Understanding Neurons: Core Components and Functions
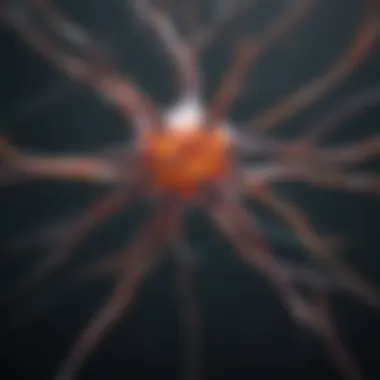
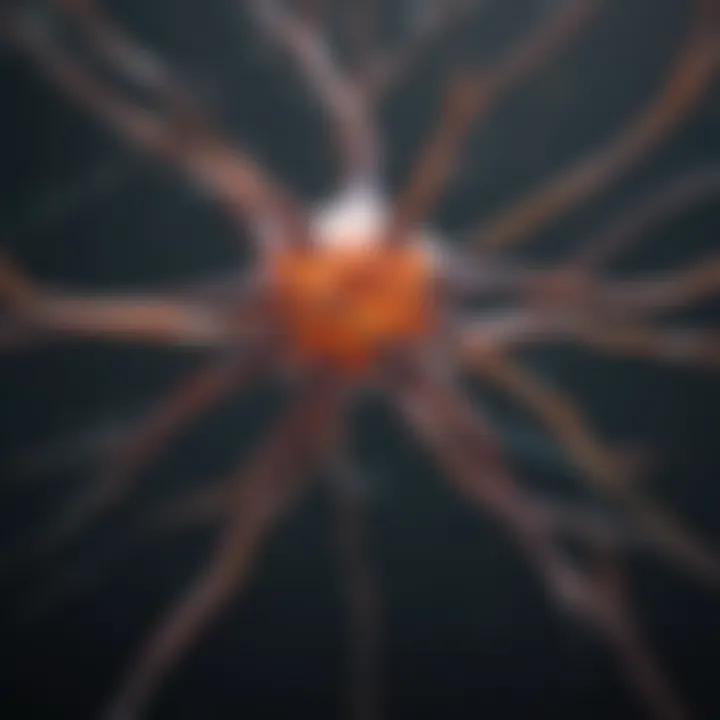
Overview of Research Topic
Brief Background and Context
Neurons form the bedrock of the nervous system, acting as the primary cells responsible for the transmission of signals across the body. Their discovery dates back to the late 19th century, when Santiago Ramón y Cajal pioneered the study of these intricate cells. Since then, neurons have captivated scientists, giving rise to an expansive field of neuroscience. These cells are not merely passive conduits for information; they actively participate in the processing and integration of sensory input, enabling organisms to react appropriately to their environment.
Importance in Current Scientific Landscape
In recent years, the significance of neurons has only intensified, especially with the advent of advanced imaging techniques and molecular biology tools. These advances have unlocked doors to understanding complex neural networks and their roles in cognition, emotion, and behavior. As modern science seeks to demystify conditions like Alzheimer’s, schizophrenia, and autism, neurons remain at the forefront of this exploration.
"Neurons are not just the messengers of the brain; they are the storytellers of our very existence."
Core Components of Neurons
Neurons possess unique structures that contribute to their functionalities, which can be broken down into several core components:
- Cell Body (Soma): This is the neuron's headquarters. It contains the nucleus and is essential for maintaining the cell's health and functionality.
- Dendrites: Branching out from the cell body, dendrites are designed to receive signals from other neurons, much like how antennas catch radio waves.
- Axon: The long, slender projection that transmits electrical impulses away from the soma to other neurons or muscles. Axons can vary significantly in length, influencing signal transmission speed.
- Myelin Sheath: A fatty layer encasing the axon, providing insulation that enhances the speed of electrical impulses through a process known as saltatory conduction.
- Synapses: The junctions where neurons communicate with each other. Through synapses, neurotransmitters are released and absorbed, ensuring that signals are conveyed effectively.
Functions of Neurons
Neurons perform a multitude of functions within the body:
- Signal Transmission: Neurons transmit signals at remarkable speeds, often faster than one might realize, creating a seamless connection between different parts of the body.
- Information Processing: Beyond mere signaling, neurons process information and participate in higher cognitive functions, such as reasoning and memory recall.
- Response Generation: Neurons play a key role in generating responses to stimuli, allowing organisms to react to their environment.
