From simple in vitro transcription systems to more advanced applications, T7 RNAP's efficiency is hardly matched.
Importance in Current Scientific Landscape
The importance of T7 RNA polymerase cannot be understated. In recent years, researchers have leaned heavily on its capabilities to produce high yields of RNA, facilitating advancements in vaccine development, gene therapy, and other biotech applications. With the ongoing excitement surrounding mRNA technology, particularly in light of recent public health challenges, the role of T7 RNAP has taken on newfound significance.
Understanding Processivity
At its core, processivity refers to the enzyme's ability to catalyze consecutive reactions without releasing its substrate. T7 RNA polymerase showcases an impressive level of processivity due to its unique structural elements. The enzyme maintains a stable attachment to the DNA template as it synthesizes RNA, leading to a high rate of transcription. Surprisingly, it can add thousands of nucleotides in a single binding event, making it stand out among other polymerases.
"T7 RNA polymerase can execute long RNA transcripts with remarkable speed and accuracy, often producing full-length RNA molecules in minutes."
Methodology
Research Design and Approach
This article approaches the exploration of T7 RNA polymerase's processivity through a blend of literature review and theoretical analysis. By integrating various primary studies alongside biochemical evidence, the aim is to outline the fundamental mechanisms that dictate T7 RNAP’s activity.
Data Collection Techniques
Data is gathered through multiple channels, including:
- Primary research articles focusing on biochemical assays of T7 RNA polymerase.
- Reviews that compile existing knowledge about the enzyme’s structure and function.
- Experimental data showcasing RNA synthesis rates under various conditions.
Through this rigorous methodology, we aim to paint a detailed picture of T7 RNA polymerase's functional prowess. By the end of this article, readers will have gained a substantial understanding of why T7 RNA polymerase is not just another enzyme, but a cornerstone of molecular biology.
Prolusion
Transcription is one of the fundamental processes in molecular biology, enabling the synthesis of RNA from a DNA template. T7 RNA polymerase, an enzyme derived from the T7 bacteriophage, plays a pivotal role in this process. Its unique ability to synthesize RNA with high efficiency and fidelity makes it a valuable tool in various research and biotechnological applications. Understanding its processivity—the ability to continuously synthesize RNA without releasing the DNA template—is crucial for grasping how it operates in the cellular environment.
The importance of focusing on T7 RNA polymerase stems from its widespread use in laboratory settings. Researchers use this enzyme for synthesizing large quantities of RNA that are essential for various experiments, including in vitro transcription assays, RNA sequencing, and the development of RNA-based vaccines.
Moreover, examining processivity provides insight into the molecular mechanisms that enable T7 RNA polymerase to maintain engagement with its DNA template. Increased processivity correlates with higher yield and speed in RNA synthesis, making T7 RNA polymerase a preferred choice among scientists.
In the sections that follow, we will dissect the features and factors contributing to the processivity of T7 RNA polymerase, illustrating its molecular structure, the significance of elongation in transcription, and the implications it holds for future research and industry innovations.
Overview of T7 RNA Polymerase
T7 RNA polymerase is an enzyme that primarily transcribes DNA into RNA, specifically employing the T7 promoter as a binding site. This enzyme is characterized by its ability to recognize specific promoter sequences, forming a stable initiation complex before embarking on the elongation phase. T7 RNA polymerase is known for its rapid transcriptional speed and is often cited as one of the most efficient RNA polymerases known.
Here are some key features of T7 RNA polymerase:
- Single Subunit Composition: Unlike multi-subunit RNA polymerases found in eukaryotes, T7 RNA polymerase is a single polypeptide chain, which simplifies the understanding of its catalytic mechanisms.
- High Specificity: The enzyme is selective in its interaction with the DNA template, ensuring that it transcribes the intended genes accurately.
- Fidelity and Efficiency: T7 RNA polymerase showcases remarkable fidelity, ensuring minimal errors during transcription, which is of utmost importance in producing functional RNA.
This overview lays the groundwork for understanding how T7 RNA polymerase achieves its unique properties, particularly its processivity during transcription, which will be further explored in the next subsection.
Importance of Processivity in Transcription
The concept of processivity in RNA polymerase is key to its function. In simple terms, processivity refers to the enzyme's ability to add nucleotides to the growing RNA strand without detaching from the DNA template. This is crucial, as higher processivity leads to increased RNA output in a shorter amount of time.
