Impact of Light on Water Quality and Ecosystems
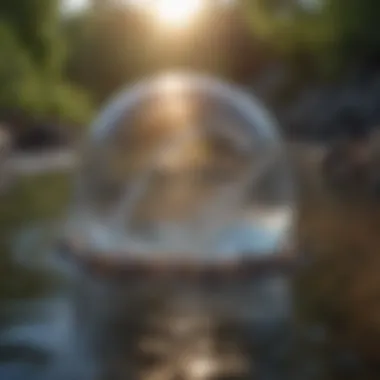
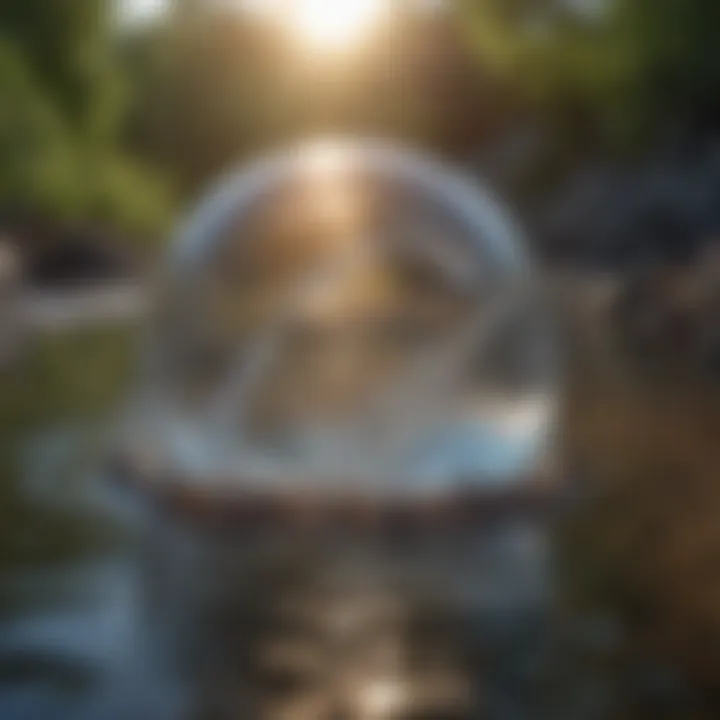
Intro
Understanding how various light sources impact water quality is like opening a Pandora's box – what’s inside might surprise you. Light, often taken for granted in daily life, plays a critical role not only for vision but also in driving essential biological processes in aquatic ecosystems. This interrelation between light wavelengths and water characteristics is a nuanced realm of study, bringing forth profound implications for fields such as ecology, **aqua- culture, and even environmental conservation.
The effect of light on water quality can be viewed from many angles. Do you know why certain fish thrive in clear waters with abundant sunlight, while others prefer the shadowy depths? This variability highlights the importance of light in determining ecological balance and integrity. Various types of light sources – whether natural sunlight or artificial LEDs – interact with water's chemical and biological properties differently. Some wavelengths may promote the growth of beneficial algae, while others can exacerbate harmful bloom scenarios or even degrade water quality through photochemical reactions. With this complexity in mind, let’s dive deeper into the broader research topic.
Overview of Research Topic
Brief Background and Context
The journey to understand the interaction between light and water quality is both ancient and evolving. Historically, scientists have recognized that water is more than a simple solvent; it is a dynamic environment where energy, nutrients, and organisms coexist and interact.
Recent studies have illuminated specific aspects of this relationship. For instance, researchers have discovered that ultraviolet (UV) light can significantly alter the chemical composition of water. In freshwater environments, this can affect nutrient cycling and the biological community structure.
Key facets of this exploration include:
- The effect of different light intensities on photosynthesis in aquatic plants.
- The influence of light spectra on microbial activity and their role in ecosystems.
- How light alters water chemistry, including pH and dissolved oxygen levels.
Importance in Current Scientific Landscape
As scientists face pressing issues like climate change, pollution, and biodiversity loss, understanding these interactions becomes critical. The ability to manage water quality through strategic light source application can have widespread implications.
For example, in aquaculture, optimizing lighting conditions can enhance fish growth and feeding efficiency, which leads to better yield in a sustainable manner. Furthermore, artificial lighting is being harnessed to mitigate harmful algal blooms by adjusting exposure times and intensities.
"By tapping into the natural synergies between light and water quality, we can create adaptive strategies for conservation and management that reflect the realities of environmental changes."
So, as we journey through this topic, keep an eye on how light sources do not merely illuminate our world but actively shape the aquatic communities that reside within it.
Methodology
Research Design and Approach
The exploration of light and water quality demands a careful and methodical approach. A mix of laboratory experiments and field studies is typically employed. The use of controlled environments helps isolate variables, while real-world studies offer practical insights.
Data Collection Techniques
To gather relevant data, researchers may utilize:
- Spectrophotometry to measure light absorption and transmission in water.
- Water sampling to assess chemical and biological parameters before and after light exposure.
- Remote sensing technologies for large-scale analysis of water bodies, enabling them to track changes in water quality over time related to light conditions.
Each method brings its strengths and weaknesses, and together they form a comprehensive framework for understanding the intricate dance between light and water quality.