Current Trends in Neuroscience Research
Recent developments in neuroscience have heightened understanding of the neuroplasticity of neurons—their ability to adapt and reorganize. This aspect is crucial in rehabilitation practices and recovery from brain injuries. Today's researchers are also delving into the genetic and molecular bases for various neurological disorders, aiming to put an end to age-old mysteries.
In summary, neurons are not just cells; they are intricate components that underpin not only individual health but also the broader understanding of various neurological phenomena. Their continual study is paramount in shaping the future of medical science and cognitive studies.
Intro to Neurons
The world of neurons encapsulates a realm where tiny cells perform monumental tasks within the nervous system. Understanding neurons is essential because they serve as the foundation for all neurological functions. These are not mere cells; they are the architects of thoughts, movements, and even our emotions. Without them, the intricate web of communication within our bodies would unravel, leaving us unable to react, feel, or even think.
A closer look at neurons reveals their complex structure and diverse functions, illuminating why they are regarded as the cornerstone of neuroscience. The functionality of the nervous system hinges on these cells delivering messages across various pathways, making knowledge of their biology vital for students, researchers, and professionals alike. From aiding motor control to processing sensory input, neurons facilitate innumerable roles critical for survival and social interactions.
Definition and Importance
Neurons can be defined as specialized cells that transmit nerve impulses. They are distinct from other cell types due to their unique structure, comprising a cell body, dendrites, and an axon. The importance of neurons cannot be overstated; they form the communication backbone within the brain and throughout the body.
Neurons are crucial because:
- They execute the brain's commands, influencing everything from muscle movement to sensory perception.
- They are involved in learning and memory, making them key players in cognitive health.
- Disorders of neurons, such as in neurodegenerative diseases, shed light on the critical role these cells play in maintaining well-being.
Highlighting the definition of neurons helps lay the groundwork for deeper discussions about their structure and diverse types. By exploring neuronal characteristics, we also gain insights into how neurons interact with one another and facilitate the complex networks that influence our daily lives.
Historical Context in Neuroscience
The history of neuroscience is a tapestry woven with discoveries that have expanded our understanding of neurons. The field has seen its share of pioneers, such as Santiago Ramón y Cajal, who, through innovative staining techniques, provided the first comprehensive maps of neurons in the late 19th century. His work established the neuron doctrine, which postulated that neurons are distinct and functional units of the nervous system.
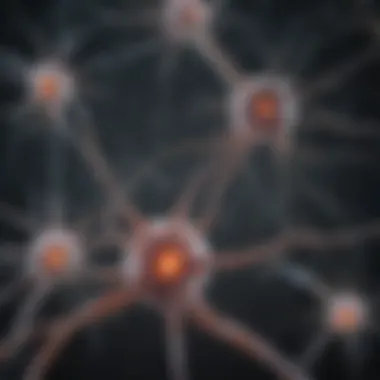
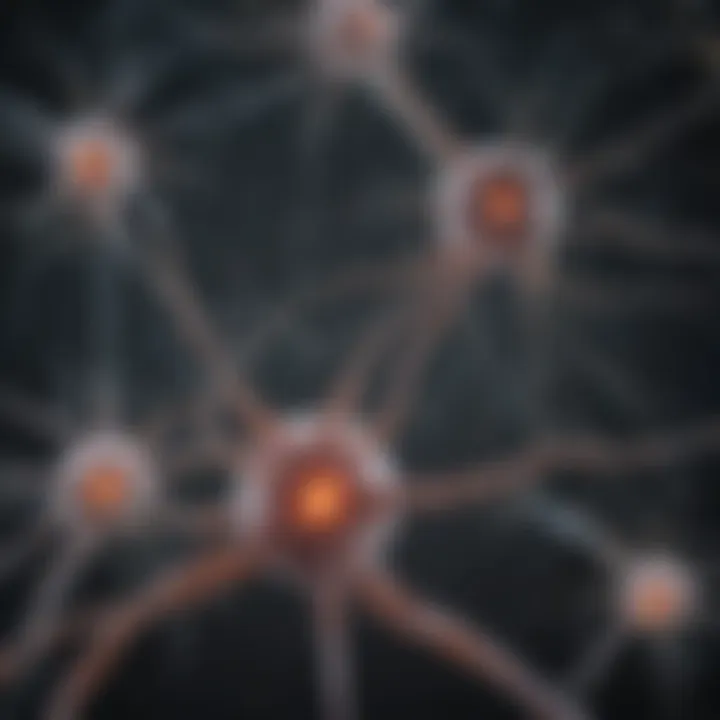
As time progressed, the advent of technology spurred further advancements in neuronal studies. The introduction of electron microscopy in the mid-20th century facilitated the observation of neurons at a microscopic level, enhancing our understanding of their intricate structures.
