Understanding DNA-E Protein: Insights and Implications
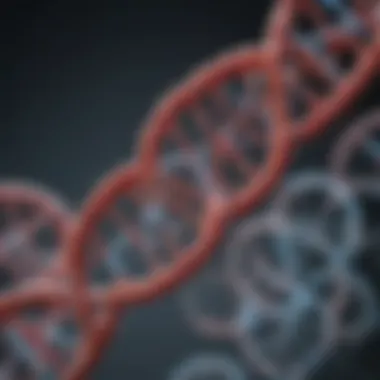
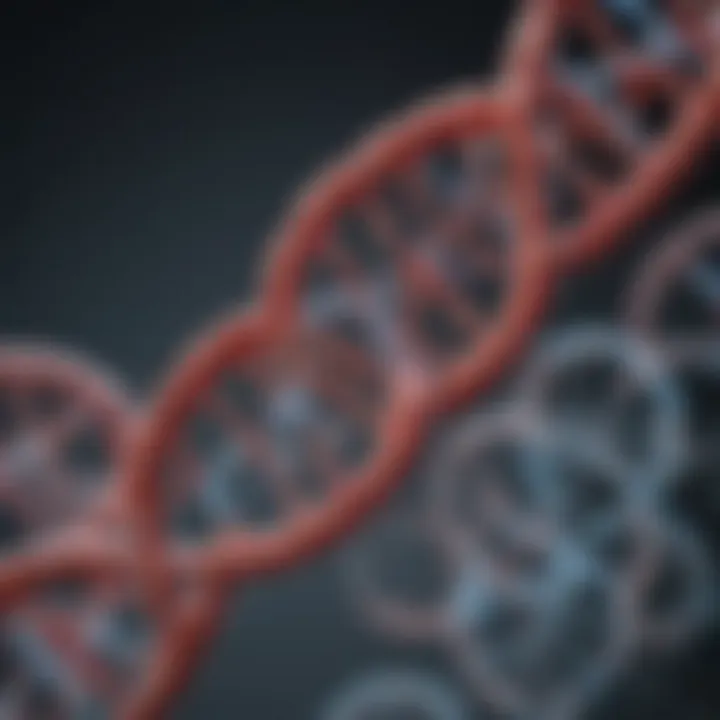
Overview of Research Topic
Brief Background and Context
DNA-E protein is an essential component in the realm of molecular biology, contributing significantly to various biological processes. Its complexity arises from its structural characteristics and function within cellular environments. Understanding this protein requires a thorough examination of its synthesis, interactions, and the regulatory mechanisms it influences. Emerging research continues to probe its significance, offering insights that could reshape how scientists view genetic expression and regulation.
Importance in Current Scientific Landscape
The relevance of DNA-E protein cannot be understated in contemporary research. As scientists delve deeper, they uncover the intricate roles this protein plays in cellular processes. The evolution of genetic studies highlights the importance of DNA-E protein in various domains, from genetic engineering to disease research. Its implications stretch beyond conventional boundaries, offering opportunities for advancements in biotechnology and therapeutic interventions.
Methodology
Research Design and Approach
The investigative framework surrounding DNA-E protein often employs a variety of research methodologies. Most studies combine experimental techniques with computational modeling to generate robust data. This dual approach allows for comprehensive insights into protein function and its biological role. Laboratory experiments frequently focus on biosynthesis pathways and protein behavior within cellular contexts.
Data Collection Techniques
The data collection methods vary based on the goals of the research. Some common techniques used include:
- Western Blotting: This method is crucial for detecting specific proteins in a complex mixture, allowing researchers to analyze the expression levels of DNA-E protein.
- Mass Spectrometry: It provides detailed insights into the structure and modifications of the protein, crucial for understanding its functionality.
- Genomic Studies: Analyzing genetic material can reveal interactions and evolutionary significance of DNA-E protein across different organisms.
"DNA-E protein’s multifaceted roles make it a focal point for both basic research and applied sciences."