Prelude to Light Sources in Aquatic Environments
In examining aquatic systems, the role of light cannot be overemphasized. Light sources are fundamental to understanding aquatic environments, as they drive photosynthetic processes and influence the overall quality and ecology of water bodies. This section sets the stage for exploring how light interacts with water, impacting both the biotic and abiotic components essential for a balanced ecosystem.
Definition of Light Sources
Light sources, broadly speaking, are any origins of light that can penetrate aquatic environments. These sources can be classified into different categories, primarily natural and artificial. Understanding these distinctions is crucial because the type of light present can drastically alter water chemical properties and aquatic life behaviors.
Types of Light Sources
Both natural and artificial light sources have distinct characteristics and functions. They play vital roles in shaping the conditions within aquatic environments.
Natural Light
Natural light primarily refers to sunlight, which filters through atmosphere and into water bodies. One major benefit of natural light is its ability to support photosynthesis in aquatic plants, algae, and phytoplankton. This process is essential for oxygen production and serves as the foundation of the aquatic food web.
A key characteristic of natural light is its variability throughout the day; sunlight intensity changes with cloud cover, season, and time of day. Such variability can create a dynamic environment influencing species distribution and growth.
Advantages:
- Supports photosynthesis and oxygen production.
- Fosters a balanced ecosystem by providing energy at different times of the day.
Disadvantages:
- Limited by geographic location and seasonal changes, which can create fluctuations detrimental to sensitive species.
Artificial Light
Artificial light, created by human-made sources such as LED lamps and fluorescent lights, is used increasingly in controlled environments like aquaculture. Its main contribution is the possibility to simulate day/night cycles, which assists in optimizing growth and enhances productivity among aquatic species. A key characteristic of artificial light is its controllability; light intensity and spectrum can be adjusted according to specific requirements, offering significant advantages in research and commercial settings.
Advantages:
- Can be customized to suit particular species' needs.
- Enhances growth rates in aquaculture settings, enabling improved yields.
Disadvantages:
- Can disrupt natural circadian rhythms of aquatic organisms, potentially leading to stress or abnormal behaviors.
UV Light
Ultraviolet (UV) light, although invisible to the naked eye, holds a specialized role in managing water quality. It is primarily employed in disinfection processes, targeting pathogens and helping maintain hygiene in both aquaculture and public water supplies. The ability of UV light to deactivate microorganisms contributes directly to the improvement of water quality. A key characteristic of UV light is its short wavelength, which means it does not penetrate water as deeply as visible light but has potent effects at the surface level.
Advantages:
- Highly effective in water treatment, ensuring safe aquatic environments.
- Prevents the growth of harmful bacteria and viruses, critical for aquaculture health.
Disadvantages:
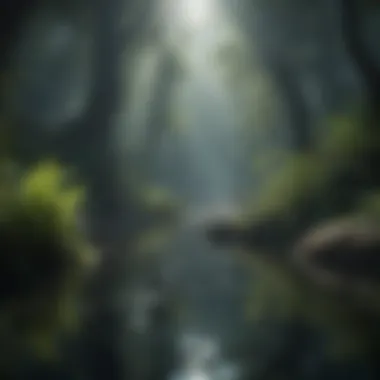
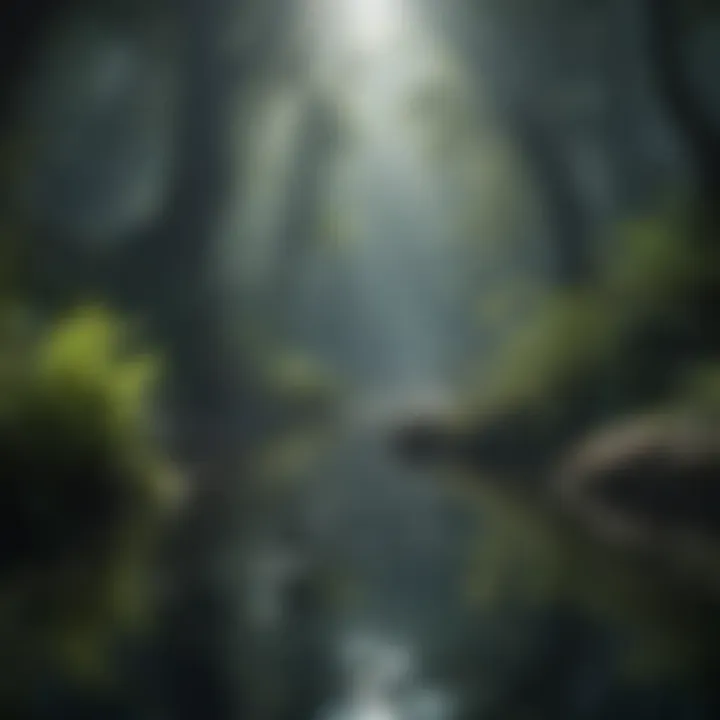
- Limited penetration depth in water limits its effectiveness in larger bodies unless used in conjunction with other treatment methods.
"The interaction between different light sources and aquatic systems is a rich and complex tapestry that forms the foundation of many ecological studies."
In summary, understanding the variety of light sources and their implications is essential for anyone involved in aquatic research or management. As we dive deeper into this article, we will explore these concepts further, looking more closely at how these light sources impact aquatic biology and chemistry.
