Understanding Messenger RNA: Its Role and Importance
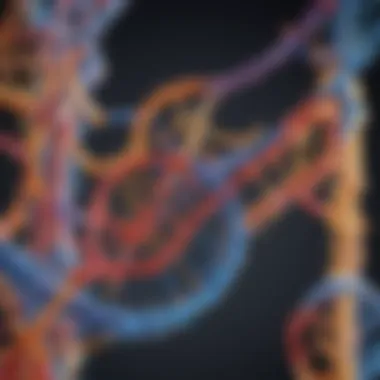
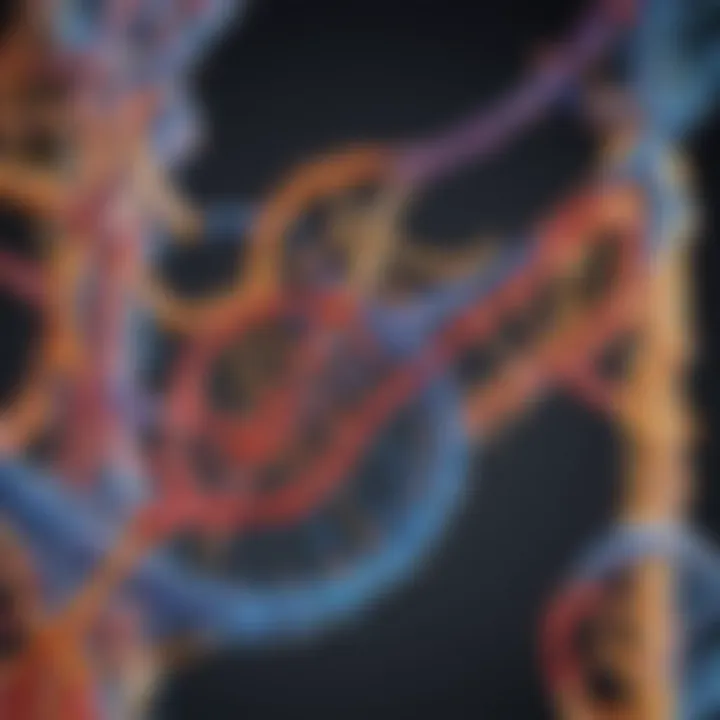
Intro
Messenger RNA, commonly known as mRNA, serves a critical role in the molecular biology of cells. It acts as a template for protein synthesis, enabling the translation of genetic information from DNA into functional proteins. Understanding mRNA is essential, given its profound implications in both biological processes and medical innovations.
Overview of Research Topic
Brief Background and Context
mRNA was first discovered in the early 1960s. Researchers recognized its function in conveying genetic information from DNA to ribosomes, the cellular machinery responsible for protein synthesis. As a single-stranded molecule, mRNA differs from DNA in structure, providing it the ability to direct protein production efficiently. This dynamic allows cells to respond to various stimuli, emphasizing the adaptability of biological systems.
Importance in Current Scientific Landscape
In recent years, mRNA has garnered significant attention, particularly in the context of vaccine development and therapeutic interventions. The rapid advancements in mRNA technology, especially during the COVID-19 pandemic, have demonstrated its potential for revolutionizing medicine. As scientists explore additional applications of mRNA delivery systems, its significance continues to grow.
"The versatility of mRNA technology opens new avenues in therapeutic development, revolutionizing our approach to vaccine design and disease treatment."
Methodology
Research Design and Approach
Analyzing the importance of mRNA involves a multifaceted approach. Various studies are indispensable in assessing its role in cellular processes and therapeutic applications. Researchers typically employ both laboratory experiments and computational modeling to gain insights into mRNA dynamics.
Data Collection Techniques
Data related to mRNA is gathered through several methods, including:
- Experimental assays: Utilizing techniques such as Northern blotting and RT-qPCR to quantify mRNA levels.
- High-throughput sequencing: Identifying mRNA transcripts on a larger scale.
- Bioinformatics tools: Analyzing mRNA sequences for functional predictions.