High processivity in T7 RNA polymerase translates to several important benefits:
- Amplification of RNA Products: With the ability to polymerize long RNA strands without frequent pausing or resetting, T7 RNA polymerase facilitates the efficient production of extensive transcripts required for various applications, such as research and diagnostics.
- Reduced Off-target Effects: When the enzyme remains associated with its template, it minimizes the chances of mis-firing or engaging in non-specific transcription, ultimately enhancing the accuracy of RNA synthesis.
- Consistency in Research: For researchers relying on synthesized RNA, consistent results and high yields are vital. Processive transcription ensures that experiments yield reliable and reproducible outcomes.
Molecular Structure of T7 RNA Polymerase
Understanding the molecular structure of T7 RNA polymerase is key to grasping how this enzyme achieves such remarkable levels of processivity during the transcription process. The architecture of the enzyme lays the groundwork for its function, influencing both the speed and accuracy of RNA synthesis. By focusing on specific components such as protein composition, active site configuration, and the role of metal ions, one can appreciate the intricacies that equate to T7 RNA polymerase’s efficiency.
Protein Composition
The protein composition of T7 RNA polymerase consists of multiple domains that serve distinct roles in the transcription cycle. The enzyme is primarily made up of a single polypeptide chain, which is about 97 kDa long. It incorporates regions that can be grouped into the following categories:
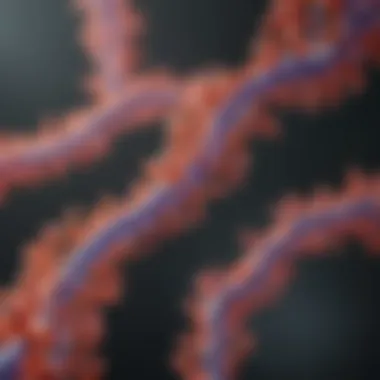
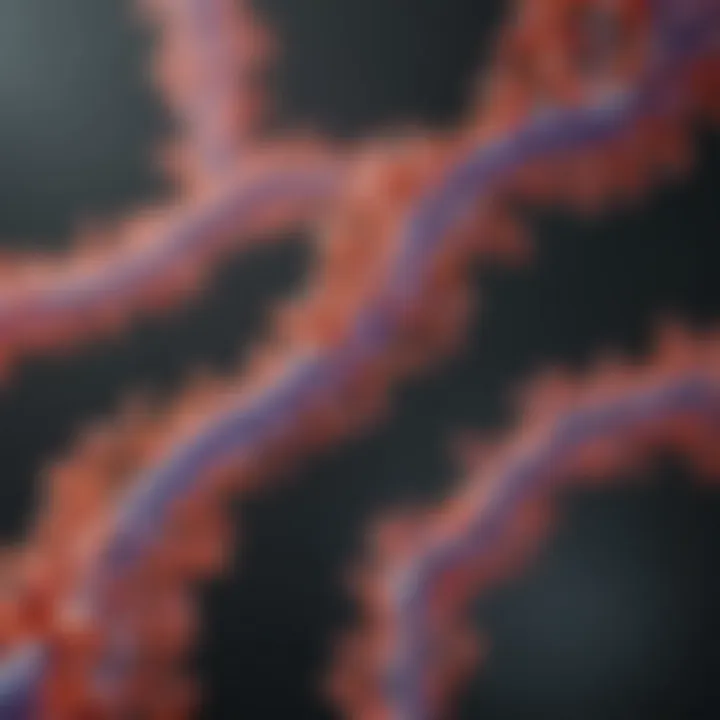
- Core Enzyme: This section possesses the basic units needed for the transcription of RNA, featuring a highly conserved structure.
- Promoter-Binding Domain: Known for its ability to recognize specific DNA sequences, this domain critically contributes to the initiation phase of transcription.
- Catalytic Domain: It directly facilitates the formation of phosphodiester bonds as nucleotides are added to the growing RNA strand.
Differential interactions among these domains enable T7 RNA polymerase to swiftly engage with its DNA template and transition from initiation to elongation. This versatility is essential for maintaining high levels of processivity across many transcription cycles.
Active Site Configuration
At the heart of T7 RNA polymerase’s function is its active site, a unique landscape shaped for RNA synthesis. The active site configuration is characterized by a cleft that accommodates the DNA-RNA hybrid structure. Within this region, key residues play a pivotal role in the catalysis of nucleotide addition. The landscape of the active site comprises:
- Nucleotide-Binding Sites: These sites ensure that incoming nucleotides are correctly matched with the corresponding template base, prompting accurate RNA synthesis.