The Role of Light in Aquatic Photosynthesis
Light plays an indispensable role in aquatic ecosystems, participating directly in the process of photosynthesis, which is fundamental to life in water. Understanding this relationship is vital for grasping how light influences not just the growth of aquatic plants but also the larger framework of food webs within these environments. The interplay between light and photosynthesis is a critical subject in various scientific spheres including ecology, environmental science, and aquaculture. By shedding light on how different light sources affect this biological process, we can better understand their implications for water quality and aquatic health.
Photosynthetic Processes in Aquatic Plants
Photosynthesis in aquatic plants occurs primarily in the upper layers of water where light penetration is strongest. This process involves chlorophyll pigments in plant cells capturing light energy, which then transforms carbon dioxide and water into glucose and oxygen. This transformation isn't just a neat trick; it drives the whole ecosystem. For instance, in a freshwater lake, submerged plants like Elodea can photosynthesize efficiently during daylight, contributing significantly to the oxygen content in the water. This oxygen is essential for the survival of fish and other aquatic organisms.
Moreover, aquatic photosynthesis helps regulate carbon levels in the water, mitigating excessive carbon dioxide that could lead to problems such as acidification. While algae, often seen as nuisances, can dominate in certain conditions, they also play an essential role in aquatic photosynthetic processes. When conditions are right, these organisms multiply and contribute dramatically to the region’s primary productivity—all of which depends heavily on available light.
Impact of Light Intensity on Photosynthesis
The intensity of light absolutely matters. In shallower waters, where sunlight is more abundant, aquatic plants often thrive. But if light intensity is too low, photosynthesis slows, stunting plant growth. Each species has its own optimal light intensity, which is crucial for its ability to photosynthesize efficiently. For example, seagrasses, which are vital in coastal ecosystems, require moderate light levels to flourish. Conversely, some algae can tolerate lower light intensities but may suffer when exposure is too high.
Factors like turbidity—cloudiness in the water caused by sediment or phytoplankton—can also limit light penetration. As a result, areas with high turbidity may experience decreased photosynthetic rates. The outcome can cascade through the ecosystem, affecting not only plant growth but also herbivores and, consequently, the predators that rely on them. Thus, monitoring light availability becomes essential for maintaining healthy aquatic habitats.
In summary, the role of light in aquatic photosynthesis is multifaceted, influencing not just plant growth but the entire ecosystem's health and water quality. Understanding these dynamics enables better management strategies in areas like aquaculture and conservation, ensuring that aquatic systems thrive under the right light conditions.
Light Wavelengths and Their Effects on Water Chemistry
The relationship between light wavelengths and water chemistry is pivotal in understanding how aquatic environments function. Different wavelengths of light carry varying energies, and their absorption influences a spectrum of chemical reactions within water. Not only do these reactions play a significant role in the health of aquatic ecosystems, but they also affect water treatment strategies and overall water quality. Recognizing the effects of light wavelengths is critical for scientists and industry professionals aiming to design effective water management practices.
Absorption Spectra of Water
Water possesses unique optical properties, which are determined by its molecular structure and the wavelength of light. Each wavelength interacts differently when it encounters water, leading to distinct absorption spectra. Not surprisingly, water's absorption peaks occur predominantly in the ultraviolet and infrared ranges, leaving a narrow band within the visible spectrum that is crucial for photosynthetic activity.
- Ultraviolet Light: Water strongly absorbs UV light, thus protecting marine life from harmful radiation. However, the energy from UV can drive some chemical reactions, aiding in the breakdown of organic pollutants.
- Visible Light: The blue-green spectrum penetrates water more effectively, allowing photosynthesis to occur at significant depths. This aspect is essential since it affects primary production by aquatic plants and phytoplankton, forming the base of the aquatic food web.
- Infrared Light: While mainly absorbed as heat, infrared radiation can influence the thermal dynamics of water bodies, thereby affecting the solubility of gases and the reaction rates of various chemical processes.
Understanding these absorption characteristics allows researchers and environmentalists to monitor and model aquatic systems more effectively.
Influence of Light Wavelengths on Chemical Reactions
The wavelength of light doesn’t just dictate absorption; it also has profound implications on chemical reactions in water. Different wavelengths can catalyze specific reactions, influencing everything from nutrient availability to pollutant degradation.
- Nutrient Cycling: Light promotes the transformation of nutrients. For example, ultraviolet light plays a role in the photolysis of certain compounds, such as nitrogen species, thus enhancing nitrogen cycling in aquatic systems.
- Pollutant Degradation: Light-driven processes such as photocatalysis can utilize specific wavelengths to break down harmful contaminants in water. The efficacy of these reactions often depends on the intensity and type of light source.
- Oxygen Production: In photosynthesis, the absorption of specific wavelengths by chlorophyll in aquatic plants leads to oxygen production, which is vital for aquatic organisms. The inefficiencies or variations in light availability can directly affect the oxygen levels in water, impacting aquatic life.
- Strategic illumination can, therefore, support nutrient cycling, contributing to healthier ecosystems.
It’s crucial to note that harmonizing artificial lighting to optimize these wavelengths can significantly enhance water quality management in contexts like aquaculture and wastewater treatment.
In summary, the absorption spectra of water and the influence of light wavelengths on chemical reactions are indispensable topics in aquatic science. A deeper understanding of these phenomena can provide insights that help improve water management techniques and ensure the sustainability of aquatic ecosystems.