Understanding these methodologies is crucial for appreciating the complexities of mRNA research and its implications for the scientific community.
Prologue to Messenger RNA
Messenger RNA is essential in the understanding of molecular biology and genetics. It serves as a critical intermediary that conveys genetic information from DNA to the cellular machinery responsible for protein synthesis. This section focuses on the importance of messenger RNA in cellular functions, highlighting key elements, benefits, and considerations regarding its role in biological systems.
Overview of Nucleotides
Nucleotides are the fundamental building blocks of nucleic acids, including both DNA and RNA. Each nucleotide consists of three components: a phosphate group, a sugar molecule, and a nitrogenous base. In the context of RNA, the sugar is ribose, which differs from the deoxyribose found in DNA. There are four types of nitrogenous bases in RNA: adenine, guanine, cytosine, and uracil. The sequence of these nucleotides encodes the genetic instructions necessary for cellular function.
The arrangement of nucleotides within messenger RNA influences its stability and function. For instance, the presence of a 5' cap and a poly-A tail at the ends of the mRNA molecule protects it from degradation and aids in the translation process. Understanding nucleotides is therefore foundational for comprehending the role of mRNA in genetics.
Role of RNA in Cellular Biology
RNA fulfills several crucial roles in cellular biology beyond serving as a messenger. It acts in various capacities, such as catalyzing biochemical reactions and regulating gene expression. RNA molecules, particularly ribosomal RNA and transfer RNA, collaborate with mRNA during the process of translation, which is vital for protein assembly.
Messenger RNA is particularly critical in gene expression regulation. It can affect how genes are expressed by determining the amount of protein produced in response to various cellular signals. Furthermore, the study of RNA can reveal insights into cellular metabolism and intricate networks of gene regulation.
"Messenger RNA is not just a template; it is a dynamic player in the cellular orchestra of life."
In summary, messenger RNA plays a significant role in translating genetic information into functional proteins, impacting cellular structure and function directly. Understanding its intricacies paves the way for advances in biotechnology and therapeutic applications.
Defining Messenger RNA
Defining messenger RNA (mRNA) is crucial to understanding its role in cellular processes. mRNA serves as the intermediary between DNA and protein synthesis. This section explores its definition and its significance in biology and biotechnology.
What is Messenger RNA?
Messenger RNA, also known as mRNA, is a single-stranded molecule transcribed from the DNA in a cell's nucleus. Its primary role is to carry genetic information from the DNA to the ribosomes, where proteins are synthesized. The mRNA molecule encodes the sequence of amino acids that make up a protein. This process begins with transcription, where a specific gene in the DNA is copied to form mRNA, and then followed by translation, where ribosomes read the mRNA to produce proteins.
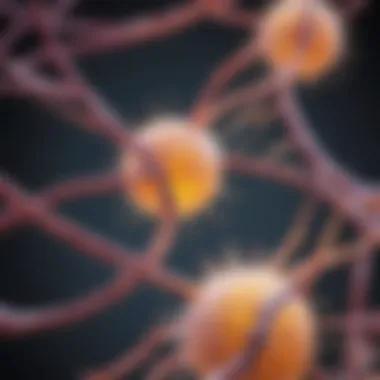
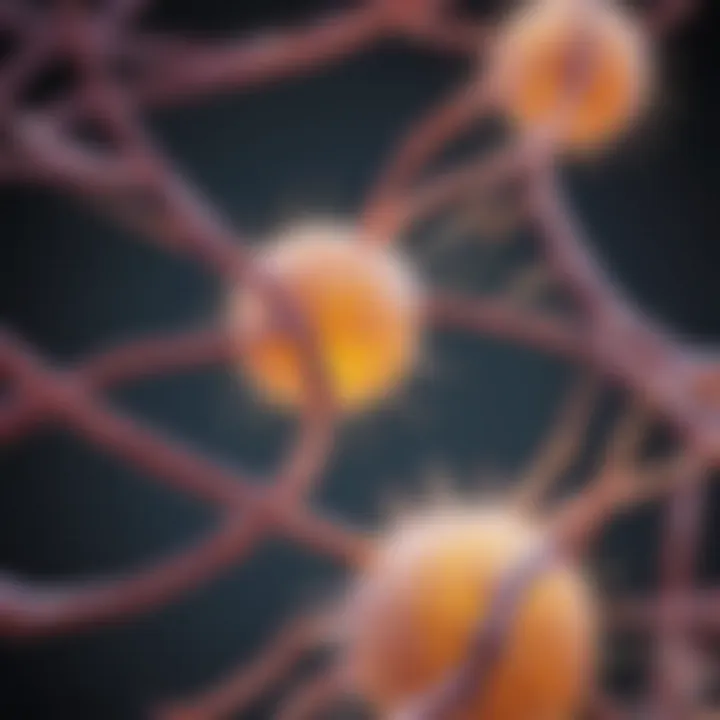
The structure of mRNA includes a sequence of nucleotides, which are the building blocks. Each nucleotide consists of a sugar, a phosphate group, and a nitrogenous base. The sequence of these bases is critical as it determines the order of amino acids in a protein, hence affecting protein function.
