Power SYBR Green qPCR Protocol for Accurate Quantification
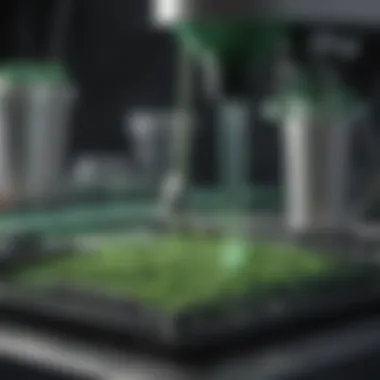
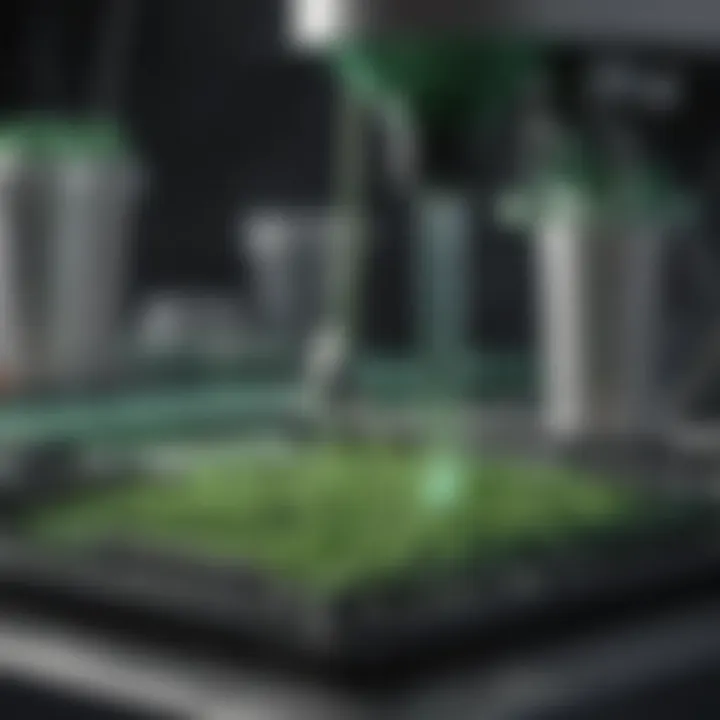
Intro
The Power SYBR Green qPCR protocol represents a critical advancement in molecular biology, particularly in the quantification of nucleic acids. In modern research, the need for precise and reliable measurement techniques has escalated. The flexibility and effectiveness of this protocol have made it widely adopted in various scientific disciplines, from environmental studies to clinical diagnostics.
Understanding this protocol requires not just knowledge of the mechanisms involved, but also the procedures that underpin its success. The steps taken in the SYBR Green qPCR process are significant, ranging from sample preparation to the interpretation of results. It is vital for researchers to master these components to ensure high-quality data.
This article aims to demystify the intricacies of the Power SYBR Green qPCR protocol. By offering detailed guidance on each stage, from reagent selection to final data analysis, it prepares researchers for effective implementation, ultimately enhancing the reproducibility and reliability of experimental results.
Overview of Research Topic
Brief Background and Context
Quantitative Polymerase Chain Reaction (qPCR) has developed into a cornerstone technique in molecular biology. The introduction of SYBR Green, a fluorescent dye that binds specifically to double-stranded DNA, has revolutionized how researchers quantify nucleic acids. Originally, this method relied on intercalating agents, which presented issues in specificity and accuracy. However, with SYBR Green, the quantification process has become more straightforward, allowing real-time monitoring of DNA amplification.
Importance in Current Scientific Landscape
In today’s scientific environment, the importance of quantification cannot be overstated. From gene expression studies to pathogen detection, accurate measurement enables researchers to draw valid conclusions. Power SYBR Green qPCR is known for its efficiency and capability to work with various sample types. This versatility enhances its relevance, as it can be applied in multiple fields including biotechnology, clinical research, and environmental monitoring.
Understanding the principles behind SYBR Green qPCR is essential for anyone engaged in molecular biology research. The emphasis on reproducibility and the avoidance of common pitfalls can be the difference between successful experiments and those that produce unreliable data. It is within this framework that the subsequent sections will delve into the methodology, presenting a thorough exploration of how to optimize the protocol for best results.
Intro to SYBR Green qPCR
The Power SYBR Green qPCR protocol is pivotal in molecular biology for accurate quantification of nucleic acids. Understanding SYBR Green qPCR is essential for researchers aiming to implement this technique effectively. This introduction provides the framework for appreciating the nuances of the methodology, the advantages it offers, and considerations that must be taken into account during experimentation.