Foreword to DNA-E Protein
DNA-E protein plays a crucial role in the realm of molecular biology. It is essential for the regulation of various cellular processes, such as gene expression and protein synthesis. By studying DNA-E protein, researchers can gain insights into its involvement in genetic regulation and its potential applications in genetic engineering. Understanding this protein is important because it serves as a platform for further discoveries in the field.
Defining DNA-E Protein
DNA-E protein is a type of protein that is integral to the functioning of numerous biological processes at the molecular level. It is commonly associated with the replication of DNA and the stabilization of DNA structures. The actual structure and function of DNA-E proteins can vary among different organisms, reflecting diverse evolutionary paths. This adaptability makes it a subject of interest for studies aimed at comprehending both basic and complex biological systems.
Historical Context and Discovery
The discovery of DNA-E protein dates back to the mid-20th century when researchers began to unravel the complexities of genetic material. Initial studies focused on the basic components of DNA and its derivatives. The significance of DNA-E protein emerged as scientists delved deeper into genetic regulation. Over time, advancements in molecular biology techniques led to the identification and characterization of DNA-E protein in various species. This historical context illustrates how scientific inquiry evolves, uncovering the crucial roles of proteins like DNA-E in biological functionality.
Molecular Structure of DNA-E Protein
Understanding the molecular structure of DNA-E protein is crucial in catalyzing deeper insights into its biological functions. The intricate arrangement of its amino acids not only defines the protein's shape but also directly correlates with its role in various cellular processes. This section elaborates on different aspects of the structure, guiding readers through its primary structure, secondary and tertiary configurations, and the vital functional domains and motifs that together create the functional landscape of DNA-E protein.
Primary Structure
The primary structure of DNA-E protein refers to the linear sequence of amino acids that form its backbone. This specific arrangement is determined by the genetic code from the corresponding DNA. Each amino acid is connected via peptide bonds, creating a polypeptide chain. The sequence matters significantly; even a single change can alter the protein's ability to function properly.
- Significance of the Sequence: The arrangement influences folding and stability.
- Stability Factors: Factors like hydrophobicity and charge play a role.
- Genetic Encoding: Mutations in the genetic coding can lead to variations in protein function, which can have downstream effects in biological systems, potentially leading to various diseases.
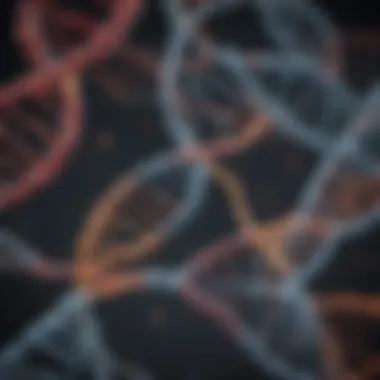
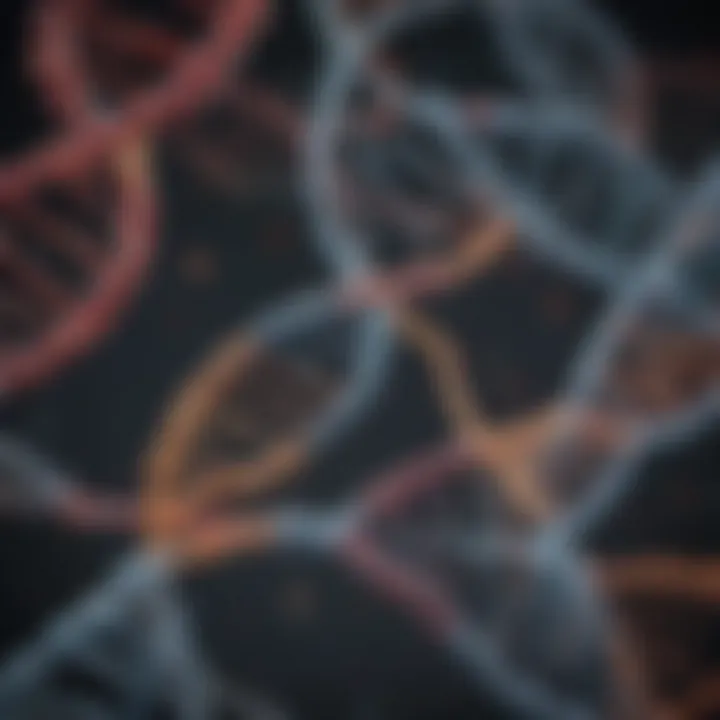
A deep understanding of this structure can help in predicting how the protein will behave when synthesized in cells.