Comparison with Other Forms of RNA
Understanding mRNA requires comparing it with other types of RNA. Each type has its distinct role in the process of gene expression and protein synthesis.
Transfer RNA (tRNA)
Transfer RNA, or tRNA, is essential for translating the mRNA sequence into proteins. Each tRNA molecule carries a specific amino acid and recognizes the corresponding codon on the mRNA during translation. This specificity is crucial, as the accuracy of protein synthesis depends on correct amino acid placement.
One key characteristic of tRNA is its cloverleaf shape, which facilitates the binding of amino acids. This feature allows tRNA to function effectively during translation. Its unique ability to adapt to various codons makes it a vital component in the translation machinery. However, tRNA cannot initiate protein synthesis and relies on other molecules like mRNA and ribosomes for this task.
Ribosomal RNA (rRNA)
Ribosomal RNA, or rRNA, forms the core of ribosome structure and is essential for protein synthesis. It plays a significant role in catalyzing the formation of peptide bonds between amino acids. rRNA constitutes a substantial part of the ribosomal subunits, providing the enzymatic activity needed for translation.
A notable characteristic of rRNA is its complex secondary structure, which is required for its function in the ribosome. This unique nature makes rRNA irreplaceable in the translation process. However, unlike mRNA, rRNA does not encode the information for amino acids but instead serves as a scaffold for assembling the translation machinery.
Overall, mRNA is distinct from tRNA and rRNA due to its specific role as the messenger of genetic information, crucial for the synthesis of proteins.
The Structure of Messenger RNA
The structure of messenger RNA (mRNA) plays a significant role in its functionality within cellular processes. Understanding this structure contributes valuable insights into how mRNA operates in the contexts of protein synthesis and gene expression. It also offers clues about its interactions with other cellular components. The structure of mRNA is not just a mere arrangement of nucleotides; it is fundamental to its ability to convey genetic information from DNA to ribosomes, where proteins are synthesized.
Components of mRNA
Nucleotide Sequence
The nucleotide sequence is the basis of mRNA. Each sequence dictates which amino acids are assembled into a protein. This property is central to the biological significance of mRNA. Each nucleotide contains a nitrogenous base, a ribose sugar, and a phosphate group. The arrangement of these bases—adenine (A), uracil (U), cytosine (C), and guanine (G)—determines the specific instructions for protein synthesis. The key characteristic making the nucleotide sequence advantageous for mRNA is its direct role in coding for proteins. The unique feature of the nucleotide sequence lies in its potential for coding a variety of proteins due to the vast array of combinations possible among the four bases. However, mutations or errors in this sequence can result in dysfunctional proteins, highlighting a disadvantage.