- Catalytic Residues: Specific amino acids within the active site are responsible for facilitating the formation of the covalent bond between nucleotides, laying the groundwork for processivity.
- Metal Ion Coordination: The presence of divalent metal ions, typically magnesium or manganese, is of utmost importance as they help stabilize the developing RNA strand and are critical for the catalytic mechanism.
Through this intricate active site configuration, T7 RNA polymerase is engineered to efficiently extend RNA sequences with minimal errors, underscoring its reputation as a high-fidelity enzyme.
Role of Metal Ions in Activity
Metal ions, particularly magnesium ions, play an indispensable role in the functionality of T7 RNA polymerase. They serve several essential purposes:
- Catalytic Function: Metal ions participate directly in the catalytic process by coordinating with the phosphate groups of nucleotides, thereby reducing the activation energy needed for bond formation.
- Structural Stability: They contribute to maintaining the structural integrity of the active site, ensuring that the enzymatic function is not compromised.
- Regulatory Mechanisms: The concentration and availability of these ions can influence the activity of T7 RNA polymerase, adjusting the rate of transcription according to the cellular environment.
Their involvement in both the catalytic and structural aspects emphasizes the significance of metal ions in sustaining the dynamic activity of T7 RNA polymerase.
"The careful balance of molecular composition and ionic influence makes T7 RNA polymerase a standout in the realm of biocatalysis, allowing researchers to leverage its traits for biotechnological applications."
Mechanism of Transcription Initiation
Understanding the mechanism of transcription initiation is fundamental when studying T7 RNA polymerase, primarily because it sets the stage for transcription to occur effectively. This enzyme initiates the synthesis of RNA by recognizing specific sequences on the DNA, making it a crucial player in the transcription machinery. The intricate series of steps involved in this initiation phase lay the groundwork for the subsequent elongation of RNA, ensuring that the process is not just efficient but also precise.
Promoter Recognition
Promoter recognition is the very first step in transcription initiation. T7 RNA polymerase pinpoints DNA sequences known as promoters which are typically located upstream of the genes. These promoter regions contain distinctive motifs that uniquely identify them, allowing the polymerase to bind with high specificity.
- Key Features of Promoters:
- Consensus Sequences: These are short, conserved sequences that are critical for binding affinity.
- Positioning: The promoters must be exactly positioned relative to the gene to ensure accurate transcription initiation.
T7's ability to recognize its specific promoters is crucial. Unlike other polymerases which may require additional factors, T7 polymerase adeptly binds to its promoters autonomously. This is noteworthy, as it simplifies the transcription process and allows for rapid responses to cellular needs. The efficiency of transcription initiation hinges largely on this recognition step, underscoring its importance in the overall transcription mechanism.
Formation of the Open Complex
Once T7 RNA polymerase binds to the promoter, the next step is the formation of the open complex. In this stage, the enzyme undergoes conformational changes that enable it to unwind the DNA around the promoter region. This unwinding is critical as it exposes the DNA template strand necessary for RNA synthesis.
- Characteristics of Open Complex Formation:
- Local DNA Unwinding: The double helix of DNA unwinds at the promoter, creating a bubble-like structure.
- Binding of the Template Strand: The single-stranded template becomes accessible to the enzyme, ensuring that all subsequent actions can occur smoothly.
The formation of the open complex is a turning point in transcription initiation. If this process is efficient, T7 polymerase can begin synthesizing RNA swiftly, which is vital during high-demand moments for the cell.
First Nucleotide Incorporation
Following the establishment of the open complex, T7 RNA polymerase initiates the synthesis of RNA by incorporating the first nucleotide. This step is particularly significant as it sets the tempo for the entire transcription process. The correct integration of the first nucleotide is essential to establish a solid foundation for elongation.
- Mechanics of Nucleotide Incorporation:
- Nucleotide Selection: The enzyme selects the complementary ribonucleotide based on the template DNA strand.
- Catalytic Mechanism: A highly efficient catalytic mechanism guides the formation of a phosphodiester bond, linking the first two nucleotides together to initiate the RNA chain.
The efficiency of this initial incorporation can influence the overall transcription rate. A slow start could mean a lag in RNA synthesis, which is not ideal when rapid protein production is necessary.