Biological Implications of Light in Aquatic Systems
Understanding the biological implications of light in aquatic systems is crucial for several reasons. Light, as an energy source, directly impacts photosynthesis, which forms the foundation of food webs in marine environments. Furthermore, organisms in these systems, from microscopic phytoplankton to large marine mammals, rely on light for their biological rhythms and ecological interactions. This section delves into two key subtopics: light and marine ecosystems, as well as how variations in photoperiod affect these delicate interactions.
Light and Marine Ecosystems
Marine ecosystems are like a well-oiled machine, with each cog playing a vital role in maintaining balance. Light is the driving force behind this dynamism. It allows for photosynthetic organisms to thrive, providing oxygen and sustenance that supports a myriad of other marine organisms. In essence, without light, much of life in our oceans would simply grind to a halt.
Here are some critical points to consider regarding this relationship:
- Photosynthetic Dependence: Phytoplankton, the backbone of the ocean food chain, use sunlight to produce energy. Their productivity is directly linked to the amount and quality of light available.
- Habitat Specification: Different marine environments, like coral reefs and deep-sea ecosystems, have distinct light conditions that cater to specific organisms. For instance, corals have symbiotic relationships with zooxanthellae, algae that require light to photosynthesize.
- Biodiversity: Regions with abundant light see a greater diversity of life. This includes not just plants but also herbivores, carnivores, and decomposers, each occupying a unique niche and contributing to the ecosystem's structure.
In focusing on light, researchers can better understand potential shifts in marine biodiversity prompted by climate change or pollution. Any disruption to this light-organism interconnectedness can have cascading effects on the entire system.
Photoperiod Variation and Its Effects
Photoperiod, the duration of light and darkness in a 24-hour cycle, plays an integral role in the biological rhythms of aquatic organisms. Various species have adapted specific behavioral and physiological responses to changes in photoperiod, ensuring their survival and adaptability in fluctuating environments.
Consider these notable effects:
- Reproductive Cycles: Many fish and invertebrates time their spawning activities to coincide with certain light conditions. For example, some species synchronize their mating rituals with lunar cycles, which influence both light intensity and duration.
- Feeding Behavior: Marine animals often alter their feeding patterns based on the time of day. Nocturnal species may dive deeper during daylight, while diurnal animals will be more active during light hours.
- Growth Rates: Enhanced light during longer days can stimulate growth in aquatic plants. Conversely, extended darkness can lead to stunted growth or even die-offs in sensitive species.
"It's crucial for managers and researchers to recognize how changes in light exposure can directly alter ecological balance."
The implications here are substantial, especially in light of human impacts like light pollution. As cities expand and artificial lights proliferate, we may inadvertently disrupt traditional behavioral patterns in marine life.
In summary, light is not merely a backdrop in aquatic systems; it is a pivotal element that dictates biological processes. By deepening our understanding of how light influences marine ecosystems and how organisms adapt to photoperiod variations, we can better protect these vital systems. This knowledge serves as a powerful tool for researchers and policymakers aiming to ensure the sustainability of aquatic life.
Artificial Lighting in Aquaculture
Artificial lighting plays a crucial role in aquaculture, aligning closely with the aims of maintaining optimal environments for aquatic life. This section delves into how different lighting technologies contribute to the health and productivity of fish and plant species in aquaculture systems. Understanding these elements can lead to significant benefits like enhanced growth rates, better feed conversion efficiency, and overall improved water quality management.
Types of Artificial Lighting Used
When it comes to aquaculture, various types of artificial lighting technology can be employed. Each type comes with its own specifications and impacts:
- LED (Light Emitting Diodes): This technology is becoming increasingly popular due to its energy efficiency and long lifespan. LEDs emit specific light wavelengths that can enhance growth and reproduction in aquaculture species. They can be tailored for specific aquatic environments, which allows for a more controlled approach.
- Lamps with High-Pressure Sodium (HPS): These lamps are common in larger aquaculture systems. HPS provides a high output of light but can be less energy-efficient than LEDs. They are beneficial for cultivating certain types of aquatic plants and species that require intense light.
- Fluorescent Lights: Traditionally used in fish farming, fluorescent lights offer a broad spectrum of light, making them a suitable choice for various environments. They are effective in promoting visual comfort among aquatic species, which is instrumental for stress reduction.
Understanding the specifics of each type allows aquaculturists to choose the best option for their individual systems.