' Cap
The 5' cap is another critical component of mRNA. It consists of a modified guanine nucleotide that is added to the beginning of the mRNA transcript. This cap serves multiple roles; it protects mRNA from degradation and aids in the initiation of translation. The primary advantage of having a 5' cap is that it enhances the stability of mRNA, allowing for a more efficient translation process. The unique feature of the 5' cap is its ability to interact with the ribosome, facilitating the binding process during translation. Without this protective element, mRNA would be less stable and more susceptible to degradation, which could compromise its functionality.
Poly-A Tail
The poly-A tail is a string of adenine nucleotides added to the 3' end of the mRNA. This component is vital for the stability and longevity of the mRNA molecule. The poly-A tail enhances the efficiency of translation by promoting ribosome binding and preventing degradation. Its key characteristic is that it increases the half-life of the mRNA in the cytoplasm, allowing more time for translation to occur. The unique feature of the poly-A tail is its regulatory function; it can influence how much protein is produced from a given mRNA molecule. However, if the poly-A tail is too short, it can lead to rapid degradation and a decrease in protein synthesis.
Secondary Structure of mRNA
The secondary structure of mRNA refers to the way the molecule folds into specific shapes due to interactions between its nitrogenous bases. This folding can affect mRNA stability and its translation efficiency. Secondary structures can include hairpins and loops, which arise from complementary base pairing. These formations can create regions that are more or less accessible for ribosome binding. The significance of understanding the secondary structure of mRNA is that it can inform researchers about potential regulatory mechanisms influencing gene expression. Recognizing these structures is critical for advancements in biotechnology and therapeutic applications.
Functionality of Messenger RNA
Messenger RNA (mRNA) is essential for the flow of genetic information within a cell. Its main role lies in the transmission of genetic instructions from DNA to the ribosomes, where proteins are synthesized. Understanding the functionality of mRNA is crucial, as it aids in appreciating its broader significance in biological processes and biotechnology innovations.
Transcription Process
The transcription process is the first step in gene expression. During this process, an enzyme called RNA polymerase binds to a specific segment of DNA. It then synthesizes a complementary strand of RNA, which becomes the mRNA. This mRNA copy carries the genetic code from the DNA—located in the nucleus—to the cytoplasm, where protein synthesis occurs.
This transcription of DNA into RNA is a precise and tightly regulated process. Errors during transcription can lead to malfunctioning proteins, which can negatively affect cellular health. Therefore, high fidelity during transcription is vital.
Translation Mechanism
After transcription, mRNA undergoes a process known as translation. This takes place in the cytoplasm and involves several key components to form proteins. The mechanism behind translation is intricate and critical for cellular function. Below are key aspects that define its importance in understanding mRNA.
Role of Ribosomes
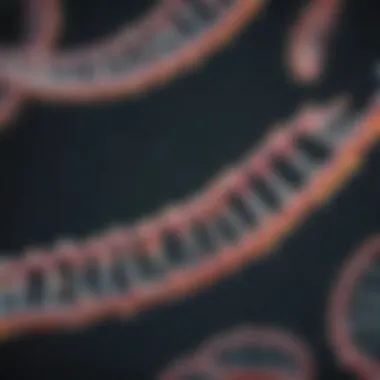
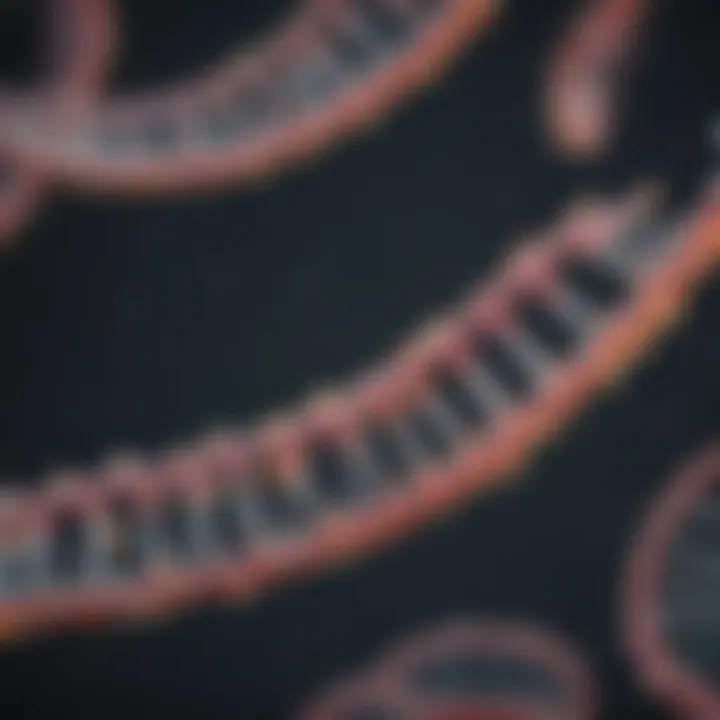
Ribosomes are often called the "molecular machines" of the cell. They serve as the site for protein synthesis. These structures read the sequence of mRNA and facilitate the translation into amino acids.