Secondary and Tertiary Structures
The secondary and tertiary structures emerge as the polypeptide chain folds into specific shapes due to interactions between non-adjacent amino acids. In DNA-E protein, common secondary structures include alpha-helices and beta-sheets, stabilized by hydrogen bonds.
- Alpha-helices: They provide flexibility and resilience in the protein's framework.
- Beta-sheets: They offer strength and stability, serving as a scaffold for functional motifs.
The tertiary structure encompasses the overall three-dimensional shape of the protein, formed by interactions among the R groups of the amino acids. These interactions can include hydrophobic interactions, ionic bonds, and Van der Waals forces. Understanding these structures is essential for the following reasons:
- Functionality: The specific folded shape is where the protein attains its biological function.
- Interaction with Other Molecules: This structure can create binding sites for other cellular components, influencing the protein's role in gene expression, signaling, and enzymatic activities.
Functional Domains and Motifs
Functional domains and motifs represent specific regions within the DNA-E protein that are responsible for its activities. These are distinct parts of the protein that have specific roles, often conserved across different proteins.
- Domains: Each domain can contribute uniquely, for example, DNA-binding domains are crucial for interactions with genetic materials.
- Motifs: Simple recurring patterns that may indicate specific functions or structural properties.
In the context of DNA-E protein, the identification of these domains can uncover:
- Mechanisms of Action: How the protein interacts with other proteins, RNA, or DNA members.
- Targets for Research: Understanding what these domains do can lead researchers to discover new applications in genetic engineering or therapy.
"The molecular architecture of DNA-E protein is not just a blueprint; it is the manual of life that instructs proteins on their tasks within the cell."
In summary, the molecular structure is vital for appreciating the full potential of DNA-E protein in biological contexts. Each level of structure contributes to its overall function, shedding light on its essential role in gene regulation and cellular function.
Synthesis and Regulation of DNA-E Protein
The synthesis and regulation of DNA-E protein is vital for understanding its functional role in various biological processes. This section unfolds the intricate mechanisms that govern the production of DNA-E protein, detailing the regulatory elements involved and their significance in cellular activities. The synthesis process ensures that DNA-E protein is produced in appropriate amounts, aligning with the cell's needs and contributing to its overall homeostasis.
Gene Regulation Mechanisms
Gene regulation mechanisms are essential for controlling the expression of DNA-E protein. These mechanisms can be categorized into several layers, including transcriptional, post-transcriptional, and epigenetic regulation. Each layer plays a distinctive role in ensuring that DNA-E protein is synthesized accurately.
- Transcriptional Regulation: This involves activators and repressors that interact with promoter regions of the gene coding for DNA-E protein. Transcription factors bind to these regions, facilitating or inhibiting the transcription process. For example, the presence of specific transcription factors can enhance the gene's expression, while the absence or binding of repressors may lead to decreased synthesis.
- Post-Transcriptional Regulation: Once the mRNA is produced, it undergoes various modifications such as splicing, capping, and polyadenylation. These processes are crucial in determining the stability and translational efficiency of the mRNA. MicroRNAs (miRNAs) also play a significant role in post-transcriptional regulation, where they can bind to target mRNAs, leading to degradation or inhibition of translation, thus affecting DNA-E protein levels.
- Epigenetic Regulation: This layer involves modifications to DNA and histone proteins that affect gene expression without altering the underlying DNA sequence. Methylation of DNA and the acetylation or deacetylation of histones can either promote or inhibit access of transcription machinery to the DNA. Understanding these epigenetic factors is important for comprehending how DNA-E protein expression can be influenced by environmental factors.