Overall, the mechanism of transcription initiation in T7 RNA polymerase exhibits a beautifully orchestrated series of events that are integral to its high processivity and efficiency. Understanding these details not only enriches our comprehension of this particular polymerase but also opens doors to broader applications in biotechnology, where precision is paramount.
The process of transcription initiation is vital for the regulation of gene expression and has implications in various fields of molecular biology and therapeutic development.
Transcription Elongation and Processivity
In the realm of molecular biology, the transcription elongation phase plays a decisive role in mRNA synthesis, particularly when concerning T7 RNA polymerase. Understanding this phase is crucial for grasping how T7 efficiently creates RNA strands. Processivity, the ability of an enzyme to continuously synthesize nucleic acids without dissociating from the DNA template, stands as a pillar of transcription elongation. Essentially, if the polymerase can maintain its grip on the template, it becomes a powerhouse of RNA production, leading to enhanced efficiency and fidelity.
The advent of T7 RNA polymerase, known for its remarkable processivity, changes the game in various applications. It’s capable of rapid transcription, often reaching synthesis rates of up to 2000 nucleotides per second under optimal conditions. This exceptional performance is not just academic; it has practical implications in fields like gene therapy, where a robust RNA synthesis apparatus can significantly boost efficacy.
Nature of Processivity in T7 RNA Polymerase
T7 RNA polymerase exhibits an intrinsic ability to remain bound to the DNA template during transcription elongation, a phenomenon that enhances its processivity. The main influence for this stickiness lies in its molecular architecture. Notably, T7 RNA polymerase has a long and flexible linker region connecting its two domains, which helps it adapt to various structural changes as it encounters obstacles on the DNA template.
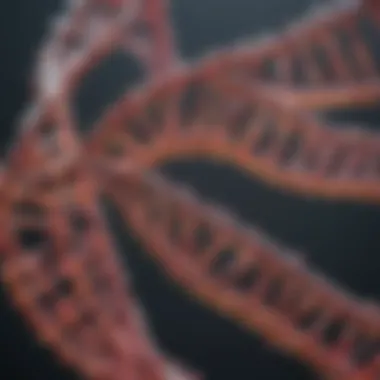
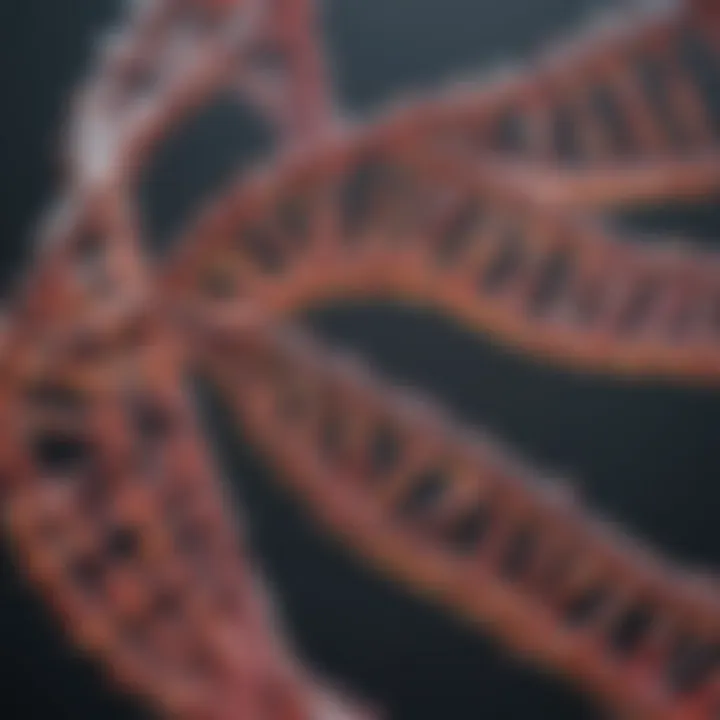
Moreover, its catalytic core is optimized for rapid nucleotide incorporation. The enzyme efficiently transmits conformational changes needed for incorporating nucleotides, meaning it can keep the transcript flowing smoothly. Essentially, this robust design allows T7 to engage in transcription with remarkable speed and accuracy, often considered as a well-oiled machine.
