Exploring Freeze-Thaw Lysis: Mechanisms and Applications
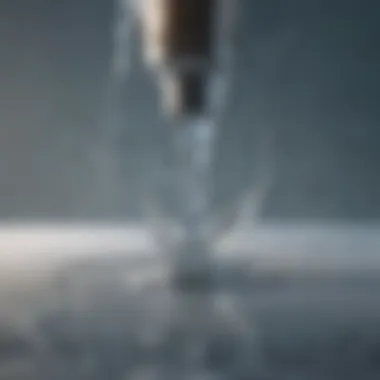
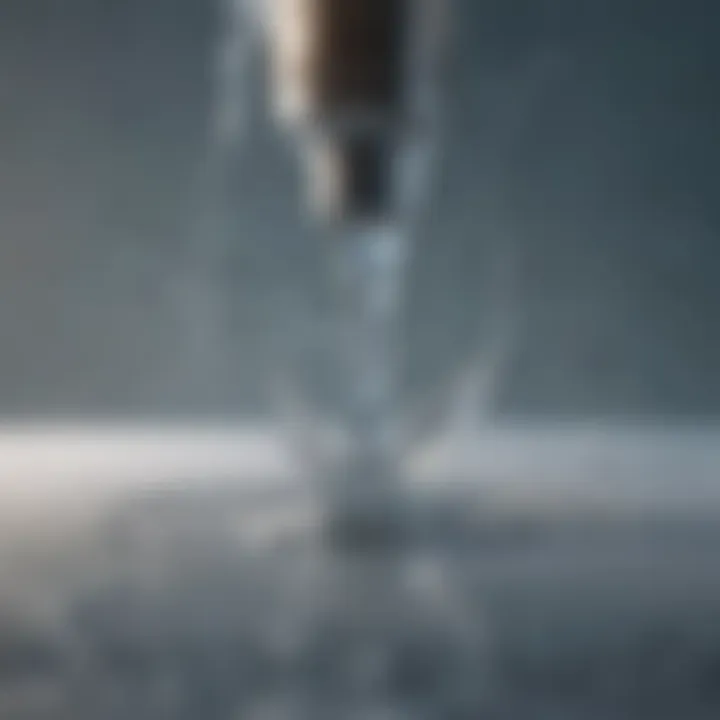
Overview of Research Topic
Brief Background and Context
Freeze-thaw lysis is a pivotal technique in cellular biology. It involves subjecting cells to cycles of freezing and thawing, subsequently causing disruption of cellular membranes. This occurrence arises due to the formation of ice crystals within cells during freezing, which leads to mechanical stress on cellular structures. The understanding of this process is crucial for various scientific applications, including molecular biology, microbiology, and biochemistry. It offers a straightforward method to lyse cells, thereby releasing their components for further analysis.
Importance in Current Scientific Landscape
The significance of freeze-thaw lysis in research cannot be overstated. In recent years, there has been a growing reliance on this method for obtaining cellular extracts, enabling the study of proteins, nucleic acids, and other biomolecules. As modern science evolves, the demand for efficient and effective lysis techniques increases. Researchers seek to balance efficacy with minimal cell damage, making freeze-thaw lysis an appealing option due to its simplicity and cost-effectiveness. Additionally, with the progression of genetic research and biotechnology, the precise mechanisms behind this lysis method warrant deeper exploration to optimize its application.
Methodology
Research Design and Approach
In examining freeze-thaw lysis, a systematic research design is essential. This encompasses an exploration of the physical principles governing this method. Key variables include substances used in the lysis process, temperature gradients, and freeze-thaw cycles. These factors can significantly influence the efficiency of cell lysis.
Data Collection Techniques
Essential techniques for data collection range from quantifying cell lysis via spectrophotometry to utilizing flow cytometry for assessing cell viability post-lysis. Additionally, analytical methods such as western blotting or PCR can help evaluate the integrity of released biomolecules. Such methodologies provide vital insights into the effectiveness of freeze-thaw lysis across various cell types.
"The freeze-thaw method provides a flexible approach to cellular lysis, valuable across multiple scientific fields."
Understanding the strengths and limitations associated with this technique aids in refining protocols. Optimizing the freeze-thaw cycles and exploring combinatorial methods can lead to enhanced yield and quality when extracting cellular components. The subsequent sections will delve deeper into these crucial aspects, shedding light on the intricacies of freeze-thaw lysis.