Transcription and Translation Processes
The transcription and translation processes are key components in the synthesis of DNA-E protein. The two steps work in tandem, converting genetic information into functional proteins.
- Transcription Process: This begins with the unwinding of the DNA double helix, allowing RNA polymerase to synthesize a complementary strand of mRNA from the template DNA. This process is tightly regulated. Key factors like promoter sequences and enhancers are crucial in determining the efficiency of transcription initiation.
- Translation Process: After transcription, the mRNA molecule is transported to the ribosome, the site of protein synthesis. The ribosome reads the mRNA sequence, interpreting it in sets of three nucleotides, known as codons. Each codon corresponds to a specific amino acid, which is brought to the ribosome by transfer RNA (tRNA). The ribosome catalyzes the formation of peptide bonds between adjacent amino acids, resulting in the formation of the DNA-E protein.
In summary, understanding the synthesis and regulation of DNA-E protein involves examining gene regulation mechanisms and the transcription and translation processes. These elements are crucial for elucidating how cells control the production of this important protein, influencing various cellular functions.
Function of DNA-E Protein in Cellular Processes
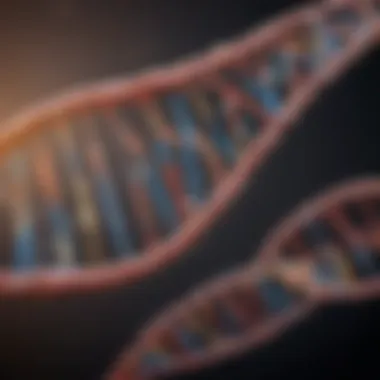
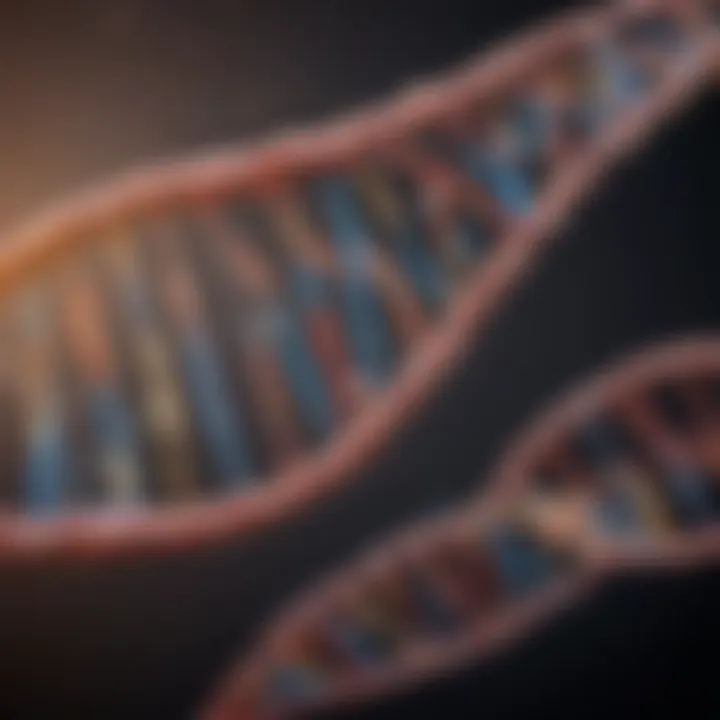
The role of DNA-E protein in cellular processes is fundamentally significant. It acts as a key player in regulating various functions that are vital for cell survival and function. Understanding these functions helps to illuminate the broader complexities of molecular biology. The role of DNA-E protein can be categorized mainly into two areas: gene expression and interaction with other proteins. Each of these areas contributes to the essential tasks that maintain cellular integrity and responsiveness to environmental changes.
Role in Gene Expression
DNA-E protein directly influences gene expression, which is crucial for cellular function and adaptability. This protein is involved in several stages of gene expression, including transcription and post-transcriptional regulation.