Overview of qPCR
Quantitative Polymerase Chain Reaction (qPCR) enables the real-time quantification of specific DNA sequences. The technology relies on the amplification of nucleic acids, allowing researchers to assess the amount present in a sample accurately. Unlike traditional PCR, which only provides qualitative results, qPCR provides quantifiable data. This capability is critical in fields such as gene expression analysis, pathogen detection, and genetic variation studies.
Key benefits of qPCR include:
- Sensitivity: Detects low quantities of nucleic acids, offering precise measurements even in complex samples.
- Specificity: Allows for the amplification of specific sequences, minimizing background noise from non-target products.
- Speed: Delivers results in a fraction of the time needed for traditional methods, expediting the research process.
Researchers must, however, pay close attention to various factors that influence results, such as primer design, reagents used, and cycling conditions to ensure reliable data.
What is SYBR Green?
SYBR Green is a fluorescent dye that intercalates into double-stranded DNA. It is a key component in many qPCR experiments due to its ability to emit fluorescence once bound to DNA. This property allows for the detection of amplified products during the qPCR process. The more DNA that is amplified during cycles, the greater the fluorescence emitted, which can be monitored in real-time.
Advantages of using SYBR Green include:
- Cost-Effectiveness: SYBR Green-based assays are generally less expensive than probe-based methods like TaqMan.
- Broad Range of Applications: It can be used for various types of nucleic acids across multiple species.
- Simplicity: The assay design is straightforward, as it requires only one dye for detection, compared to dual-labeled probes.
"SYBR Green offers a versatile and cost-effective solution for researchers delving into nucleic acid quantification."
However, users must also consider some limitations, such as the potential for non-specific binding, which can lead to false positives or inaccurate quantification. Proper experimental design and validation can mitigate these challenges.
Components of the Power SYBR Green qPCR Kit
The Power SYBR Green qPCR Kit contains essential components that are pivotal for the effectiveness and accuracy of qPCR experiments. Understanding these components enables researchers to harness their individual roles in ensuring precision and reliability of quantification. Each element of the kit is formulated to contribute to the overall performance of the assay.
Reagents Overview
At the core of the Power SYBR Green qPCR Kit lies a carefully selected set of reagents. These include:
- SYBR Green Dye: This is a key player in the detection of amplified DNA. It binds to double-stranded DNA molecules, emitting fluorescence that corresponds to the amount of DNA produced in the reaction.
- Taq Polymerase: This enzyme is crucial for the synthesis of new DNA strands during the PCR process. Its high fidelity and resistance to inhibitor substances are vital for accurate results.
- Buffer System: This component provides optimal conditions for enzyme activity, including the right pH and salt concentration. It stabilizes the reaction and enhances the efficiency of DNA amplification.
- dNTPs (Deoxynucleotide Triphosphates): These building blocks are necessary for DNA replication. A balanced mix of dATP, dCTP, dGTP, and dTTP is essential for proper DNA synthesis.
- Primers: Short sequences of nucleotides that are designed to match specific regions of the target DNA. They initiate the DNA synthesis process and are critical for ensuring specificity in qPCR.
Each of these reagents plays a unique role in the performance of the qPCR process. Their careful selection and validation help ensure that the reactions are sensitive, reproducible, and specific to the target nucleic acids.
Master Mix Preparation
Master mix preparation is a crucial step in the workflow of SYBR Green qPCR. It combines all the necessary reagents into a single solution. This approach streamlines the setup process and reduces the risk of pipetting errors. To prepare the master mix, follow these steps:
- Calculate the Total Volume: Determine the number of samples and replicate reactions. Multiply the individual reaction volume by this number along with an additional 10% volume to account for pipetting losses.
- Combine Reagents: In a clean tube, gently mix:
- Mix Thoroughly: Avoid generating bubbles. Use a pipette to gently swirl the contents, ensuring homogeneity.
- Aliquot the Master Mix: Dispense the prepared master mix into each PCR tube, ensuring equal distribution. This step is essential for reproducibility across all reactions.