Prolusion to Freeze-Thaw Lysis
Freeze-thaw lysis is a vital technique in many scientific fields, ranging from molecular biology to biotechnology. This process involves the cyclic freezing and thawing of cell samples, which disrupts the cellular structure and leads to the release of intracellular contents. Understanding the mechanisms behind freeze-thaw lysis can provide insights into its various applications and inherent challenges.
Definition and Historical Context
Freeze-thaw lysis refers to the breakdown of cell membranes caused by the expansion of ice when water within the cells freezes. When the water freezes, it creates ice crystals that can puncture the cell membrane, leading to cell death and the release of cellular components. This method has been utilized in laboratories since the mid-20th century, primarily for its simplicity and effectiveness in breaking open cells for further analysis. Historical techniques have evolved alongside advancements in freezing technologies, making this process more reliable and applicable to various organisms and cell types.
Significance in Scientific Research
The significance of freeze-thaw lysis in scientific research cannot be overstated. This technique serves multiple purposes, especially in the extraction of proteins, nucleic acids, and other biomolecules. It is favored for its ability to yield a high purity of cellular components without the need for complex chemical lysis buffers. Furthermore, the protocol is adaptable and can be modified depending on the type of cells being disrupted.
Freeze-thaw lysis is essential for obtaining samples in numerous applications, including drug development, genetic studies, and diagnostic tools.
Despite its many benefits, researchers must also be aware of potential limitations. For example, repeated freeze-thaw cycles can lead to significant cell damage. This aspect could alter the properties of the extracted components, which may impact downstream applications. Therefore, understanding the delicate balance of conditions during freeze-thaw lysis is crucial for maximizing its efficiency in scientific inquiries.
Cellular Structure and Temperature Sensitivity
Understanding cellular structure and its sensitivity to temperature is fundamental in the context of freeze-thaw lysis. Each cell is encased in a membrane composed of phospholipids, proteins, and carbohydrates, all contributing to its unique properties. The integrity of this membrane is critical for cellular function and stability, as it regulates what enters and exits the cell. When exposed to temperature changes, especially during freeze-thaw cycles, these membranes can experience significant stress, potentially leading to cellular rupture or lysis.
This aspect of cellular structure is not merely academic; it has real implications for research methodologies. Identifying how different cell types respond to temperature fluctuations guides laboratory protocols. For instance, certain mammalian cells might withstand freeze-thaw cycles better than bacterial cells. A clear grasp of these differences aids in optimizing lysis conditions, minimizing cell damage, and ensuring reliable extracts of cellular components for further study.
Overview of Cell Membrane Composition
The cell membrane plays a pivotal role in maintaining cellular homeostasis. It is primarily composed of a bilayer of phospholipids with embedded proteins that can have various functions, including transport, signaling, and structural support. This composition allows for selective permeability, which is crucial during lysis processes.
When cells undergo freeze-thaw lysis, the lipid bilayer can become rigid. Ice crystals may form within and outside the cell, disrupting the lipid arrangement. This disruption can cause proteins to misfold, leading to loss of functional integrity. Understanding the specific composition of cell membranes, such as the types of fatty acids present, is essential for predicting how different cells may respond to thermal stress.
Responses to Temperature Changes
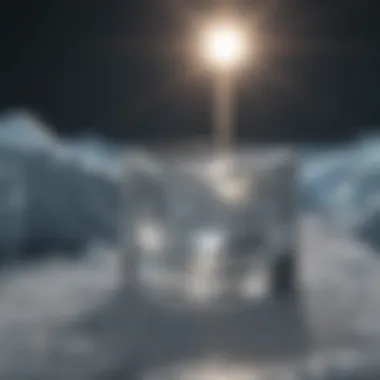
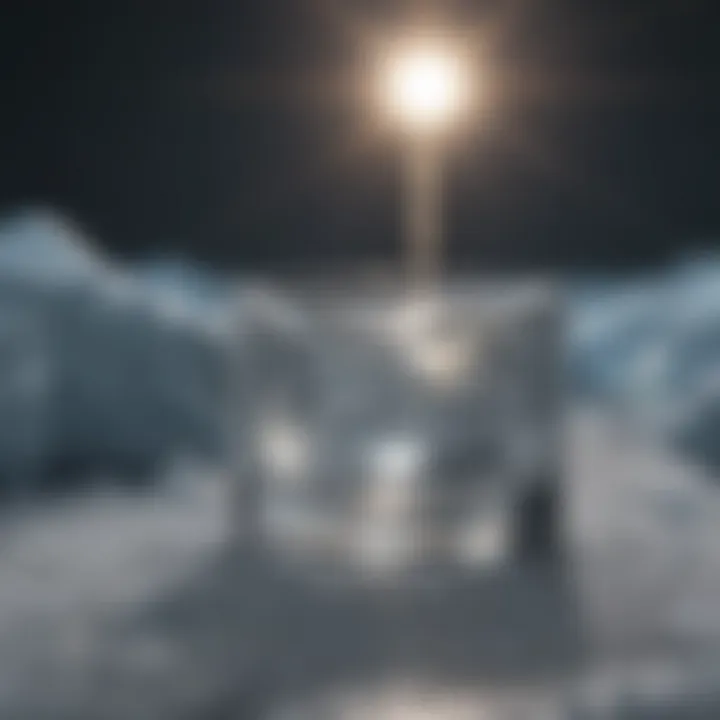
Cells respond to temperature fluctuations in various ways. When cooled during the freezing phase, the metabolic processes slow down, and the fluidity of the membrane decreases. These changes are reversible to some extent during thawing, but the formation and growth of ice crystals can cause irreversible damage. Therefore, it is crucial to manage the rate of freezing and thawing. Slow cooling may allow cells to adapt, while rapid changes can lead to detrimental results.