A key characteristic of ribosomes is their ability to bind with mRNA through its ribosomal RNA (rRNA) component. This feature allows them to accurately interpret the genetic message encoded in mRNA, leading to the synthesis of proteins. The efficiency and accuracy of ribosomes play a significant role in maintaining protein synthesis in cells.
Ribosomes are essential in determining the speed and accuracy of protein synthesis, making them indispensable in cellular functions.
Initiation, Elongation, Termination
The phases of translation consist of initiation, elongation, and termination. Each of these phases plays a vital role in the overall efficiency of protein production.
- Initiation is where the ribosome assembles around the mRNA and the first tRNA (transfer RNA) molecule attaches. This sets up the stage for translation.
- In the elongation phase, amino acids are sequentially added based on the codons present in the mRNA strand. The ribosome facilitates the binding of tRNA to the mRNA, ensuring that amino acids are added in the correct order.
- Termination occurs when the ribosome encounters a stop codon on the mRNA. This signals the end of protein synthesis, and the newly formed protein is released.
The sequential nature of this process highlights the importance of precision at each stage. Any slight deviation during initiation, elongation, or termination can lead to incorrect protein formation. These aspects contribute significantly to our understanding of mRNA functionality and its implications for cellular biology.
Significance of Messenger RNA in Protein Synthesis
Messenger RNA (mRNA) holds a pivotal role in the complex process of protein synthesis, functioning as a critical intermediary between genetic information and the construction of proteins. Understanding its function in this context enhances our comprehension of cellular processes, making mRNA a topic of crucial relevance in cellular biology and biotechnology. By examining the mRNA’s role, we can appreciate the intricate mechanisms that govern the flow of genetic information from DNA, through RNA, to proteins, which are essential for life.
Mechanism of Protein Synthesis
The process of protein synthesis is fundamentally divided into two main stages: transcription and translation.
- Transcription: This initial stage takes place in the nucleus. During transcription, a specific segment of DNA is transcribed to produce mRNA. RNA polymerase, an enzyme, binds to the promoter region of the gene and unwinds the DNA strands. The RNA polymerase then synthesizes a strand of mRNA by adding RNA nucleotides that are complementary to the DNA template strand. This results in a pre-mRNA that undergoes splicing to eliminate introns, producing a mature mRNA molecule.
- Translation: The mature mRNA exits the nucleus and enters the cytoplasm, where it engages in translation. Ribosomes, which are molecular machines composed of ribosomal RNA (rRNA) and proteins, play a crucial role in translating mRNA into a specific protein. The ribosome reads the mRNA in sets of three nucleotides, called codons. Each codon corresponds to a specific amino acid. Transfer RNA (tRNA) molecules, each carrying an amino acid, bind to the corresponding codons on the mRNA sequence. This assembly process continues, yielding a polypeptide chain that eventually folds into a functional protein.
This precise coordination illustrates the significance of mRNA not only as a template for protein synthesis but also as a regulator that influences which proteins are produced in a cell and when.