Key milestones in the history include:
- The development of the action potential concept, which laid the groundwork for understanding how neurons communicate.
- The discovery of neurotransmitters and their role in synaptic transmission, revealing the chemical nature of neuronal communication.
Through this lens, the historical context of neuroscience underscores the evolution of thought about neurons, emphasizing their complexity and marking significant strides towards the modern understanding we possess today. This exploration prepares readers for further discussions on the structural aspects of neurons, illustrating how understanding these core components is essential for grasping the larger picture in neuroscience.
Neuronal Structure
Neuronal structure serves as the foundation for understanding how neurons function in different contexts. Each component plays a critical role in facilitating communication within the nervous system and contributes to the overall health of the brain and body. Grasping these structural details helps highlight not just how neurons work, but also why they are essential to our everyday functioning, including perception, movement, and cognition.
Cell Body: The Soma
The cell body, also known as the soma, is often viewed as the control center of the neuron. It's where the nucleus resides, housing the genetic material that dictates the neuron's operations. This central location is crucial, as it processes signals received from dendrites and effectively manages the metabolic functions necessary for overall neuron health. By synthesizing proteins essential for neurotransmitter production, the soma ensures that the neuron remains functional.
An important aspect of the soma is its composition, which includes organelles such as ribosomes and mitochondria. These components aid not only in generating energy but also in supporting cellular repair and growth. The health of the soma can significantly influence the neuron's capability to propagate signals effectively.
Dendrites: Receiving Signals
Dendrites serve as the primary receptors of information for neurons. They branch out like the arms of a tree, forming connections with multiple other neurons. This extensive branching increases the surface area, allowing for a more significant amount of incoming signals. Each synapse that connects a dendrite to an adjacent neuron represents a potential path for signal transmission.
When an incoming signal arrives, dendrites detect it and convert it into electrical impulses that travel toward the soma. Their ability to receive numerous signals simultaneously supports complex functions, such as learning and memory. The structure of dendrites can even change over time, a phenomenon known as synaptic plasticity, which is linked to how we adapt and learn from experiences.
Axon: Conducting Impulses
The axon acts like a highway for the electrical impulses generated within the neuron. It extends from the soma and can vary significantly in length—some might be just a millimeter, while others in larger animals can span over a meter. The main role of the axon is to transmit signals away from the soma toward other neurons, muscles, or glands.
One critical feature of the axon is the presence of voltage-gated channels, which open and close in response to changes in electrical charge, allowing ions to flow in or out. This movement of ions generates what is known as an action potential—a rapid change in electrical charge that travels along the axon to its terminal. This action potential is essential for the communication between neurons and thus, the entire nervous system.
Myelin Sheath: Insulating Axons
The myelin sheath is a segmented layer of lipid-rich material that wraps around the axon of many neurons. Think of it like the rubber insulation on an electrical wire. This critical structure not only provides electrical insulation but also speeds up the transmission of impulses along the axon by promoting a process called saltatory conduction.
During saltatory conduction, the action potential jumps from one node of Ranvier (the gaps in the myelin sheath) to another, rather than traveling the entire length of the axon. This greatly increases the speed of signal transmission, allowing for rapid communication between neurons. The integrity of the myelin sheath is essential for proper neural function, and damage to this covering can lead to significant neurological diseases, such as multiple sclerosis.
"The structure of neurons is not just a matter of biology; it reflects the intricate details of how we process information and interact with the world around us."
Understanding these components—the soma, dendrites, axon, and myelin sheath—provides valuable insights into how neurons operate and communicate. This knowledge lays the groundwork for exploring the dynamic world of neuronal communication and how it contributes to overall neural health.
Types of Neurons
In understanding the array of functions within the nervous system, it’s essential to grasp the different types of neurons that contribute to our body’s complex connectivity. The classification of neurons—sensory, motor, and interneurons—addresses the unique roles each plays in processing information, executing actions, and maintaining communication between the brain and the rest of the body. This knowledge not only elucidates how the nervous system operates but also serves as a foundation for exploring neurological research and treatment options.