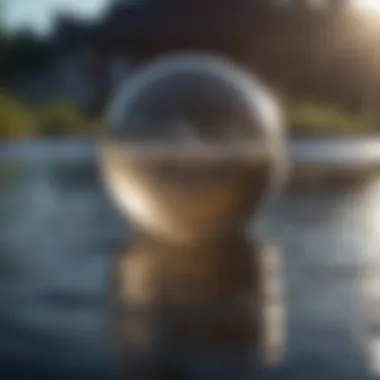
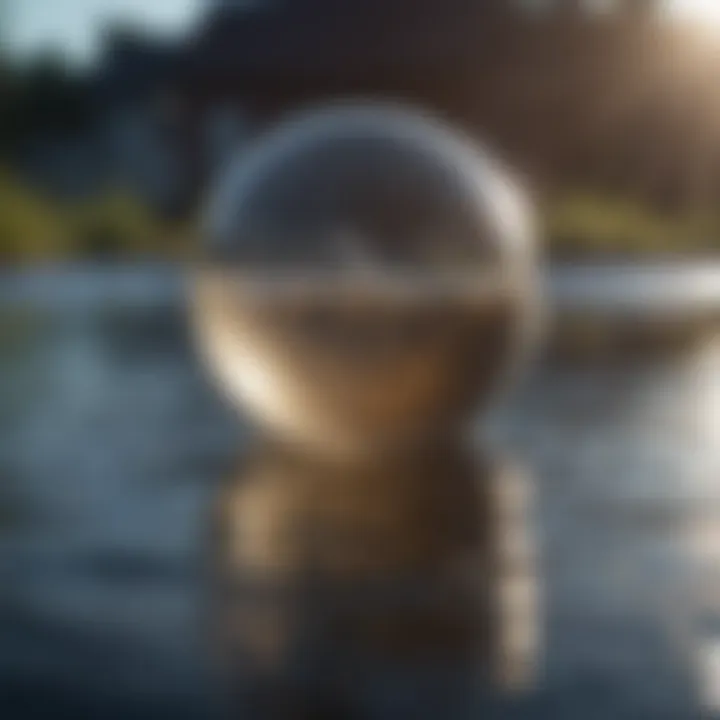
Optimizing Growth in Aquatic Species
Optimizing the growth of aquatic species hinges on effective use of artificial lighting. Proper lighting techniques can lead to improved growth rates, health, and yield. Here are some key strategies:
- Tailored Spectra: The specific wavelengths of light can stimulate photosynthesis in aquatic plants or enhance the growth of fish. For instance, blue light is crucial for photosynthetic processes, while red light can accelerate growth and reproduction.
- Photoperiod Management: Balancing light periods and darkness is essential. For many species, a day length of around 12-16 hours is ideal. Manipulating this photoperiod can result in better spawning and growth.
- Integration with Nutritional Programming: Lighting conditions should be synchronized with feeding schedules. Adjusting light intensity during feeding times helps encourage greater feed uptake.
"Appropriate lighting is not just an accessory; it’s a cornerstone of success in aquaculture fostering better growth and health of aquatic species."
Light-Dependent Reactions in Water Treatment
Light-dependent reactions are crucial in the realm of water treatment. These processes harness energy from environmental light sources to drive essential reactions that can lead to the purification of water. By utilizing light effectively, various technologies are able to address water quality issues, making it cleaner and safer for consumption and use.
One of the key benefits of incorporating light-dependent reactions in water treatment systems is their effectiveness in breaking down harmful contaminants. This technology can be much more sustainable than traditional methods, which often require high levels of energy input and complex chemical treatments. Moreover, employing light to facilitate reactions is compatible with natural processes occurring in various ecosystems, enhancing not only the efficiency of purification but also ensuring minimal ecological disruption.
Photocatalysis in Water Purification
Photocatalysis is a prime example of how light can be utilized for effective water purification. It involves using a catalyst that can absorb light to initiate chemical reactions. When UV light, for instance, strikes a photocatalyst like titanium dioxide, it activates the material, leading to the breakdown of organic pollutants and microorganisms in water.
The process can effectively reduce toxic compounds, pesticides, and even some heavy metals in water. As a result, photocatalysts can serve a dual purpose: breaking down pollutants while simultaneously generating cleaner water. However, there are important considerations to keep in mind. Optimal light intensity and wavelength are necessary for maximizing the efficiency of photocatalytic reactions. Additionally, the stability of photocatalysts over long-term usage affects practical application.
Some benefits of photocatalysis include:
- Low energy requirements compared to other treatment methods.
- Continuous operation capability when exposed to light.
- The potential for minimal by-products, leading to fewer secondary pollutants.
Solar Disinfection Techniques
Solar disinfection, commonly referred to as SODIS, is another fascinating method that employs light to treat water. This technique uses solar energy to eliminate pathogens in contaminated water primarily through ultraviolet radiation. In simple terms, when water is exposed to sunlight in transparent containers, the UV rays effectively kill bacteria and viruses, thus enhancing water quality.
This method is especially beneficial in regions with limited access to advanced water treatment infrastructure. It’s cost-effective and doesn’t require extensive equipment or energy consumption, making it an appealing option for rural communities around the globe.
Here are some advantages of solar disinfection:
- Simplicity of implementation, requiring only sunlight and clear containers.
- Increased public health through easier access to safe drinking water.
- Minimal environmental impact as it utilizes a renewable energy source.
However, like any treatment method, solar disinfection does bear limitations. It is heavily reliant on weather conditions, and treatment times may vary significantly based on sunlight intensity and the clarity of the water. As a result, it might not be suitable as a standalone treatment in some situations.
In summary, light-dependent reactions hold substantial potential for revolutionizing water treatment processes. Both photocatalysis and solar disinfection techniques demonstrate the relationship between light and water quality, indicating that with continued research and development, these methods could become integral components of sustainable water management practices.
Ecological Considerations of Light Pollution
Light pollution is an increasingly pressing environmental challenge that affects aquatic ecosystems and their inhabitants. It refers to excessive, misdirected, or obtrusive artificial light, which can alter natural behavioral patterns and disrupt delicate ecological balances. In the context of water quality, understanding the implications of light pollution is crucial, as it can influence both the chemical and biological characteristics of water bodies.
When discussing light pollution, one must consider how it interferes with the natural rhythms of aquatic life. Most aquatic fauna, such as fish and invertebrates, have adapted to specific light cycles, which govern their migratory patterns, breeding behaviors, and feeding routines. When artificial light permeates these environments, it can lead to confusion among species. For instance, many fish spawn at night. If bright lights are present, they may not find the right cues to reproduce successfully, which can hinder population growth in the long term.