Factors That Enhance Processivity
Several factors come into play that can elevate the processivity of T7 RNA polymerase:
- Nucleotide Concentration: Higher concentrations of nucleotides facilitate faster incorporation, thus maintaining the polymerase’s grip on the template.
- Presence of Accessory Proteins: Certain co-factors can bolster the enzyme's stability and processivity. For example, using T7-specific transcription factors may help keep it engaged with the DNA.
- Environmental Conditions: Optimal pH and temperature can significantly affect the enzyme's efficiency. T7 performs best at specific conditions, where these variables allow it to retain its processive nature.
Each of these factors acts as a cog in the wheel of transcription, ensuring that the polymerase remains productive.
Comparison with Other RNA Polymerases
When pitted against other RNA polymerases, T7 RNA polymerase stands out due to its exceptional processivity. For instance, Escherichia coli RNA polymerase, while crucial in many cellular processes, typically demonstrates lower processivity. This often results in more frequent disengagement from the template during transcription, leading to slower RNA synthesis.
Conversely, polymerases like phage SP6 have competitive processivity; however, they often fall short of T7’s speed and efficiency. The streamlined structure and evolved mechanisms of T7 RNA polymerase give it an edge, enabling unique applications in biotechnological tools, such as in vitro transcription systems.
The ability of T7 RNA polymerase to maintain high processivity during transcription elongation not only underscores its utility in research but also places it at the forefront of biotechnological advancements.
In sum, the intricacies of transcription elongation and processivity are pivotal, especially when examining T7 RNA polymerase’s contributions in molecular biology. Understanding these elements can propel insights into how we harness these enzymes for innovative applications.
Termination of Transcription
The termination of transcription is a crucial phase in the life of an RNA molecule, particularly when we discuss T7 RNA polymerase. This enzyme, renowned for its high processivity and efficiency, does not terminate transcription haphazardly. Rather, it engages in a well-orchestrated closing act of mRNA synthesis, ensuring that the transcribed RNA is appropriate for its subsequent roles in the cell. The significance of this process, therefore, extends beyond mere completion; it shapes the quality and functionality of the RNA produced.
This part of the transcription cycle highlights key elements such as the mechanisms by which transcription ceases and the vital sequences embedded within the DNA that guide this conclusion. Understanding the termination phase sheds light on how errors might be minimized and fidelity maintained, which is paramount in molecular biology applications. It opens discussions on therapeutic potentials, influences on biotechnology, and molecular research—all realms where the implications of accurate RNA synthesis can’t be understated.
Mechanisms of Termination
The termination of transcription by T7 RNA polymerase generally involves two primary mechanisms: intrinsic and factor-dependent termination. Intrinsic termination relies on specific sequences within the RNA product itself, creating a hairpin structure followed by a stretch of uracil residues. This configuration exerts physical tension on the RNA-DNA complex, leading to a disengagement of the RNA from the DNA template, ultimately ceasing transcription.
On the other hand, factor-dependent termination necessitates the involvement of additional proteins, such as rho factor. This protein interacts with the RNA transcript and facilitates the release of the RNA molecule from the polymerase. While T7 predominantly utilizes intrinsic mechanisms, understanding factor-dependent pathways can provide insights into comparative transcription dynamics across various organisms and polymerases.
- Intrinsic Termination
- Factor-Dependent Termination
- Involves sequence-directed folding of RNA
- Creates a hairpin loop followed by uracil, causing disengagement
- Requires rho factor or other termination proteins
- Engages in active disengagement of RNA from the enzyme
Therefore, the precision in these termination mechanisms is essential in preserving the integrity of the genetic information. The efficiency with which T7 RNA polymerase executes this step ultimately dictates the success of downstream applications.
Role of Terminator Sequences
The terminator sequences embedded within the DNA play a pivotal role in guiding T7 RNA polymerase toward a successful transcriptional termination. These sequences are not just mere stopping points; they are intricate instructions that dictate how and when to halt transcription. Typically, they contain specific motifs recognized by the RNA polymerase, which trigger the necessary conformational changes leading to termination.
For instance, a common feature among terminator sequences is the presence of a GC-rich region that promotes the formation of a stable hairpin in the RNA. This structure is crucial as it acts as a signal for the polymerase to cease RNA synthesis. In the absence of these sequences, the polymerase might continue surfing along the DNA, potentially transcribing non-coding regions or even overlapping genes, which could jeopardize the organism’s genetic integrity.