Key point: The rate of temperature change significantly impacts cell survival during freeze-thaw cycles.
Moreover, the cytoplasmic contents can also affect how cells react to temperature changes. High levels of solutes can increase osmotic pressure during freezing, intensifying stress on the membrane. In laboratory settings, differential responses to temperature fluctuations must be considered, especially when aiming to extract proteins or nucleic acids post-lysis. This knowledge allows researchers to tailor their approaches, thereby improving the efficiency and efficacy of freeze-thaw lysis techniques.
Mechanisms of Freeze-Thaw Lysis
Freeze-thaw lysis is a critical process used extensively in laboratories to break open cells and release their contents. Understanding the mechanisms involved can illuminate how cellular structures respond to extreme temperature changes. This understanding is essential not just for academic inquiry but also for practical applications in biochemistry, molecular biology, and genetic engineering. The knowledge gained from comprehending these mechanisms guides researchers to optimize the lysis process effectively.
Physical Changes During Freezing
When cells are subjected to freezing, several physical changes occur within both the cellular structure and the surrounding environment. One key aspect is the formation of ice crystals. As the temperature drops, water within the cells begins to solidify, resulting in ice crystal formation. These crystals are not merely static structures. They grow in size and can puncture the cell membranes.
The osmotic effects associated with freezing also play a significant role. Water molecules move from areas of higher concentration to lower concentration during the freezing process. This movement can lead to cellular dehydration and subsequent damage to membranes. It is crucial to recognize that the rate of freezing can influence the size of ice crystals formed. Rapid freezing tends to create smaller ice crystals, which can minimize damage to cellular integrity. On the other hand, slow freezing often leads to larger crystals that significantly compromise the structure of cell walls.
Thawing Process and Its Effects
Once the freeze has been completed, the thawing process introduces its own set of challenges. The transition from a frozen state to a liquid state is not just a simple reversal of the freezing process. During thawing, the ice crystals melt, releasing the contents contained within the cells. This sudden increase in liquid volume can lead to osmotic shock, which may cause further membrane rupture.
Additionally, if the thawing occurs unevenly, it can result in localized concentrations of solutes, which may further stress the cells. For optimal lysis, the thawing process should be controlled and carefully executed to minimize damage. Thawing should generally be performed gradually, allowing cells to equilibrate slowly back to room temperature.
Role of Ice Crystal Formation and Rupture
The ice crystals formed during the freezing stage are vital to the lysis process. Their formation and subsequent rupture are key drivers of cellular disruption. Ice crystals, when they expand, can compromise the integrity of not only the cell membrane but also the cytoskeletal components within cells. This breaking down of structural components effectively leads to lysis.
Moreover, it is essential to examine how different types of cells react to ice crystal formation. Certain cell types are more resilient to ice crystal damage than others, adding complexity to the freeze-thaw lysis process. Eukaryotic cells, for example, typically have a more complex structure than prokaryotic cells, making them potentially more susceptible to freeze-thaw damage. Understanding these variances is crucial for maximizing extraction yields and ensuring reproducibility.
"In freeze-thaw lysis, the precise control over freezing and thawing conditions directly influences the efficiency of cellular content extraction."
Methods for Implementing Freeze-Thaw Lysis
Methods for implementing freeze-thaw lysis are crucial when examining how temperature influences cellular integrity and function. This section outlines essential protocols, optimization strategies, and variations among different organisms. Each aspect is designed to maximize cell lysis efficiency while minimizing potential damage.