- Transcriptional Regulation: DNA-E protein can bind to specific DNA sequences, influencing the transcription of target genes. By modulating the activity of RNA polymerase, DNA-E protein can enhance or repress the transcription of genes essential for various cellular responses, including stress responses and developmental processes.
- Regulation of mRNA Stability: Beyond the initiation of transcription, DNA-E protein can influence the stability of mRNA. This function plays a major role in determining how long mRNA is available for translation into proteins.
- Feedback Mechanisms: DNA-E protein also participates in feedback loops, helping to ensure that gene expression levels are adjusted according to the needs of the cell. These feedback mechanisms are vital for maintaining homeostasis within the cellular environment.
The implications of these functions are vast. By regulating gene expression, DNA-E protein plays a key role in cellular differentiation and response to environmental stress. This can lead to various applications in genetic engineering and biotechnology.
Interaction with Other Proteins
Interaction with other proteins is another essential function of DNA-E protein. This protein does not work in isolation; rather, it forms complexes with an array of other molecules.
- Protein-Protein Interactions: DNA-E protein often interacts with transcription factors and co-regulators. Through these interactions, it helps to build a sophisticated network that fine-tunes gene expression.
- Signaling Pathways: DNA-E protein may also be involved in signal transduction pathways, translating external signals into cellular responses. This ability allows cells to adapt quickly to changes in their environment.
- Multi-Protein Complexes: The formation of multi-protein complexes is critical for the functionality of DNA-E protein. When it interacts with other cellular components, it can affect cellular processes such as DNA repair and apoptosis, further emphasizing its importance.
Understanding the mechanisms behind these interactions can provide insight into the regulation of cellular processes and the development of targeted therapies for various diseases.
In summary, the functions of DNA-E protein in cellular processes highlight its multifaceted role in maintaining cellular function. By regulating gene expression and interacting with other proteins, DNA-E protein ensures that cells can respond appropriately to internal and external stimuli.
Implications of DNA-E Protein in Research
The implications of DNA-E protein within research are vast and highly significant. This protein plays an essential role in understanding genetic processes and molecular interactions. As research progresses, DNA-E protein is noted for its potential applications in various fields including genetic engineering and medicine. Its multifaceted nature warrants attention from both theoretical and applied perspectives, underscoring the necessity of further study.
Applications in Genetic Engineering
Genetic engineering has evolved significantly and DNA-E protein is at the forefront of this advancement. Scientists leverage its unique properties to modify genes and enhance traits in organisms.
- CRISPR Technology: The use of DNA-E protein enhances the efficiency of CRISPR systems, enabling precise gene editing. This protocol opens avenues for developing crop varieties with improved yields or resistance to diseases.
- Gene Therapy: Understanding DNA-E protein contributes to developing gene therapies aimed at repairing or replacing faulty genes. Such techniques show promise in treating genetic disorders, highlighting the protein's relevance in healthcare.
- Synthetic Biology: DNA-E protein assists in constructing synthetic pathways, enhancing the ability to produce complex biomolecules, useful in pharmaceuticals.
Role in Disease Mechanisms
Further investigation of DNA-E protein offers insight into various disease mechanisms. Its role in molecular biology implicates it in several pathological states, improving the understanding of these conditions.
- Cancer Research: Changes in DNA-E protein expression can influence tumor progression. Researching this relationship helps in formulating targeted therapies aimed at cancer treatment.
- Infectious Diseases: Work has shown that DNA-E protein may be involved in the response to viral infections. Understanding its mechanisms can aid in developing antiviral therapies.
- Autoimmune Disorders: The expression levels of DNA-E protein can impact immune responses. Studying this relationship may provide avenues for managing autoimmune diseases more effectively.
"Research into the implications of DNA-E protein is crucial for innovative solutions in genetic disorders and biotechnology."
Ultimately, the implications of DNA-E protein in research extend beyond theoretical considerations. Its applications in genetic engineering and understanding disease mechanisms reveal its potential to reshape both treatment and biotechnology industries.