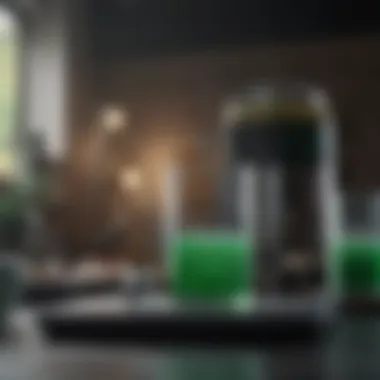
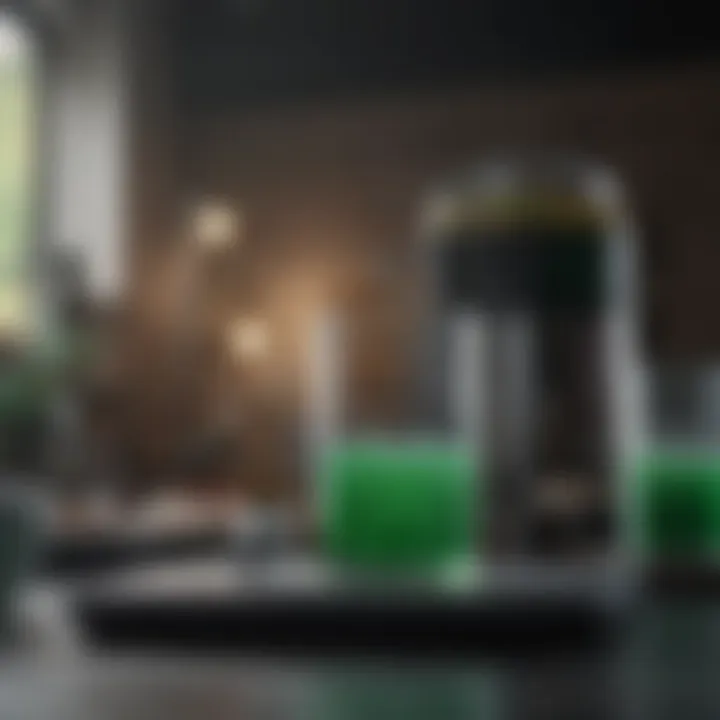
- SYBR Green Master Mix
- Primers (forward and reverse)
- dNTPs if not included in the master mix
- Nuclease-free water to adjust volume
Following these steps allows for efficient preparation of the qPCR reactions. A well-prepared master mix ensures that every sample is treated uniformly, reducing variability and enhancing the reliability of quantitative results. Efficient preparation is critical for achieving reproducible experimental outcomes, which is a cornerstone of scientific research.
Sample Preparation Techniques
Sample preparation is a crucial step in achieving reliable results with SYBR Green qPCR. The accuracy and reproducibility of quantitative measurements largely depend on the integrity and quality of nucleic acids extracted from the biological samples. Improper sample preparation can lead to inconsistent results and interpretation errors, undermining the purpose of the qPCR experiment.
Effective sample preparation techniques ensure that the extracted nucleic acids are free of contaminants that could interfere with the amplification process. These techniques involve meticulous attention to detail, from choosing the right extraction methods to ensuring proper sample handling and storage. Clear protocols will guide researchers in refining these steps to obtain optimal results. Here, we will delve into two essential aspects of sample preparation: nucleic acid extraction and sample dilution methods.
Nucleic Acid Extraction
Nucleic acid extraction forms the foundation of any qPCR analysis. The process captures DNA or RNA from various sources like cells, tissues, or environmental samples. Several methods exist, including organic solvent extraction, silica-based column purification, and magnetic beads. Each method has unique advantages and might be more suitable for specific sample types.
When selecting an extraction method, several factors should be considered:
- Sample Type: Different biological materials may require tailored approaches to maximizing yield.
- Integrity of the Nucleic Acid: The extraction method should preserve the quality of the nucleic acids.
- Downstream Applications: Some methods may introduce inhibitors that affect qPCR performance.
All choices impact the yield and purity of the nucleic acids, which directly affects the qPCR results. For example, a common method, the Qiagen DNeasy kit, effectively extracts high-quality genomic DNA suitable for qPCR applications. Ensuring that the nucleic acid is of high integrity helps in achieving consistent and reliable amplification signals in subsequent reactions.
Sample Dilution Methods
Appropriate sample dilution is vital in optimizing qPCR results. This process ensures that the concentration of nucleic acids is within the optimal range needed for effective amplification. Too high or too low concentrations can lead to skewed results or failure to detect the target sequence altogether.
Common dilution strategies include:
- Serial Dilutions: systematically reducing sample concentration by transferring equal volumes into new samples, achieving a range of known concentrations.
- Single-Step Dilutions: adding a specified volume of solvent to a target sample to create a desired dilution.
Both methods can serve different purposes, depending on experimental designs. For instance, serial dilutions are particularly useful in establishing standard curves for quantification. A clear understanding of dilution factors helps ensure that data interpretation remains grounded in reliable quantitative assessment.