Sensory Neurons: Input from Environment
Sensory neurons, often referred to as afferent neurons, serve as the body’s sensory interface with the environment. These specialized cells are responsible for transmitting information from sensory receptors to the central nervous system (CNS). The fascinating part is how they respond to stimuli, whether it's light, sound, touch, or taste.
- Structure: Sensory neurons have distinct structures that allow them to receive specific types of sensory inputs. For instance, photoreceptors in the retina convert light into signals, while mechanoreceptors in the skin respond to touch and pressure.
- Examples: The neurons that help us perceive the warmth of the sun or the sound of music are sensory neurons. They play an indispensable role in our interaction with the world, enabling us to react to our surroundings.
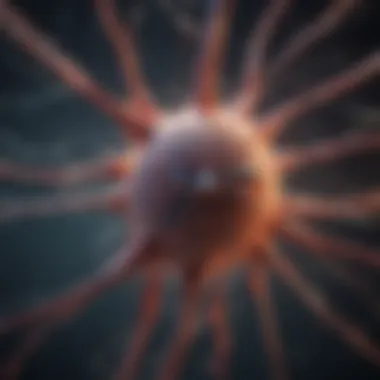
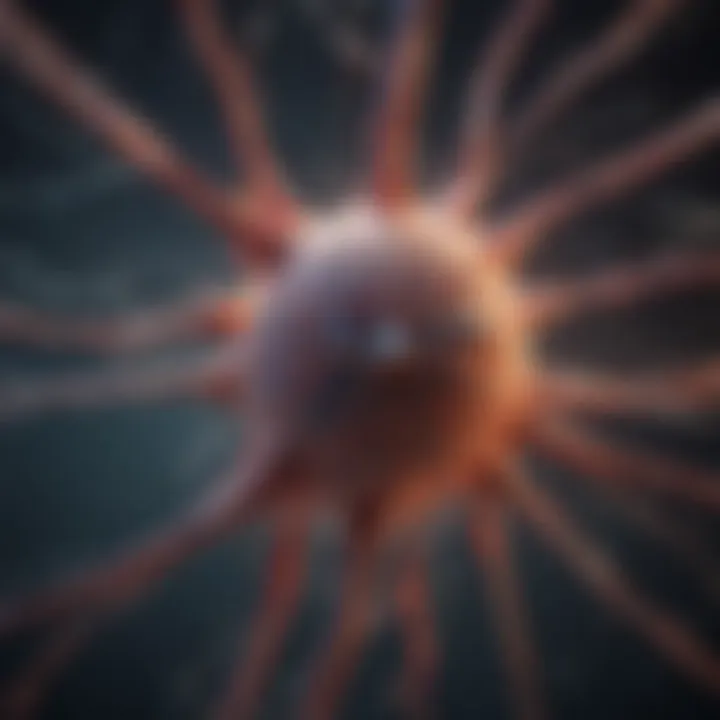
"Without sensory neurons, actions like stepping back from a hot surface or tasting food would be impossible, highlighting their critical function in survival and experience."
The significance of sensory neurons extends beyond simple reaction; they also contribute to our perceptions and overall experiences. Understanding these neurons lays the groundwork for grasping disorders like sensory processing disorders, which can affect how individuals interpret sensory information.
Motor Neurons: Commanding Movements
Motor neurons, or efferent neurons, are the heavy lifters of movement, translating signals from the CNS to muscles throughout the body. They provide the necessary commands for voluntary actions, like lifting an arm, and involuntary responses, such as reflexes.
- Motor Pathways: These neurons are often categorized into upper and lower motor neurons. Upper motor neurons originate in the brain and send messages down the spinal cord, while lower motor neurons connect from the spinal cord to the muscles.
- Role in Movement: Each motor neuron can innervate multiple muscle fibers, resulting in coordinated actions. For example, in playing an instrument, motor neurons facilitate the precise movements of fingers and hands, translating cognitive desires into physical action.
The interaction between motor neurons and muscle fibers is fundamental to our ability to move. An understanding of motor neurons opens pathways to explore conditions like amyotrophic lateral sclerosis (ALS) that directly affect muscle control, underscoring the importance of this type of neuron in both health and disease.