Current Trends in DNA-E Protein Research
The study of DNA-E protein is evolving rapidly, influenced by technological advancements and a growing understanding of molecular biology. Current trends in DNA-E protein research are crucial as they provide insights into how this protein functions within cells, how it interacts with other biological molecules, and its implications for fields such as genetic engineering and disease treatment.
Innovative Methodologies
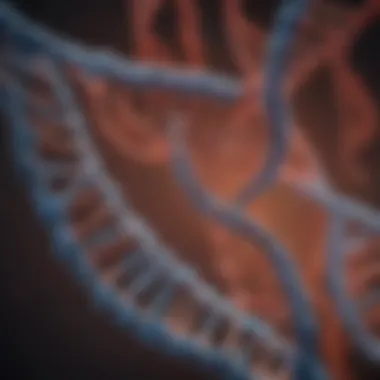
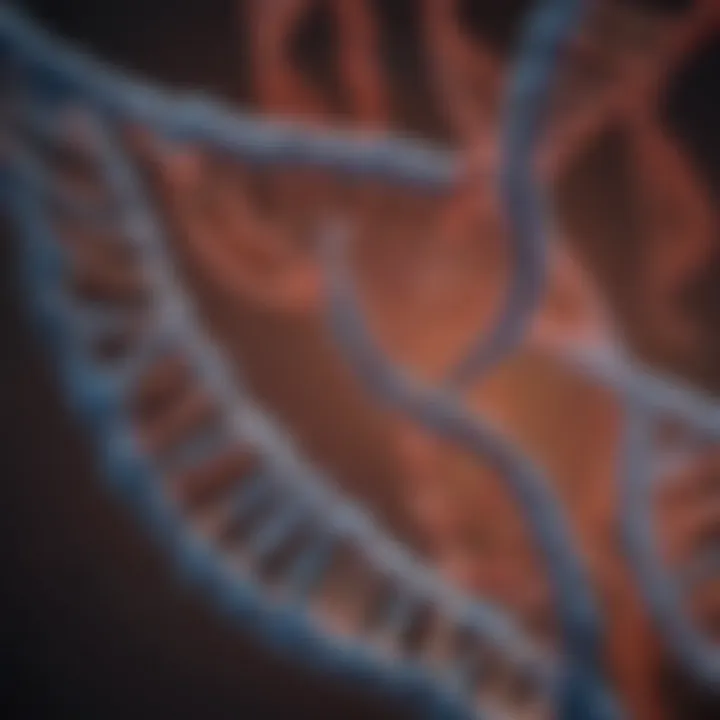
Recent innovations in methodologies are transforming DNA-E protein research. Scientists now employ sophisticated techniques like CRISPR-Cas9 gene editing and high-throughput sequencing. These methods allow researchers to manipulate DNA-E protein coding genes with precision, enhancing the ability to explore its functions in various biological contexts.
- CRISPR-Cas9 Technology: This groundbreaking tool enables targeted editing of the DNA sequence. By applying CRISPR, researchers can disrupt or enhance the expression of the DNA-E protein. This helps understand the specific roles it plays in gene regulation and cellular processes.
- High-Throughput Screening: This approach allows for the simultaneous analysis of large numbers of samples. It facilitates the identification of how DNA-E protein interacts with other molecules, revealing its biological networks.
Additionally, advances in imaging techniques, such as super-resolution microscopy, are providing detailed visualizations of DNA-E protein dynamics within living cells. These techniques are pivotal for understanding the real-time functions of the protein and can lead to further discoveries about its role in health and disease.
Interdisciplinary Approaches
Interdisciplinary approaches are increasingly prominent in DNA-E protein research. Fields such as bioinformatics, structural biology, and systems biology are integrating with molecular biology to provide a comprehensive viewpoint on DNA-E protein.
- Bioinformatics: This field leverages computational tools to analyze large datasets. By using bioinformatics, researchers can predict the potential interactions of DNA-E protein with other biomolecules, thus streamlining hypothesis generation for experimental validation.
- Structural Biology: Examining the three-dimensional structures of DNA-E protein gives insights into how it functions. Techniques like X-ray crystallography and nuclear magnetic resonance (NMR) spectroscopy are employed to elucidate these structures. Understanding the shape and conformation of DNA-E protein is vital for designing targeted therapies in diseases where it plays a role.