Proper sample preparation techniques create a robust starting point for successful SYBR Green qPCR experiments.
Setting Up the qPCR Reaction
Setting up the qPCR reaction is a critical step that significantly influences the reliability and accuracy of quantitative results. Properly configured reactions ensure optimal amplification of target nucleic acids while minimizing variability. This phase lays the groundwork for achieving consistent findings across experimental repeats. The significance of each component and parameter in this setup cannot be understated, as they collectively contribute to the performance of the qPCR.
Optimal Primer Design
Efficient primer design is vital for successful qPCR. Primers should be specific to the target sequence, with a balance of melting temperatures (Tm) to promote effective annealing. Ideally, the Tm of both forward and reverse primers should be within 2 degrees Celsius of each other. Keeping amplicon size between 70 to 200 base pairs enhances sensitivity and specificity.
Using software tools to analyze primer potential can help predict secondary structures and potential dimer formations. Avoiding sequences that may bind non-specifically increases the chances of obtaining accurate Ct values. Moreover, validating primer efficiency through standard curve analysis can help determine the quantitative capabilities of the primers used.
Reaction Volume and Conditions
The volume of the qPCR reaction plays a significant role in dilution effects and reagent consumption. Most typical reactions range from 10 to 50 microliters. A smaller reaction volume often results in reduced reagent costs, but might risk inconsistencies in the data. Therefore, a balance is needed to maximize reliability without excessive waste.
Appropriate conditions for qPCR include the selection of ideal reaction temperatures. The denaturation step usually takes place at around 95 degrees Celsius, causing the DNA strands to separate. This is followed by the annealing phase, which typically occurs between 50 to 65 degrees Celsius, allowing primers to bind. Finally, the extension step generally happens at 72 degrees Celsius, where the polymerase synthesizes new DNA strands.
Choosing the right conditions can be the difference between successful amplification and a failed experiment.
To conclude, taking careful consideration during the qPCR reaction setup promotes accurate quantification. Attention to detail in primer design and reaction conditions ensures reproducibility and reliability for experimental outcomes.
Thermal Cycling Parameters
Thermal cycling parameters form the backbone of the Power SYBR Green qPCR protocol. Mastery of these parameters amplifies the accuracy and reliability of nucleic acid quantification. Understanding each element is essential for effective data interpretation and reproducibility.
Denaturation and Annealing Steps
The denaturation step marks the initiation of the qPCR cycle. This process involves heating the reaction mixture to around 95°C, causing the double-stranded DNA to separate into single strands. This step is crucial as it ensures the availability of single-stranded templates for the primers in subsequent reactions. Without proper denaturation, amplification efficiency will be significantly compromised.
Following denaturation, the temperature is lowered to approximately 50-65°C during the annealing phase. In this phase, primers that are designed to flank the target region attach to their complementary sequences on the single-stranded DNA. The precise temperature for annealing is often specific to the primers used. If the temperature is too high, primers may not bind sufficiently, while too low a temperature can lead to non-specific binding. This delicate balance is vital for optimizing qPCR performance. Adjusting the annealing temperature can largely impact the specificity and yield of the results.
"Appropriate denaturation and annealing steps are not just technical requirements; they are key factors influencing the qPCR results."
Extension Phase Considerations
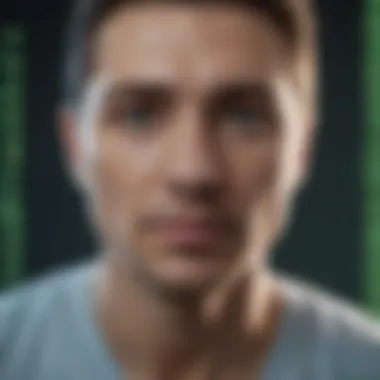
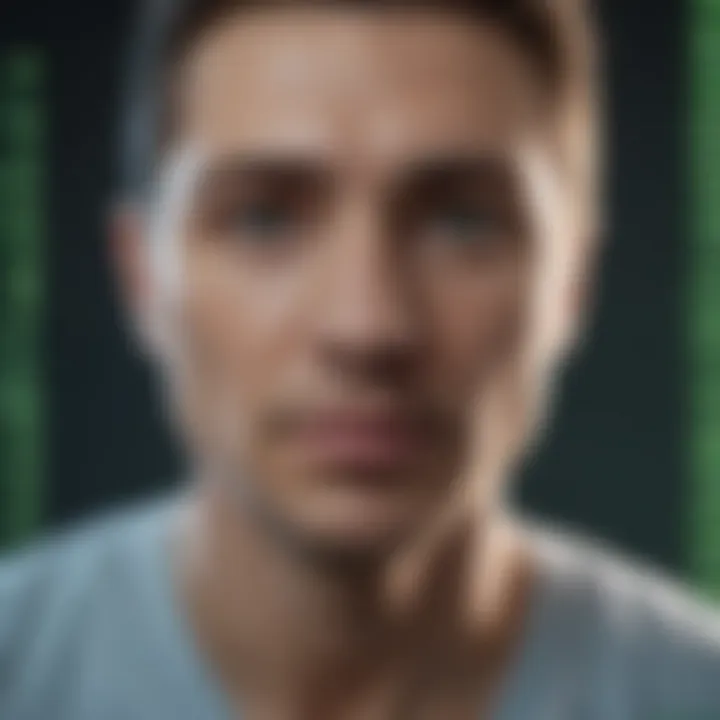
The extension phase follows the successful annealing of primers. Here, the temperature is typically raised to about 72°C, which is optimal for Taq polymerase activity, the enzyme responsible for DNA synthesis. This phase allows the enzyme to add nucleotides to the growing strand, effectively synthesizing a new strand of DNA.