Interneurons: Connecting Neurons
Interneurons are the silent orchestrators of the nervous system, connecting sensory neurons to motor neurons. They reside exclusively within the CNS and play a crucial role in processing information, particularly in reflexes and complex behaviors.
- Facilitation of Communication: These neurons act as intermediaries, relaying signals from sensory neurons to motor neurons, thus allowing for rapid responses. For instance, when touching something hot, sensory neurons send signals to interneurons which then relay the message to motor neurons for an immediate withdrawal response.
- Types of Interneurons: They can be excitatory, promoting action, or inhibitory, tempering responses, which provides balance and coordination in neural communications.
Regardless of the situation, interneurons underpin critical processes like learning and memory by allowing for feedback loops and adaptations in signaling. Their role emphasizes the interconnected nature of neuronal types, playing a fundamental part in our cognitive functions.
Understanding these three types of neurons—sensory, motor, and interneurons—provides a window into the myriad functions of the nervous system, underlining their significance not only in daily life but also in various neurological conditions. This knowledge fosters further exploration into how these neurons can be targeted in research or treatment, impacting our understanding of both the functioning and malfunctions of the human brain.
Neuronal Communication
Neuronal communication is the thread that binds individual nerve cells into a cohesive network, allowing for the transmission and integration of information across the nervous system. This collaboration forms the backbone of our ability to respond to stimuli, interact with our environment, and engage in cognitive functions. Exploring how neurons communicate not only sheds light on the mechanics of our nervous system but also highlights why understanding this process is crucial for advancements in neuroscience and medicine.
Action Potentials: Signal Transmission
An action potential can be likened to a sudden shout in a crowded room. It's a rapid electrical impulse that travels along the axon of a neuron, enabling it to carry messages quickly and efficiently. When a neuron is sufficiently stimulated, it reaches a threshold that triggers this action potential. Think of it as a domino effect; once the first domino falls, it sets off a chain reaction down the line.
This process hinges on the movement of ions in and out of the neuron, particularly sodium (Na+) and potassium (K+) ions. When a neuron is at rest, it maintains a balance, but upon activation, sodium rushes in, temporarily reversing the charge inside the cell. This change travels along the axon, signaling neighboring neurons to act. Once the wave of depolarization passes, potassium ions exit the neuron to restore the resting state. In essence, action potentials are vital signals that dictate how information is relayed throughout the nervous system.
Synaptic Transmission: Signal Relay
The term synaptic transmission refers to the intricate process of transferring signals between neurons at specialized junctions called synapses. Imagine these synapses as the turnstiles at a concert; only so many people can pass through at once, and the flow is carefully managed. When an action potential reaches the end of an axon, it prompts the release of neurotransmitters, which are chemical messengers.
These neurotransmitters traverse the synaptic cleft and bind to receptors on the surface of the adjacent neuron, which can either provoke a new action potential or inhibit signal firing altogether. This dual capability allows for nuanced control over neuronal communication, ensuring that the nervous system is responsive yet stable. Factors such as neurotransmitter type, receptor affinity, and post-synaptic response influence how effectively information is transferred.
Neurotransmitters: Chemical Communicators
At the crux of neuronal communication are neurotransmitters, the chemical agents responsible for conveying messages across synapses. These molecules are like postmen in the neurosystem, dutifully delivering messages between neurons. Each neurotransmitter has a specific role, with some promoting excitation while others might inhibit signals. For instance, dopamine is associated with reward processing, while serotonin plays a crucial role in mood regulation.
The balance of these neurotransmitters is paramount. An excess of one type or a deficit of another can lead to a variety of neurological disorders. For instance, insufficient dopamine levels are linked to Parkinson's disease, showcasing how pivotal neurotransmitter activity is for maintaining mental and physical health. The ongoing research into neurotransmitter function and their pathways promises significant advancements in therapeutic interventions for numerous neurological conditions.
"The intricate dance of action potentials and neurotransmitters exemplifies the beauty of neuronal communication, where harmony is vital for maintaining the health of the nervous system."
In summary, understanding neuronal communication—through action potentials, synaptic transmissions, and the roles of neurotransmitters—provides invaluable insights into how our nervous system operates and regulates both simple and complex functions. Each component plays a distinct role, showcasing the intricate interplay necessary for maintaining cognitive and physical health.