Moreover, terminator sequences can have profound implications in biotechnological applications. For example, when designing synthetic constructs for gene expression, understanding and utilizing proper terminator sequences enhances the reliability of RNA production, making it critical for therapeutic developments.
The correct identification and utilization of terminator sequences is not just critical for terminating transcription but for ensuring the fidelity and functionality of the RNA transcribed from the DNA template.
In summary, the termination of transcription represented by T7 RNA polymerase does not occur in isolation but involves complex interactions and sequences that together play significant roles. From maintaining high fidelity to enabling innovative applications in research and therapy, understanding these elements will only bolster the ongoing exploration of RNA polymerases and their capacities.
Experimental Approaches to Study Processivity
The intricate nature of T7 RNA polymerase's processivity requires a careful examination through various experimental approaches. Understanding how this enzyme maintains its continuous RNA synthesis helps researchers unravel the molecular mechanisms that govern transcription. This section aims to highlight the significance of these approaches, emphasizing their benefits and the specific elements that contribute to a comprehensive analysis of processivity. By employing rigorous methodologies, scientists glean invaluable insights into the efficiency with which T7 RNA polymerase carries out transcription, paving the way for advancements in the field.
In Vitro Transcription Assays
In vitro transcription assays are crucial for studying the processivity of T7 RNA polymerase. These controlled laboratory experiments provide a means to manipulate conditions, allowing researchers to focus on the enzyme's activity without the complications often found in living organisms. Here are some noteworthy points regarding in vitro assays:
- Controlled Environment: These assays offer a stable setting, where variables such as temperature, ionic strength, and substrate availability can be finely tuned. This control helps in understanding how each factor influences the polymerase's processivity.
- Quantitative Measurements: Researchers can measure RNA synthesis rates accurately by analyzing the produced RNA products. This quantitative aspect is essential for comparing different experimental conditions.
- Diverse Applications: Assays can be modified to examine various conditions, from the presence of accessory proteins to the effect of different template DNA sequences.
In summary, in vitro transcription assays provide a detailed look into T7 RNA polymerase behavior, shedding light on the intricate aspects of its processivity during RNA synthesis.
Single-Molecule Techniques
Single-molecule techniques represent a significant leap forward in our understanding of T7 RNA polymerase. Unlike traditional bulk analysis, which averages the behavior of millions of molecules, these methods allow direct observation of individual enzymes at work. This level of detail can yield unique insights not visible through conventional approaches. Here are a few pivotal elements of single-molecule techniques:
- Real-Time Monitoring: Researchers can track the action of a single T7 RNA polymerase throughout the transcription process, observing how it interacts with the DNA template and RNA product in real-time.
- Heterogeneity Analysis: These techniques enable the exploration of variability between individual molecules. For instance, differences in processivity among single enzymes can highlight what influences the overall transcription efficiency.
- Advanced Imaging: Utilizing state-of-the-art microscopy, scientists can visualize complex transient states of the enzyme, offering a window into its catalytic cycle.
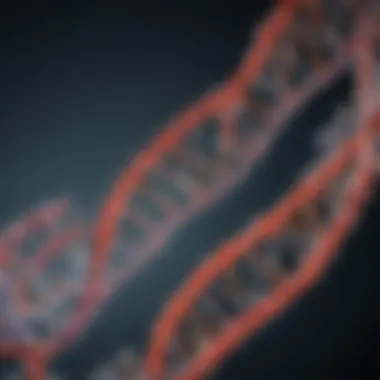
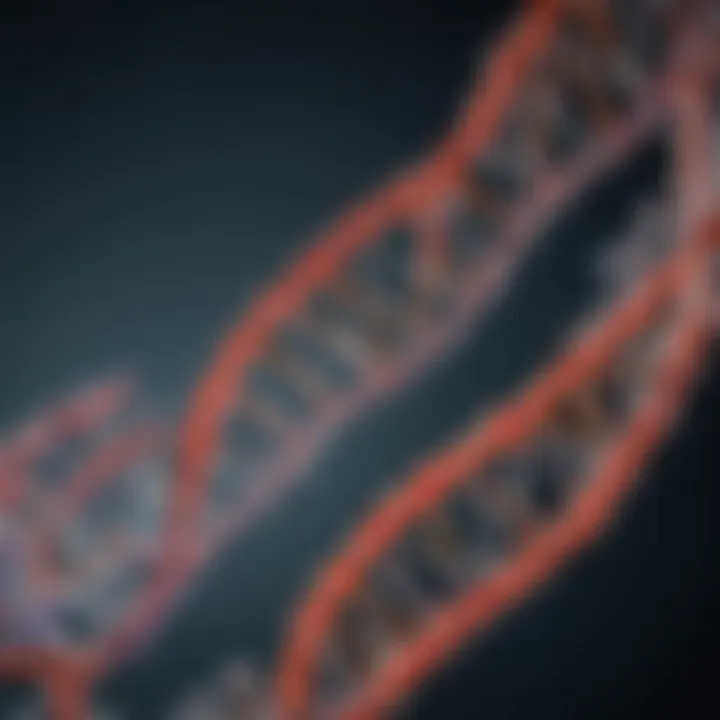
"The integration of in vitro assays and single-molecule approaches showcases the innovative strategies that are crucial for dissecting the nuances of enzyme activity."