Timing is critical during this step as well. The length of the extension should correspond with the size of the DNA fragment being amplified. Generally, it is recommended to allow one minute of extension per kilobase of target DNA; however, smaller targets may require less time, and excessive time may lead to non-specific products. Monitoring these parameters is necessary to ensure fidelity in the amplification process.
By meticulously adjusting the thermal cycling parameters, researchers significantly increase their chances of achieving reliable qPCR results. Remember that these cycles must be optimized for each individual experiment to get the best data possible.
Data Acquisition and Analysis
Data acquisition and analysis are critical components of the Power SYBR Green qPCR protocol. These processes determine how accurately and reliably the quantitative data regarding nucleic acids are captured and interpreted. Understanding the nuances of data acquisition involves recognizing how the qPCR machine records fluorescence and outputs data concerning amplification. This is crucial, as the quality of data influences the conclusions drawn from experiments.
During the data acquisition phase, the qPCR instrument measures the fluorescence emitted by the SYBR Green dye bound to double-stranded DNA during the extension phase. Accurate data collection ensures that correct cycle threshold (Ct) values can be generated, which is essential for reliable quantification. Failures in this stage may lead to misleading results, affecting downstream analyses and interpretations.
The merits of thorough data analysis can't be overstated. By employing proper quantification methods, researchers can not only document but also validate their findings against established standards. Various methods of quantification, including absolute and relative quantification, provide different perspectives on the same data set, helping to elucidate biological significance. It's wise to always validate assay performance, considering factors like efficiency and specificity to enhance the robustness of conclusions drawn.
Understanding Ct Values
Cycle threshold (Ct) values emerge as pivotal elements in any qPCR analysis. The Ct value represents the number of cycles required for the fluorescent signal to exceed a specified threshold. Lower Ct values suggest higher initial amounts of target nucleic acids in the sample, while higher Ct values indicate lower quantities.
Interpreting Ct values requires caution. Comparison among samples demands uniformity in experimental conditions, as variations can skew results. Factors affecting Ct values include primer efficiency, concentration of nucleic acids, and the quality of reagents. By standardizing these variables, researchers can ensure their Ct values are a true reflection of nucleic acid quantities. This uniformity is essential for achieving robust, reproducible results.
Quantification Methods
Quantification methods employed in the SYBR Green qPCR protocol can be broadly categorized into two approaches: absolute and relative quantification. Each method has its benefits and limitations, influencing the choice based on the experimental goals.
- Absolute Quantification: This method involves creating a standard curve using known concentrations of target DNA. It allows for the precise calculation of the starting quantity in experimental samples. The accuracy of this method hinges on the careful preparation of standards and ideal curve fitting.
- Relative Quantification: This approach assesses the expression of the target gene relative to a reference gene. By normalizing to stable reference genes, researchers can account for variations in sample quality. This method is advantageous for studies examining gene expression under different conditions, as it provides context regarding relative differences between samples.
Troubleshooting Common Issues
In the application of SYBR Green qPCR, researchers frequently encounter challenges that can compromise the integrity and reliability of their results. Understanding and addressing these common issues is crucial for producing accurate and reproducible data. This section delves into two significant problems: low amplification signals and non-specific amplification, offering insights and solutions to mitigate these concerns.