Effects on Aquatic Fauna
- Behavioral Disruption: Fish and other aquatic organisms often rely on natural light cycles for essential behaviors. Studies show that excessive light can cause nocturnal species to misinterpret day and night patterns, disrupting their essential foraging and breeding activities.
- Predation Risk: Predators, like larger fish or birds, utilize light diffusion to hunt smaller prey. With increased light pollution, prey species find themselves more exposed, leading to higher mortality rates.
- Altered Habitat Use: Many species exhibit site fidelity, relying on dark habitats for refuge. Increased illumination can drive these species away from their natural habitats, reducing their populations and biodiversity.
Mitigation Strategies
Efforts to combat light pollution are gaining momentum, with strategies aimed at reducing its adverse effects on aquatic environments:
- Implementing Shielded Lighting: Using fixtures that direct light downward can significantly reduce the amount of misdirected light entering aquatic ecosystems.
- Promoting Natural Light Alternatives: Encouraging the use of warm-colored LEDs and lower-intensity lighting can mitigate adverse impacts on wildlife by more closely mimicking natural moonlight.
- Regulating Lighting Hours: Establishing regulations regarding artificial lighting in sensitive ecological zones can help maintain natural light cycles. This includes timed lighting systems or complete shut-off during certain hours.
Investigating Light and Water Nexus in Research
The interrelationship between light and water has become a focal point for scientific inquiry. Understanding how different light sources impact water quality and aquatic ecosystems is essential for addressing environmental challenges and improving water management practices. With advancements in technology, the exploration of this nexus has broadened, allowing researchers to delve deeper into the effects of light variation on biological processes, chemical reactions, and ecological balance.
Through rigorous investigation, scientists are uncovering how light influences photosynthesis, nutrient cycling, and even the presence of harmful contaminants in aquatic environments. This knowledge is not just academic; it has practical applications that can inform policy and enhance our methods for water treatment and conservation efforts.
Key benefits of researching the light-water relationship include:
- Enhanced understanding of aquatic ecosystems
- Development of improved water treatment techniques
- Identification of sustainable practices in aquaculture
- Informed policies regarding light pollution and its impacts
Such research is critical, as it provides a framework to not only assess but also mitigate the adverse effects of human activities on water systems.
Current Research Methodologies
When examining the nexus between light and water, various research methodologies come into play. These methodologies range from laboratory experiments to field studies, each serving its unique purpose in understanding complex interactions.
- Spectroscopy: This technique evaluates how different wavelengths of light interact with water samples, allowing researchers to assess absorption characteristics and chemical equilibria.
- Remote Sensing: Utilizing satellite technology, scientists can capture vast data sets on surface water quality changes over time, providing insights into the effects of light on aquatic ecosystems on a global scale.
- Experimental Aquaria: Controlled environments enable researchers to manipulate light conditions and observe behaviors and physiological changes in aquatic organisms.
- Advantages: Broad coverage and the ability to monitor inaccessible areas.
Through these methodologies, significant knowledge has been gained about the dynamics at play between light and water, facilitating advancements in both theoretical and applied sciences.
Case Studies on Light-Driven Water Processes
Examining specific case studies also helps illustrate practical applications of the light-water investigation. Notable instances highlight innovations and results that have emerged from this research:
- Photocatalytic Water Purification: Research has shown that titanium dioxide, when excited by UV light, can degrade organic pollutants in water. Implementation of this method in wastewater treatment plants has demonstrated notable reductions in harmful contaminants.
- Algal Bloom Predictions: A case study in several freshwater lakes analyzed the relationship between light intensity and the occurrence of harmful algal blooms. The findings revealed that increased light exposure in nutrient-rich waters can heighten bloom occurrences, thus informing management practices and monitoring efforts.
- Ecological Restoration Projects: Several projects have explored how adjustments in light conditions can foster the regrowth of submerged aquatic vegetation. By installing floating structures to moderate light intensity at certain depths, researchers observed enhanced vegetation health and a subsequent rise in biodiversity.
"By understanding how light affects water quality, we can unlock new methods for tackling pollution and promoting healthier aquatic ecosystems."
These case studies exemplify the fruitful outcomes from delving into the interplay between light and water, showing how targeted research can lead to significant ecological advancements and informed regulatory approaches. Understanding this relationship holds promise for better conservation strategies and sustainable practices in water management.