These methods not only unravel the complexities surrounding T7 RNA polymerase but also set the stage for future developments in synthetic biology and biotechnology.
Applications in Biotechnology
Understanding the unique traits of T7 RNA polymerase opens the door to a myriad of applications in the field of biotechnology. This enzyme is particularly prized for its remarkable capabilities in synthesizing RNA, which has far-reaching implications across various domains such as genomics, vaccine development, and therapeutic interventions. Here, we will explore the multiple facets of T7 RNA polymerase applications and the unique benefits they confer.
Use in RNA Synthesis
T7 RNA polymerase serves as a reliable workhorse in RNA synthesis. Every time researchers need a high yield of specific RNA sequences, T7 polymerase is likely their go-to option. Its ability to transcribe DNA templates rapidly and accurately allows for the production of large quantities of RNA, simplifying the creation of functional ribonucleic acid required in several experimental setups.
Key aspects of T7 RNA polymerase in RNA synthesis include:
- Speed and Specificity: The enzyme's natural proclivity for recognizing its promoters leads to efficient transcription, ensuring that the desired RNA template can be produced in record time.
- High Fidelity: The enzyme is renowned for maintaining the accuracy of transcription, which is crucial for experimental consistency and reliability.
- Versatility: T7 RNA polymerase can work with a variety of substrates, including plasmids and linear DNA, enabling complex applications across different forms of biotechnology.
These attributes make T7 RNA polymerase a staple in laboratories focused on molecular biology.
Potential in Therapeutic Development
The therapeutic potential of T7 RNA polymerase stretches beyond simple RNA synthesis. As we move toward more personalized medical solutions, understanding how to manipulate gene expression becomes increasingly vital. T7 polymerase can create RNA molecules that serve as vital components in different therapeutic frameworks:
"T7 RNA polymerase is a cornerstone for innovative therapies, bridging the gap between basic research and clinical application."
Consider some primary areas where T7 RNA polymerase shines in therapeutic contexts:
- Vaccines: With the ongoing battles against pathogens, RNA-based vaccines have gained prominence. The capacity to produce mRNA vaccines using T7 RNA polymerase significantly expedites their development and rollout.
- Gene Therapy: Targeted gene therapy approaches often require the incorporation of specific RNA sequences. T7 polymerase provides a reliable method for generating mRNA that can then be translated into therapeutic proteins to treat genetic disorders.
- RNA Interference (RNAi): Researchers leverage T7 polymerase to synthesize small interfering RNAs (siRNAs), enabling the silencing of specific genes, which is fundamental in understanding gene function and developing therapies for diseases like cancer.
In summary, the applications of T7 RNA polymerase in biotechnology are broad and transformative. By facilitating RNA synthesis and offering pathways into novel therapeutic developments, T7 RNA polymerase plays an invaluable role, driving innovations that may one day revolutionize healthcare.
Challenges and Limitations
Understanding the challenges and limitations related to T7 RNA polymerase is crucial in evaluating its overall utility in both research and industrial applications. As with any biotechnological tool, there are hurdles that must be navigated to maximize the enzyme's effectiveness. Recognizing these difficulties not only helps in refining methodologies but also in enhancing the final outcomes in various applications, particularly those concerning RNA synthesis.
Stability Challenges in Vivo
When it comes to utilizing T7 RNA polymerase in living cells, stability in vivo emerges as a significant concern. Unlike controlled laboratory environments, cellular conditions can disrupt the enzyme's functionality. One of the primary issues lies in the enzyme's susceptibility to degradation by cellular proteases. Once inside a host organism, T7 RNA polymerase may face a cocktail of degrading enzymes. As such, managing the stability of this enzyme in a biological context remains a formidable challenge.