Neurons in Health and Disease
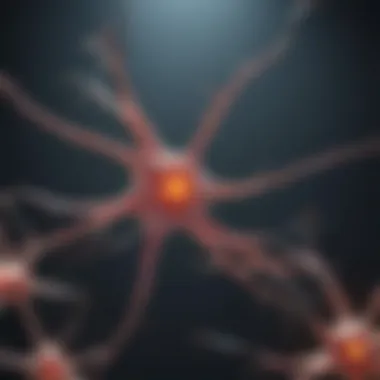
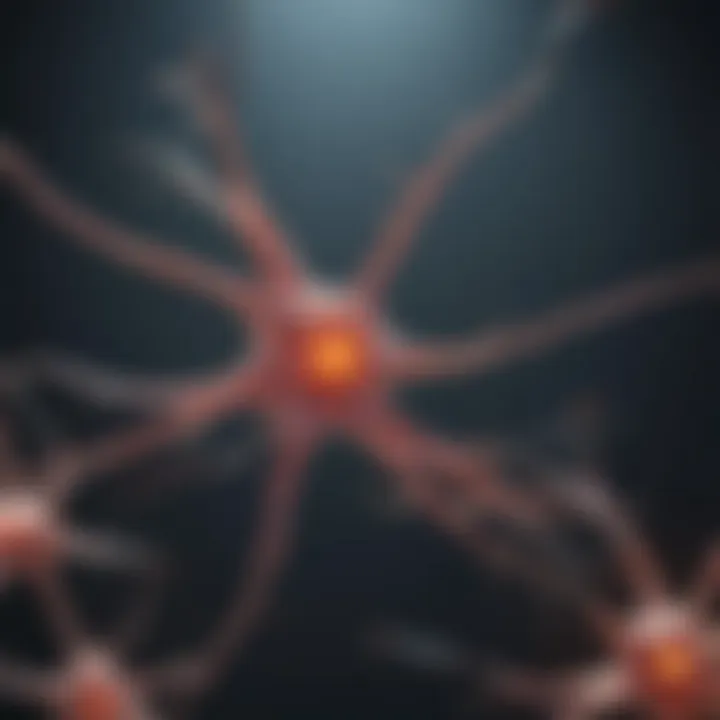
The study of neurons in the context of health and disease holds significant weight in understanding how the nervous system operates. These specialized cells not only facilitate communication within the body but also underscore various health conditions that can stem from neuronal dysfunction. By examining the relationship between neurons and health, we uncover insights into treatment possibilities and preventative measures for numerous neurological disorders. Moreover, understanding these connections is vital for researchers and healthcare professionals, offering a lens to assess therapeutic innovations and approaches on how to enhance patient outcomes.
Role in Neural Disorders
Neural disorders can affect people from all walks of life, leading to impairments that drastically alter one's quality of life. From anxiety and depression to conditions like schizophrenia or autism, these disorders often have roots in neuronal abnormalities. For instance, a disruption in neurotransmitter levels can throw off the delicate balance required for healthy brain function, resulting in mood swings or cognitive dysfunction.
"Neurons are the main players in brain activities, and their dysfunction often leads to serious mental health issues."
Consider bipolar disorder, which shows fluctuations in mood linked to erratic neuronal activity. In other cases, such as in epilepsy, bursts of unregulated neuron signals can lead to seizures, affecting millions globally. The study of these disorders illuminates how the intricate wiring of neurons can lead to vast networks of effects, and highlights the critical need for targeted therapies that can stabilize neuronal function and psychological health.
Impact of Neurodegenerative Diseases
Neurodegenerative diseases such as Alzheimer's, Parkinson's, and Huntington's are poignant reminders of how vulnerable our neurons can be. These conditions typically involve the gradual degeneration and death of neurons, leading to severe cognitive and motor deficits. When looking at Alzheimer’s, for instance, the accumulation of amyloid plaques is believed to disrupt neuronal communication, leading to memory loss and cognitive decline. This connection helps researchers identify potential therapeutic targets.
In the case of Parkinson's disease, the loss of dopamine-producing neurons leads to tremors and stiffness. Understanding these mechanisms not only sheds light on the diseases themselves but also emphasizes the importance of early detection and supportive therapies. For families and caregivers, comprehending how these diseases affect neuronal function can lead to more empathetic and effective caregiving approaches.
Neuroplasticity: Adaptability of Neurons
Neuroplasticity represents a fascinating landscape of opportunity within the field of neuroscience. It refers to the brain's ability to rewire itself based on experiences, injuries, or changes in the environment. This adaptability is critical for rehabilitation following brain injuries or strokes, as compensatory pathways can be formed in the wake of neuronal loss.