Technological Advances in Light and Water Research
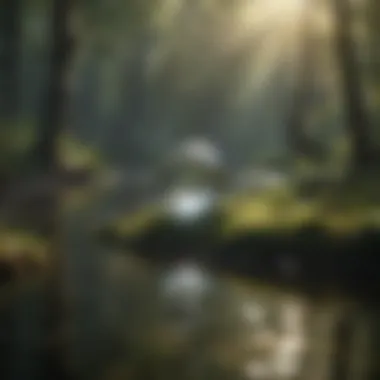
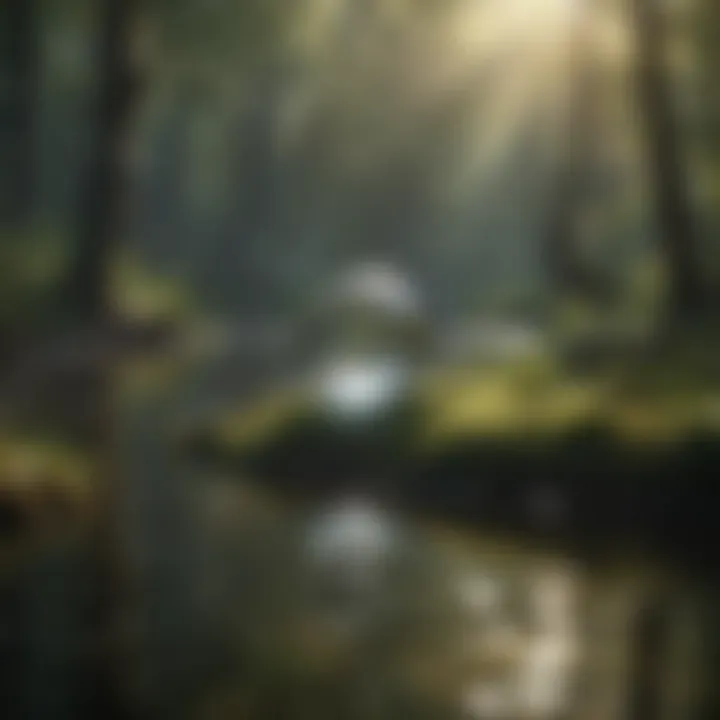
In the ever-evolving field of environmental science, the links between light sources and water quality are becoming clearer through advanced technologies. These innovations facilitate a better grasp of the dynamic interplay between aquatic systems and light. Understanding these developments is crucial not only for academic inquiry but also for practical applications in water management and conservation efforts.
The integration of technology and environmental research presents new avenues for monitoring and analysis. By harnessing these advancements, researchers and professionals can gain insights that were previously out of reach. From improving water treatment processes to enhancing ecosystem assessments, the potential benefits are vast.
Remote Sensing Technologies
Remote sensing encompasses a range of techniques used to gather data about water bodies from a distance. These technologies can identify changes in water quality by analyzing reflected light and other radiation phenomena.
An example of remote sensing is the use of satellite imagery to monitor algal blooms. By examining the optical signals and assessing the chlorophyll concentrations, scientists can pinpoint areas where blooms are likely forming.
Some significant elements of remote sensing include:
- Cost Efficiency: Reduces the need for extensive on-site studies.
- Large Coverage: Monitors expansive regions in a single analysis.
- Temporal Flexibility: Provides data from various time frames, allowing for trends and patterns to be established.
This technology plays a pivotal role in understanding seasonal variations in water quality, enabling managers to anticipate potential issues before they escalate.
Innovations in Water Quality Monitoring
Innovations in water quality monitoring are paramount in ensuring that aquatic environments are sustainably managed. New methods not only enhance the accuracy of data collected but also help in making prompt decisions regarding water resources.
For instance, autonomous sensor networks are becoming more prevalent. These devices continuously monitor parameters like pH, temperature, and turbidity in real-time. With their ability to send alerts when certain thresholds are exceeded, these sensors become powerful tools for proactive water quality management.
A few key benefits of these innovations are:
- Real-time Data Collection: Immediate feedback on water conditions facilitates swift interventions.
- Enhanced Precision: Advanced technologies reduce human error in data interpretation.
- Increased Reach: These devices can function in remote or hazardous locations where manual sampling might be risky.
Adopting these technologies not only elevates research capabilities but also directly contributes to better policies regarding water management and environmental conservation.
"The future of water quality monitoring lies in the synergy of technology and data—where informed decisions lead to sustainable practices."
In summary, the advancements in light and water research are revolutionizing how we understand and manage our aquatic systems. From remote sensing to sophisticated monitoring techniques, these innovations promise to enhance water quality and preserve ecosystems effectively.
Implications for Policy and Management
In recent times, the discourse surrounding light sources and their consequential impact on water quality has garnered significant attention. This topic is paramount, especially as policymakers and environmental managers seek methods to sustain aquatic ecosystems. Effective regulation and management practices can provide a foundational framework for conserving biodiversity and ensuring clean water resources.
Understanding light's role in aquatic environments extends beyond mere understanding; it informs regulations that control light emissions, which play a crucial part in combating issues like light pollution. This pollution is often overlooked but has downstream effects on aquatic fauna and flora, fostering disruption in their natural behaviors and habitats. Thus, strategies need to be integrated into existing environmental policies to ensure light emissions are managed appropriately.
Moreover, the connection between light sources and water quality must be recognized not just at a local level, but those implications ought to resonate through all layers of governance. Significant benefits arise when light management is thoughtfully woven into water management policies:
- Improved Ecosystem Health: Regulating light emissions can reduce disruptions caused by artificial lighting, thus supporting the breeding and feeding patterns of aquatic life.
- Enhanced Water Treatment: Incorporating light-based technologies in water treatment processes—such as solar disinfection—ensures that regulations are forward-looking and nurture innovation in treatment practices.
- Resilience Against Climate Change: Light-source management practices can help ecosystems adapt to the ongoing pressures of climate change, offering mechanisms to maintain resilience in these systems.
Furthermore, continuous monitoring and assessment are key. Crafting adaptable policies that can pivot based on ongoing research is fundamental. Each of these areas is interlinked, creating a delicate balance between human activities and the health of aquatic ecosystems.