- Systems Biology: This holistic approach studies complex interactions within biological systems. It helps to place DNA-E protein in the context of larger biological networks, thereby allowing researchers to understand its influence on cellular homeostasis and other processes.
"The convergence of different fields is redefining boundaries and expanding our understanding of DNA-E protein's significance in biology."
For more insights and updates, articles can be found on platforms like Wikipedia, Britannica, and discussions can be engaged on Reddit or Facebook.
Challenges and Future Directions
In the realm of DNA-E protein research, understanding the challenges and identifying future directions is crucial. This section explores some specific elements that influence the trajectory of research and underscores the benefits of addressing these issues. The complexities inherent in studying DNA-E protein require significant attention. One essential area of focus is the interaction between DNA-E protein and non-coding RNAs. These molecules play a pivotal role in regulating gene expression, which is fundamentally important for understanding cellular functions and the implications in various diseases.
Understanding Non-coding RNA Interactions
The interactions between DNA-E protein and non-coding RNAs can significantly influence gene regulation. Non-coding RNAs, such as microRNAs and long non-coding RNAs, have diverse mechanisms of action, often interacting with various proteins to modulate transcription and translation processes. The precise nature of these interactions remains an incomplete puzzle.
Research is ongoing to unveil how DNA-E protein communicates with these non-coding elements. This understanding could provide insight into how certain diseases emerge and progress, particularly in terms of altered gene expression patterns. The exploration of these interactions enhances our comprehension of molecular biology and paves the way for potential therapeutic interventions.
Addressing Bioethical Considerations
Bioethical considerations are another pressing challenge in the field of DNA-E protein research. As advancements continue, ethical questions arise regarding genetic manipulation and the implications for human health. Gene editing techniques, which may involve manipulating DNA-E protein expressions, bring forth debates about safety, consent, and potential long-term impacts on generations.
Regulatory frameworks and ethical guidelines are necessary to navigate these issues responsibly. Stakeholders, including researchers and policymakers, must collaboratively ensure that the benefits of DNA-E protein research uphold ethical standards. This vigilance is crucial to maintain public trust and promote a transparent discourse on the innovations that could stem from this field.
Ending
In summary, the topic of DNA-E protein holds significant relevance in molecular biology. Understanding its intricate mechanisms and role in cellular processes is critical for advancing research in genetic regulation and disease treatment. The findings from this exploration highlight the multifaceted nature of DNA-E protein and its extensive applications in various scientific domains. By drawing attention to these key elements, the article underscores the importance of integrating this knowledge into the broader context of biological research.
Summary of Key Findings
The article has elucidated several vital points regarding DNA-E protein:
- Structural Characteristics: DNA-E protein exhibits a unique molecular structure that contributes to its functionality.
- Synthesis Mechanisms: Insights into the gene regulation mechanisms reveal how DNA-E protein is synthesized within the cell.
- Interactivity: Its interactions with other cellular components are critical for understanding its role in gene expression.
- Research Applications: DNA-E protein has practical implications in genetic engineering and offers potential insights into disease mechanisms.
By gathering these findings, researchers and professionals can appreciate the significant role DNA-E protein plays in molecular biology.
Implications for Future Research
The future of research centered on DNA-E protein presents numerous exciting opportunities. Key implications include:
- Expanding Gene Interaction Studies: Future studies could further explore interactions with non-coding RNA. This is vital for understanding the full regulatory network involving DNA-E protein.
- Bioethical Reviews: As applications in genetic engineering evolve, addressing bioethical considerations becomes paramount. Developing guidelines ensures responsible usage of findings.
- Integrating Novel Methodologies: There are potential benefits in applying interdisciplinary approaches. This can enhance research outcomes and broaden our comprehension of DNA-E protein’s impact in various biological contexts.
Overall, a nuanced understanding of DNA-E protein not only contributes to its own study but also enriches the entire field of molecular biology.