Amplifying Gene Expression
mRNA acts as a dynamic controller of gene expression, determining both the quantity and timing of protein production. The following aspects highlight its amplifying role:
- Regulation of mRNA Stability: The stability of mRNA in the cytoplasm can influence how long it remains available for translation. Factors such as the 5' cap and the length of the poly-A tail play significant roles in protecting mRNA from degradation.
- Translational Control: The efficiency by which mRNA is translated can be modulated by the availability of ribosomes and tRNA. This allows cells to respond rapidly to changes in environmental conditions or developmental cues.
- Alternative Splicing Variants: The process by which different mRNA molecules are generated from a single gene through alternative splicing expands the range of proteins that can be synthesized from a limited set of genes. This mechanism amplifies the potential for diversity in protein function and regulation.
Researchers continue to explore how mRNA can be targeted to enhance gene expression for therapeutic purposes, illuminating its importance in modern molecular biology and biotechnology. By understanding these nuances, we can appreciate mRNA’s role as a central player in the orchestration of cellular functions.
Messenger RNA in Biotechnology
Messenger RNA plays a pivotal role in biotechnology, influencing various fields including genetic engineering and medical advancements. With its capability to convey genetic information from DNA to the ribosome, mRNA has become a fundamental element in the manipulation and application of genetic material. Its unique properties and functionality offer a myriad of possibilities that extend well beyond traditional biomolecular interactions.
The benefits of utilizing mRNA in biotechnology are plentiful. One primary advantage is its ability to serve as a template for the synthesis of proteins without the need for complex steps involved in other methods. This has streamlined processes in research and development, making it easier to produce proteins recombinantly. Furthermore, mRNA does not integrate into the host genome, reducing the risk of unintended genetic consequences, which is a crucial consideration in the realm of genetic engineering.
"The ability of mRNA to code for proteins in a transient manner is essential for advancing our understanding of gene function and regulation."
Additionally, mRNA-based technologies are relatively quick to develop compared to traditional DNA-based techniques. The rapid production process is invaluable in situations requiring urgent solutions, such as vaccine development or responding to emergent health threats.
Applications in Genetic Engineering
The applications of mRNA in genetic engineering are diverse, allowing for innovative solutions to complex problems. One notable application is the development of mRNA vaccines. Unlike conventional vaccines that often use live attenuated or killed pathogens, mRNA vaccines instruct cells to produce a harmless piece of the pathogen's protein, stimulating an immune response. This approach not only enhances safety but also improves efficacy as the immune system recognizes and learns to combat real threats.
Moreover, mRNA can be utilized in the field of synthetic biology, where researchers are engineering organisms to produce desired compounds. By inserting specific mRNA sequences into microbes, it is possible to enhance the synthesis of pharmaceuticals, biofuels and other beneficial products. This method has the potential to revolutionize industrial processes, making them more sustainable and efficient.
Role in Vaccine Development
The role of mRNA in vaccine development has gained significant prominence, especially highlighted by the rapid responses to global health crises. mRNA vaccines, such as those developed by Pfizer-BioNTech and Moderna for COVID-19, exemplify the enormous potential of this technology. The procedure involves delivering a small, synthesized strand of mRNA that encodes a specific viral protein into human cells. Once inside, the cells manufacture the protein, activating an immune response that prepares the body to fight real infections.
This mRNA approach offers several advantages in vaccine development:
- Speed: The mRNA platform allows for fast design and production. Researchers can quickly adapt mRNA sequences to new pathogens, expediting vaccine availability.
- Safety: As mRNA does not use live viruses, it minimizes the risk of causing disease, which is a significant advantage over traditional vaccine methods.
- Efficacy: mRNA vaccines have shown robust responses, leading to high protection rates against diseases.