Low Amplification Signals
Low amplification signals can arise due to various factors. When the signal is weak, it may lead to inaccurate quantification of the target nucleic acid. Among the key reasons for low amplification are suboptimal primer design, insufficient template concentration, or poor quality of nucleic acid samples. Each of these elements plays a vital role in the qPCR reaction.
To improve amplification signals, consider the following:
- Check Primer Efficiency: Confirm that the primers are designed to have optimal melting temperatures and specific binding affinities to the target sequence.
- Increase Template Concentration: If the initial amount of template is too low, amplification may not reach threshold levels. Experiment with different input amounts to determine the optimal concentration.
- Evaluate Nucleic Acid Quality: Poor quality nucleic acids can inhibit the polymerase activity, leading to weak signals. Use high-quality extraction methods and test the integrity of your nucleic acids prior to amplification.
Following these guidelines can enhance your chances of achieving robust amplification signals.
Non-Specific Amplification Problems
Non-specific amplification is another prevalent issue that may arise during SYBR Green qPCR experiments. This problem manifests when the qPCR produces unintended products, leading to misleading quantification results. Non-specific amplification can occur due to several reasons, including inappropriate primer pairs, contamination, or incorrect annealing temperatures.
To address non-specific amplification, the following approaches may be useful:
- Optimize Primer Design: Reassess the primer sequences to ensure they are specific to the target region. Avoid designing primers that can anneal to multiple regions within the genome or homologous genes.
- Adjust Annealing Temperature: Elevating the annealing temperature can reduce non-specific binding. Perform a gradient PCR to find the optimal temperature for primer binding.
- Implement Contamination Controls: Always include no-template controls to help identify potential contamination. Additionally, consider using dedicated pipettes and sterile techniques when preparing reagents.
By following these troubleshooting steps, researchers can enhance the reliability of their SYBR Green qPCR results and achieve accurate quantification.
Troubleshooting is a critical aspect of ensuring the success of experiments and should not be overlooked.
Being aware of these common issues and applying systematic approaches can significantly enhance the quality of your research outcomes.
Applications of SYBR Green qPCR
The versatility of SYBR Green qPCR has cemented its role in various fields within molecular biology. Understanding these applications is crucial for researchers who wish to leverage its capabilities for precise nucleic acid quantification. This section will dissect two prominent uses of SYBR Green qPCR: gene expression analysis and pathogen detection. Both applications highlight the method's reliability and efficiency, catering to diverse research needs.
Gene Expression Analysis
Gene expression analysis is a core application of SYBR Green qPCR. This technique allows researchers to quantify the expression levels of specific genes in a given sample. By using SYBR Green, scientists can monitor double-stranded DNA formation during the amplification process. This is essential for understanding cellular responses in various conditions, such as disease states or environmental changes.
- Sensitivity and Specificity: SYBR Green qPCR provides high sensitivity, enabling the detection of low-abundance transcripts. It is also advantageous due to its capability to assay multiple samples simultaneously, saving time and resources.
- Real-Time Monitoring: The real-time quantification aspect means that results can be obtained in a timely manner. Researchers can analyze gene expression patterns across different samples, crucial for experiments involving time-course studies or responses to treatments.
- Standardization: The method allows for the use of reference genes for normalization. This standardization ensures that variations in sample input are accounted for, thus increasing the reliability of comparative analyses.
- Applications in Research: From studying cancer to developmental biology, SYBR Green qPCR is applied in various research areas to elucidate gene regulation, identify biomarkers, and explore therapeutic targets.