For instance, after a stroke, patients often work tirelessly in therapy to boost the function of remaining neurons, bridging gaps left by damaged areas. The remarkable aspect of neuroplasticity is that it is not confined to recovery; it supports learning and memory, known as synaptic plasticity, which plays a pivotal role in how new skills or knowledge are acquired. Engaging in consistent mental activities or physical exercises can bolster the brain's adaptability, highlighting the importance of maintaining both mental and physical health as we age.
Recent Advances in Neuronal Research
The field of neuroscience is rapidly evolving, and the recent advances in neuronal research are nothing short of fascinating. These developments greatly enhance our understanding of how neurons function, but they also open new avenues for treatment and prevention of neurological disorders. From cutting-edge imaging techniques to insights gleaned from neurogenetics, these advances hold tremendous potential for both basic science and clinical applications.
Technological Innovations in Study
Technological innovations have transformed how researchers study neurons. For instance, techniques like optogenetics allow scientists to manipulate the activity of specific neurons with remarkable precision using light. This method provides insight into the function of neurons in real-time, aiding in the understanding of complex behaviors and responses. Other innovations include high-resolution imaging techniques like two-photon microscopy, which enables the visualization of neuronal activity at microscopic scales.
Furthermore, advancements in electrophysiology, such as patch-clamp recording, enhance our ability to measure electrical properties of neurons with unprecedented accuracy. These tools help pinpoint how synaptic connections operate and how neural circuits communicate, shedding light on intricate processes that underlie cognitive functions.
Future Directions in Neuroscience
The future of neuroscience is promising, particularly with the integration of interdisciplinary approaches. For example, combining artificial intelligence with neuronal data could lead to breakthrough discoveries in how we approach brain disorders. Machine learning algorithms can analyze vast amounts of neuronal data, promoting a better understanding of brain function and dysfunction.
Moreover, as our understanding of neuroplasticity deepens, researchers are exploring avenues for therapies that boost the brain's innate ability to reorganize itself. Techniques such as brain-computer interfaces are on the rise, offering ways for individuals with neurological impairments to regain lost functionality.
"The advancements in neuronal research not only amplify our comprehension of the nervous system but also pave the way for innovative therapeutic strategies that can transform patient care."
Ending
The study of neurons serves as a cornerstone for understanding not only the intricacies of the nervous system but also the broader themes of physiology and behavior. In this article, various aspects of neurons were dissected, revealing their significance within the biological framework. As we journeyed through its structure, types, and functions, we observed how these cells form the backbone of neural communication and cognitive processes.
Summary of Key Points
- Neuronal Structure: Delved into components like the soma, dendrites, axon, and myelin sheath, emphasizing how they contribute to the functionality of neurons.
- Types of Neurons: Discussed sensory, motor, and interneurons, elaborating on their specific roles in processing and relaying information.
- Neuronal Communication: Explored the processes of action potentials and synaptic transmission, highlighting neurotransmitters as the key players in communication between neurons.
- Health Implications: Addressed the role of neurons in both neural disorders and neurodegenerative diseases, pointing out the urgent need for continued research in these areas.
- Recent Advances: Summarized how technological innovations shape the future of neuroscience, paving the way for groundbreaking discoveries that could redefine our understanding of neuron functionality.
As we review these core elements, it's clear that neurons are not just passive conduits of information but rather active contributors to the entire spectrum of human experience.
Implications for Future Research
The future landscape of neuroscience hinges on our evolving comprehension of neurons. Research in this sphere is not only vital for unraveling existing medical enigmas but also for advancing therapeutic avenues for neurological conditions. Some key implications include:
- Innovative Treatments: Advances in understanding neuroplasticity can lead to novel approaches in rehabilitation for stroke victims or those with traumatic brain injuries.
- Neurodegenerative Disease Management: Gaining insights into the cellular mechanisms underlying disorders such as Alzheimer’s or Parkinson’s opens doors for early diagnostics and interventions.
- Integrative Models of Learning and Memory: Enhanced knowledge of neuronal pathways will refine existing models of cognitive processes, allowing us to teach and learn more effectively.
In summary, research into the function and role of neurons is poised to usher in transformative changes across multiple scientific domains, with promising implications for health, education, and technology.