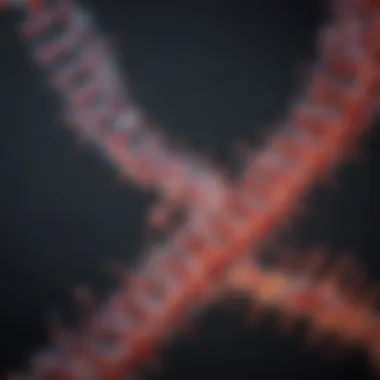
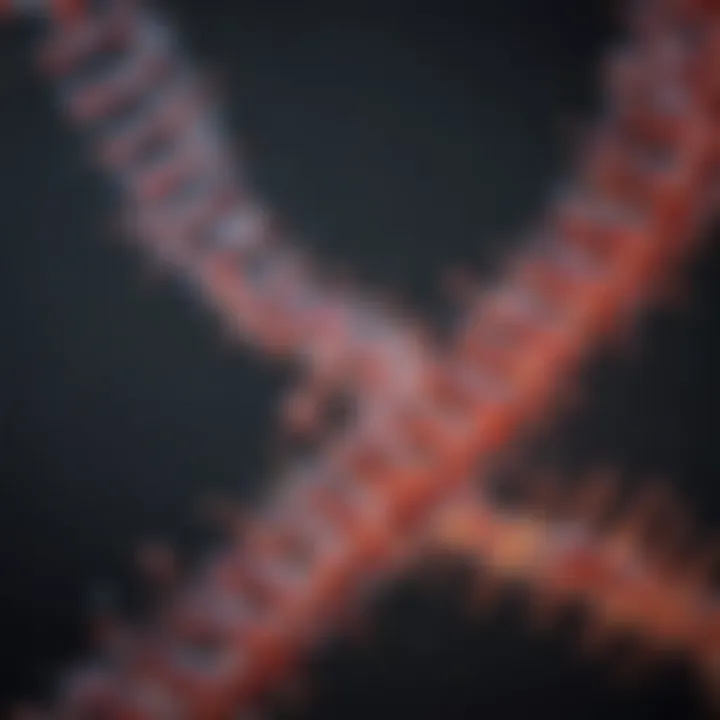
Recent Advances in mRNA Research
Recent advances in mRNA research have unveiled a multitude of significant insights into the functioning, applications, and enhancements of messenger RNA. This research is crucial, particularly given the burgeoning interest in mRNA's role in therapeutics and its ability to influence gene expression. Such progress opens doors to innovative treatment strategies and expanded understanding of cellular mechanics. The implications are far-reaching, affecting fields like biotechnology, pharmacology, and personalized medicine.
Innovations in mRNA Therapeutics
Innovations in mRNA therapeutics have underscored the potential of mRNA to serve as a platform for developing vaccines and treatments. The rise of mRNA vaccines, particularly visible during the COVID-19 pandemic, demonstrated both efficacy and safety in rapid vaccine development.
- Vaccine Development: The Pfizer-BioNTech and Moderna COVID-19 vaccines utilized lipid nanoparticles to deliver modified mRNA to cells. This approach resulted in a swift production of the immune response, showcasing how mRNA can enable rapid response to emerging infectious diseases.
- Cancer Treatments: mRNA is being explored in cancer therapies as well, where it can instruct cells to produce antigens that trigger an immune response. This creates a tailored approach, utilizing the body's own immune system to combat tumors.
- Genetic Disorders: Experimental therapies are attempting to correct genetic defects by introducing functional copies of genes via mRNA. This is particularly promising for diseases where current gene therapies have limitations.
The versatility of mRNA in these applications highlights its role as a pivotal player in the advancement of modern medicine, transitioning from theoretical concepts to practical solutions.
Challenges and Perspectives
While advances in mRNA research present numerous opportunities, the field encounters significant challenges that must be addressed to realize its full potential.
- Stability and Delivery: One of the key challenges lies in the stability of mRNA molecules. They can be prone to degradation, necessitating effective delivery systems to ensure adequate amounts reach target cells.
- Immune Response: mRNA can inadvertently trigger immune responses that may limit its effectiveness. Fine-tuning the design of mRNA sequences and delivery methods is an ongoing area of exploration.
- Cost of Production: Current production methods for mRNA vaccines and therapeutics can be expensive. Developing more cost-effective production processes is essential to making these technologies widely accessible.