Pathogen Detection
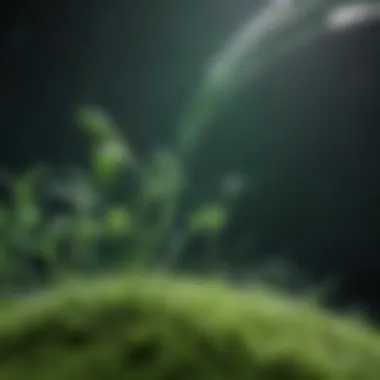
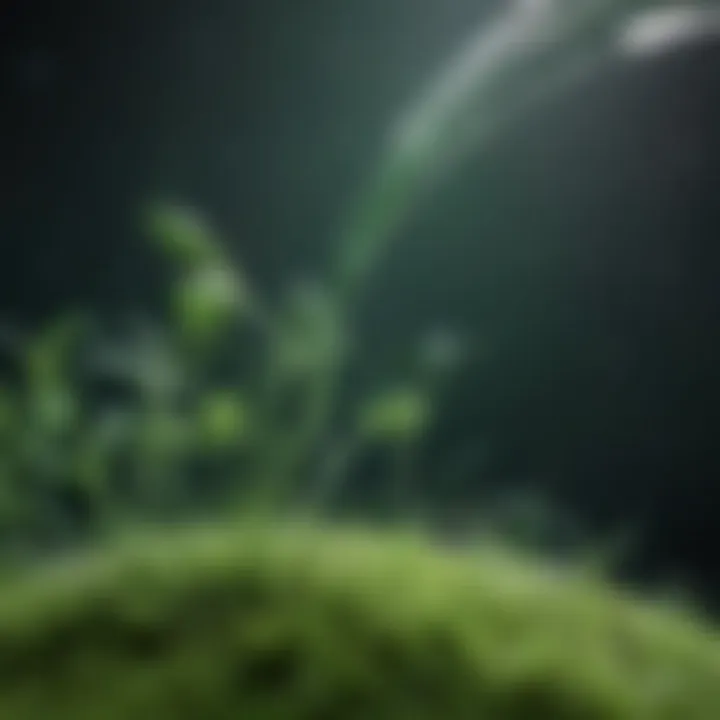
Pathogen detection is another prominent application where SYBR Green qPCR demonstrates its efficacy. With the rise of infectious diseases, rapid and accurate detection of pathogens is of great significance. This method facilitates the identification of specific DNA sequences associated with bacterial or viral infections, offering timely results crucial for diagnosis and treatment.
- Rapid Results: SYBR Green qPCR significantly reduces the time required for pathogen detection compared to traditional culture methods. In clinical settings, this speed can lead to better patient outcomes through quicker treatment decisions.
- Broad Application Spectrum: This technique can be used to detect a wide range of pathogens, including bacteria and viruses. It is commonly utilized in fields like food safety, environmental monitoring, and clinical diagnostics.
- Cost-Effectiveness: Compared to other detection methods, SYBR Green qPCR can be less expensive, making it accessible for many labs seeking to implement pathogen surveillance strategies.
The reliability of SYBR Green qPCR in detecting nucleic acids has transformed diagnostic approaches, reinforcing its role in public health and safety.
In summary, the applications of SYBR Green qPCR extend beyond gene expression analysis to crucial areas like pathogen detection. The importance of understanding these applications cannot be overstated, as they provide significant insight into biological processes and enable informed decisions in healthcare and research contexts.
Comparison with Alternative qPCR Methods
When discussing quantitative PCR (qPCR), it is crucial to assess the available methodologies. Each method has its own strengths and challenges, and understanding these differences is essential for researchers seeking accurate nucleic acid quantification. This comparison will focus on the Power SYBR Green qPCR technology in relation to TaqMan and digital PCR approaches. Each of these techniques has unique features that can influence experimental outcomes, and thus understanding them can greatly impact research effectiveness.
TaqMan vs. SYBR Green
TaqMan probes, unlike SYBR Green, employ specific fluorescent probes designed for the target sequences. During amplification, the probe hybridizes to the target DNA, and when Taq polymerase cleaves the probe, it emits a distinct signal. This specificity minimizes the likelihood of non-specific amplification, leading to more reliable quantification under certain circumstances.
On the other hand, SYBR Green binds to any double-stranded DNA. Therefore, it can be less specific than TaqMan. However, SYBR Green's advantages include lower costs and ease of use. It also permits multiplexing, where multiple targets can be quantified in a single reaction. This can streamline workflows, especially when analyzing similar or related genes.
While TaqMan offers enhanced specificity, it requires additional design for each probe, potentially increasing experimentation time. Overall, the choice between TaqMan and SYBR Green should depend on the research objectives, budget, and the complexity of the experimental design.
Digital PCR Approaches
Digital PCR (dPCR) is an emerging technology that provides absolute quantification of nucleic acids. Unlike conventional qPCR methods, which rely on quantifying the increase in fluorescence signal, dPCR partitions the sample into numerous individual reactions. This results in a simpler count of the number of positive amplifications.
One significant advantage of digital PCR is its robustness against variations, making it highly suitable for applications requiring extreme precision, such as rare variant detection or copy number variation analysis. However, it is generally more expensive and requires advanced instrumentation compared to SYBR Green qPCR.
Moreover, while dPCR is precise, it is less suited for high-throughput applications when compared to SYBR Green, which allows rapid processing of many samples with ease.
Performing a comparison between SYBR Green qPCR and digital PCR aids researchers in selecting the best approach for their specific applications. The choice ultimately revolves around factors such as sensitivity, throughput, and budget availability.