Moreover, the natural inhibitors present in cells can affect T7 RNA polymerase activity. These inhibitors can bind to the enzyme, impeding its ability to efficiently transcribe RNA, thereby leading to reduced yields of the desired product. In contrast, the enzyme shows remarkable efficiency in vitro, but scaling the process to a living system often leads to complexities that cannot be overlooked.
Researchers must explore strategies to engineer T7 RNA polymerase or the host environment itself to mitigate these stability issues. Techniques such as encapsulation or using specific plasmid systems may provide potential solutions, yet these methods can introduce their own set of complications. The balance between the enzyme's activity and stability is a tightrope walk that continues to challenge many in the field.
Synthetic Limitations in RNA Production
Another dimension that cannot be ignored is the synthetic limitations faced while producing RNA using T7 RNA polymerase. Even though this enzyme is known for its high processivity, producing long strands of RNA presents unique difficulties. One limitation arises from the tendency of T7 RNA polymerase to stall during the transcription of long templates. This can lead to incomplete transcripts, ultimately affecting the yield and integrity of the RNA product.
Furthermore, there are restrictions related to template length. While the enzyme excels at transcribing short to medium-length RNA, the production of transcripts longer than a few thousand nucleotides becomes erratic. This limitation has implications for numerous applications, especially those requiring lengthy RNA sequences for therapeutic or research purposes.
Additionally, the production scale can also pose logistical issues. Achieving high yields typically requires fine-tuning reaction conditions and optimizing various parameters, such as temperature and Mg²⁺ concentration. These intricacies can turn a seemingly straightforward task into a daunting challenge, ultimately impacting the overall workflow.
In summary, while T7 RNA polymerase presents substantial advantages for RNA synthesis, these challenges require thoughtful consideration and innovative approaches to harness its full potential in various applications.
Future Perspectives
The horizon for T7 RNA polymerase and its processivity encapsulates a multitude of exciting prospects. As the field of molecular biology continues to evolve, understanding the nuances of T7 RNA polymerase’s function can lead to significant advancements in both research and practical applications. This exploration of future trends emphasizes not only the scientific potential but also the broad implications that innovations in this area hold.
Innovations in Enzyme Engineering
With the ever-growing need for tailored solutions in biotechnology, innovations in enzyme engineering present a game-changing opportunity. For instance, researchers can now modify T7 RNA polymerase at a molecular level to enhance its properties, allowing for greater yields in RNA production. Such modifications may include altering the active site to improve substrate binding or to enhance the enzyme's resistance to inhibitors.
In particular, scientists have been experimenting with directed evolution techniques that promote desirable traits in enzymes. This fascinating approach enables the selection of variants that exhibit improved processivity and accuracy in transcription. As a result, engineered T7 RNA polymerases could be used in specialized applications, such as high-throughput RNA synthesis or the production of complex RNA structures for therapeutics.
"The future of enzyme engineering is bright, with every tweak and toss of a genetic coin, we inch closer to the ultimate efficiency in RNA synthesis."
Implications for Research and Industry
Advancements in T7 RNA polymerase engineering are not just academic pursuits; they carry profound significance for various sectors. In research, optimized polymerases will provide researchers with tools that amplify their ability to explore genetic functions, gene expression, and RNA-related phenomena. Moreover, they can revolutionize synthetic biology endeavors, allowing for the construction of sophisticated genetic circuits.
Industrially, the implications are equally remarkable. Superior T7 RNA polymerases can streamline processes in vaccine development, particularly in RNA vaccines that gained attention during the recent global health crises. Their reliability and efficiency could also pave the way for advancements in gene therapy, where precise RNA synthesis is paramount.
Additionally, by integrating computational biology with enzyme design, researchers can predict how modifications will impact enzyme performance, thus accelerating the development process.
Finale
In essence, the future perspectives for T7 RNA polymerase embody an exciting blend of scientific curiosity and practical application. The continuous evolution of enzyme engineering will undoubtedly shape the landscape of molecular biology and biotechnology for years to come. For students, researchers, and industry professionals, staying attuned to these developments may hold the key to unlocking new levels of efficiency and innovation in RNA synthesis.