"The intersection of light source management and water quality represents a crucial nexus in today’s environmental governance frameworks."
Regulations on Light Emission
Light emissions, particularly from artificial sources, have subtle yet profound impacts on aquatic systems. Though natural sunlight is indispensable for biological processes, excessive artificial light can disturb the circadian rhythms of various organisms.
Existing regulations on light emissions focus on limiting the intensity and duration of light pollution. Local governments must consider guidelines for residential, commercial, and industrial lighting, emphasizing the minimization of direct light exposure into water bodies. This involves employing technologies like shielded fixtures, which ensure that light is directed downward rather than sprawling outwards. Moreover, regulatory entities could impose restrictions on lighting during specific periods, such as breeding seasons for fish or migratory months for amphibians.
At national and international levels, there is scope for comprehensive legislation to assess and mitigate the risks posed by artificial light. Collaboration among agencies can yield a more coherent and enforceable framework. However, enforcement of these regulations often remains a challenge due to varying levels of compliance and awareness within communities.
Management Practices for Sustainable Use
Sustainable management practices regarding light and water quality ought to pair effective strategies with responsible stewardship. Adopting smart lighting solutions is key. Utilizing low-impact lighting technologies not only reduces light pollution but also cuts back on energy consumption, thus bringing down overall costs.
Engaging stakeholders—from local communities to industries—presents an opportunity to cultivate partnerships that can foster sustainable practices. This may include educational campaigns that highlight the effects of light pollution. Local schools, businesses, and conservation organizations can all play roles in disseminating information.
Additionally, establishing buffer zones around critical water bodies can mitigate the impact of light sources. Creating shaded areas with indigenous vegetation helps absorb excess light and reduce direct exposure to sensitive ecosystems.
Efforts should also be geared toward integrating these management practices within the broader context of water resource management. By incorporating a light quality assessment into water monitoring programs, agencies can better understand the ultimate effects of light sources on drinking water quality, recreational waters, and habitat integrity. This way, policies can adapt over time to the evolving knowledge base concerning light's influence on aquatic ecosystems.
Equally important is the role of research in developing more effective management strategies. The establishment of interdisciplinary teams that investigate the symbiotic relationship between light sources and aquatic environments can yield innovative solutions.
Bringing these practices to fruition will lead to healthier water bodies, ultimately benefiting both ecosystems and human well-being.
Ending
The exploration of the relationship between light sources and water quality is not just an academic endeavor; it holds vital implications for both environmental and human health. Understanding how different light wavelengths interact with water and their ecological impacts helps in comprehending the broader picture of aquatic systems. This discussion provides essential insights into the functioning of habitats and ecosystems, emphasizing the importance of maintaining balance in our natural resources.
Evidently, several elements come into play when concluding this intricate relationship. First, we need to reiterate how light sources, both natural and artificial, influence various biological and chemical processes. For instance, ultraviolet light plays a significant role in disinfection methods, while visible light is crucial for photosynthesis, affecting oxygen levels.
Secondly, acknowledging the pressing matter of light pollution magnifies the need for effective management strategies. The damaging effects of artificial lights on marine life are becoming more prominent, bringing attention to regulatory measures designed to protect aquatic ecosystems.
Lastly, a holistic understanding of this interplay promotes informed decisions in policy-making and sustainable practices. With increasing pressures from industrial activities and urbanization, recognizing the significance of light's quality and quantity can lead to improved water treatment methods and conservation efforts.
In summary, the topic explored in this article weaves a narrative that illustrates the profound connections between light and water. It serves as a solid foundation for further inquiry and highlights the ongoing need to align scientific understanding with real-world applications and ecological stewardship.
Recap of Key Insights
Several key points stand out in this discussion about light and water quality:
- Light Sources: Distinguishing between natural and artificial light sources is essential, as they serve different roles in aquatic environments.
- Photosynthesis: This is heavily reliant on light quality and intensity, directly impacting aquatic food chains and species diversity.
- Chemical Interactions: Variations in light wavelengths modify water chemistry, affecting the solubility and bioavailability of nutrients.
- Pollution Considerations: Light pollution poses a significant threat to aquatic ecosystems, necessitating careful management and mitigation strategies.
In reflecting on these insights, it becomes clear that light's influence on water quality stretches across multiple disciplines, underscoring the need for interdisciplinary approaches in research.
Future Directions for Research
Looking ahead, we can identify several promising directions for future exploration concerning light and water:
- Innovative Water Treatment Technologies: Researching new photonic treatments could lead to breakthroughs in water purification methods that harness specific light wavelengths for enhanced efficacy.
- Impact Studies on Aquatic Species: Long-term studies examining the effects of varying light conditions on different aquatic species will help understand potential shifts in ecosystems.
- Modeling Light Pollution Effects: Developing models to predict the long-term effects of light pollution on marine life can guide policy and urban planning effectively.
- Integrating Remote Sensing: Employing advanced remote sensing technologies to monitor aquatic light conditions and their correlation to water quality metrics would open new avenues for data collection and analysis.
- Collaborative Research Initiatives: Encouraging interdisciplinary collaborations among ecologists, environmental scientists, and policy-makers will ensure that the findings translate into actionable policies for ecosystem conservation.
Through focused awareness and commitment to research, we can better navigate the challenges at the nexus of light and water quality, aiming for a sustainable future.