Future Directions in qPCR Technology
The landscape of quantitative polymerase chain reaction (qPCR) is continually evolving. Future directions in qPCR technology are crucial, especially as researchers seek more efficient and accurate methods for nucleic acid quantification. The advancements in this area promise to enhance the sensitivity and specificity of qPCR assays, catering to the diverse needs across various fields including diagnostics, research, and therapeutics. With the demands of modern science increasing, it becomes necessary to not only understand these trends but also to adopt them in practice to stay relevant and effective.
Emerging Trends in Quantification
One notable emergent trend is the integration of advanced data analysis techniques. Traditional qPCR typically relies on relative quantification, which can introduce variability based on reference gene selection. However, newer methods are leveraging absolute quantification strategies through advancements in software algorithms and statistical models. This shift ensures more reliable data interpretation and reproducibility, especially when comparing results between laboratories.
Another trend is the development of high-throughput qPCR systems. These systems enable the simultaneous analysis of multiple samples, reducing time and labor costs. This capability is essential for large-scale studies or clinical applications where hundreds of samples need to be processed quickly.
Moreover, real-time PCR machines are now incorporating more sophisticated optical systems that enhance signal detection. These technologies promise improved limit of detection (LOD) and allow for better differentiation of specific signals from background noise.
Key Points to Consider:
- Shift towards absolute quantification for enhanced accuracy.
- High-throughput systems to save time in processing multiple samples.
- Improved optical systems for enhanced sensitivity and specificity.
Integration with Next-Gen Sequencing
The integration of qPCR with next-generation sequencing (NGS) is redefining the approaches to nucleic acid quantification. The coupling of these powerful techniques provides a comprehensive understanding of gene expression and variation. NGS allows for unmatched sequencing depth and breadth, while qPCR offers rapid quantification of specific target sequences.
This integrative approach enables researchers to validate NGS findings through targeted assays, ensuring that the data output is both reliable and interpretable. Furthermore, using SYBR Green qPCR in conjunction with NGS presents opportunities for multiplexing, where several targets can be analyzed simultaneously. This capability reduces time and resource expenditure, which is particularly advantageous in clinical settings where rapid results are essential.
In the realm of molecular biology, the fusion of qPCR with next-generation sequencing technology marks a significant leap forward in both accuracy and efficiency of nucleic acid analysis.
End and Recommendations
In the realm of molecular biology, the Power SYBR Green qPCR protocol stands out as a crucial tool for accurate nucleic acid quantification. This technique not only aids in research but also drives advances in diagnostic applications. As we conclude this guide, it is essential to underscore the key elements and considerations necessary for the effective implementation of this protocol.
Key Takeaways
The following insights encapsulate the essence of the findings throughout this comprehensive guide:
- Reproducibility: Consistency in results is paramount. Preparing master mixes correctly and maintaining standard conditions across experiments are crucial steps that influence the integrity of data.
- Practical Applications: The usage of SYBR Green qPCR is broad, ranging from gene expression studies to pathogen detection, making it a versatile tool in various fields.
- Optimization: Proper primer design and precise thermal cycling parameters directly impact amplification efficiency and specificity. Rigorous testing of conditions is advised to fine-tune the protocol to specific needs.
- Error Mitigation: Awareness of common pitfalls, such as non-specific amplifications and low signal outputs, empowers researchers to troubleshoot effectively, thus enhancing the reliability of the results.
- Integration with Technologies: As discussed, integrating SYBR Green qPCR with next-generation sequencing opens new avenues for research, enabling finer resolution in genomic studies.
Best Practices for Implementation
To ensure that the Power SYBR Green qPCR technique yields accurate and reliable results, researchers should adhere to the following best practices:
- Standardization: Maintain strict standard protocols for reagents and dilutions to minimize variability across experiments.
- Quality Control: Regularly assess the quality of nucleic acid extracts. Contaminants can significantly affect amplification outcomes.
- Careful Primer Selection: Choose primers with high specificity and efficiency. Employ tools like Primer3 or similar for optimal design parameters.
- Thermal Cycler Calibration: Ensure that the thermal cyclers are regularly calibrated for accurate temperature readings, as fluctuations can lead to inconsistencies.
- Data Analysis Consistency: Use the same methods for data analysis across experiments. A clear understanding of Ct values and relative quantification should be prioritized.
- Documentation: Keep thorough records of experimental conditions, reactions, and outcomes. This practice helps in troubleshooting issues and refining future experiments.
These recommendations help bridge the gap between theoretical knowledge and practical application, ensuring successful outcomes in molecular experiments.