Overall, the perspectives surrounding mRNA research remain promising. Continued investment in research and development will likely lead to overcoming these obstacles, enabling the broader application of mRNA technology across various therapeutic areas.
The significant advances seen in mRNA research illuminate the transformative potential of this molecule, setting a foundation for the next generation of medical therapies.
Future Directions in Messenger RNA Studies
The exploration of messenger RNA (mRNA) continues to evolve, presenting numerous opportunities in medical science. Understanding these future directions is pivotal. Scientists aim to alter and enhance mRNA to improve therapeutic outcomes. The advances in mRNA technology are not just theoretical but hold real promise for practical application.
Potential of mRNA in Personalized Medicine
Personalized medicine tailors treatments to individual characteristics. mRNA plays a crucial role here. It allows for the development of therapies that align with a person’s unique genetic makeup. By utilizing mRNA in this way, researchers can create targeted treatments. These treatments may be more effective and have fewer side effects compared to traditional methods.
For example, cancer therapies that target specific genetic mutations are gaining traction. This approach enhances the likelihood of successful treatment outcomes.
"Personalized mRNA therapies could redefine how we approach conditions like cancer and genetic disorders."
Moreover, mRNA can offer benefits in infectious disease treatment. Vaccines that leverage mRNA technology have shown promise in creating personalized immune responses. This adaptability can lead to rapid updates in vaccines, ensuring they are effective against emerging strains of viruses.
Exploring Novel Therapeutic Applications
The future of mRNA research also looks at innovative therapeutic applications beyond vaccines. One significant direction involves gene editing. mRNA could aid in the delivery of CRISPR components to specific cells. By precisely targeting where modifications occur, scientists can reduce unintended effects.
Another area of focus is in rare genetic disorders. Many conditions arise from a single faulty gene. mRNA therapy could potentially introduce the correct information for making proteins. This straightforward approach might correct the underlying issue at the source.
Additionally, research is underway to utilize mRNA for the treatment of autoimmune diseases. By moderating the immune response, mRNA can help prevent the body from attacking itself. This intervention opens new avenues for long-term management of chronic diseases.
The advancements in mRNA studies signify a major step in the field of biotechnology. Understanding personalized medicine and novel applications are crucial for future development in this area. Emphasizing safe, effective, and targeted therapies will define the next wave of mRNA research and applications.
Epilogue
The conclusion serves as a critical component of this article, synthesizing the information presented throughout and emphasizing the continued relevance of messenger RNA in various scientific fields. By summarizing key points, it provides clarity and reinforces the significance of mRNA in cellular processes.
Recap of Main Points
In this article, we have explored several essential aspects of messenger RNA:
- Definition and Role: Messenger RNA is a vital molecule responsible for transmitting genetic information from DNA to the ribosomes, facilitating protein synthesis.
- Structure and Functionality: The structural components of mRNA, including the nucleotide sequence, 5' cap, and poly-A tail, were discussed, highlighting their roles in stability and translation.
- Significance in Biotechnology: The application of mRNA in genetic engineering and vaccine development, especially in the wake of recent global health challenges, reflects its transformative potential.
- Future Directions: The prospects of mRNA in personalized medicine and novel therapeutic applications suggest a bright future for research and practical applications.
The Ever-Increasing Relevance of mRNA
Messenger RNA is becoming increasingly relevant in the modern scientific landscape due to its numerous implications in health, disease, and therapy. As research continues to advance, several elements contribute to this growing importance:
- Innovative Therapeutics: mRNA-based therapeutics, such as the mRNA vaccines for COVID-19, demonstrate how quickly science can adapt and respond to urgent health needs.
- Gene Expression Regulation: Ongoing studies focus on how mRNA plays a role in gene expression modulation, offering avenues for targeted therapies in cancer and genetic disorders.
- Technological Advances: The development of new techniques for synthesizing and delivering mRNA is likely to continue accelerating the pace of research and application in biotechnology.
In sum, the understanding of messenger RNA is not only foundational to molecular biology but also increasingly relevant to addressing contemporary challenges in medicine and technology.