Basic Protocols for Freeze-Thaw Lysis
The fundamental protocols for freeze-thaw lysis typically follow a straightforward pattern. The basic procedure includes the following steps:
- Preparation of Samples: Cells are harvested and washed to remove any residual growth medium or debris. A buffer solution may be added to maintain pH stability during the process.
- Freezing: The samples are then subjected to freezing, often at temperatures around -80 Β°C. This step is critical, as rapid freezing can lead to more uniform ice crystal formation, which is advantageous for effective lysis.
- Thawing: Thawing usually occurs at room temperature or in a water bath, avoiding extreme temperature shifts that may compromise cell integrity.
- Centrifugation: After thawing, centrifugation can separate lysed cellular debris from soluble components, allowing for further analysis of released materials, such as proteins or nucleic acids.
By adhering to these basic protocols, researchers can ensure reliable outcomes when employing freeze-thaw lysis in their experiments.
Optimization of Lysis Conditions
Optimizing lysis conditions is key to achieving superior results in freeze-thaw lysis. Several factors must be taken into consideration:
- Freezing and Thawing Cycles: The number of freeze-thaw cycles can significantly affect cell lysis efficiency. Typically, two to three cycles are sufficient for most cell types. Excessive cycles may lead to increased cell damage.
- Time of Exposure: The duration of time spent in both the freezing and thawing phases should be optimized. Extended exposure at suboptimal conditions might inhibit effective lysis.
- Buffer Composition: Using appropriate lysis buffers contributes to successful extraction. Buffers containing detergents, like Triton X-100, can enhance lysis by disrupting lipid bilayers.
Attention to these elements fosters a conducive environment for comprehensive cellular disruption while minimizing degradation of sensitive biomolecules.
Variations in Methodology Across Organisms
Different organisms exhibit diverse responses to freeze-thaw lysis due to variations in cellular structure and composition. Understanding these differences is essential for tailoring lysis protocols effectively.
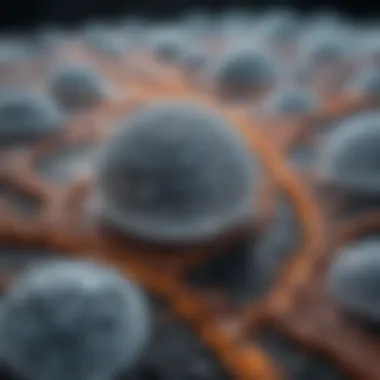
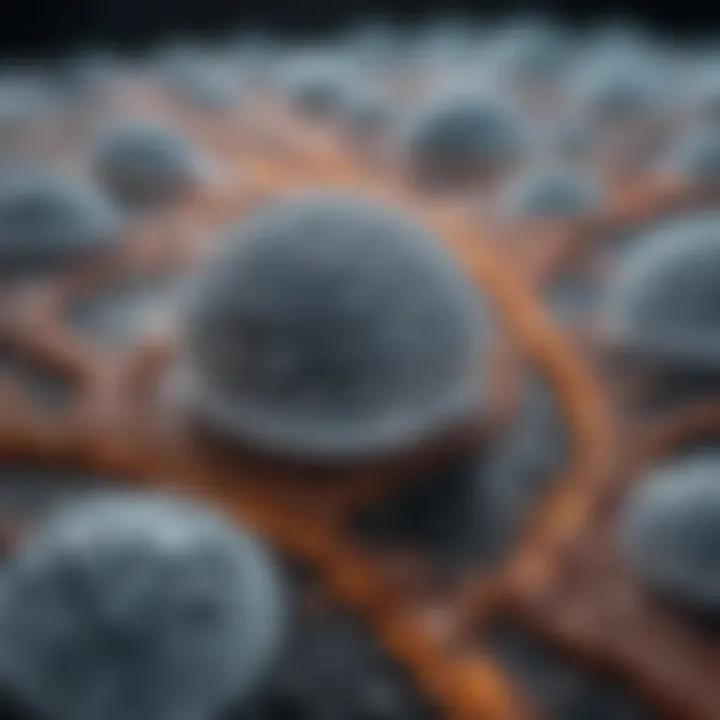
- Bacterial Cells: Bacteria are often more resilient to freeze-thaw stress. Therefore, harsher conditions may be used, including increasing the number of freeze-thaw cycles.
- Plant Cells: Plant cells, characterized by rigid cell walls, require specific treatments, such as pre-chilling or enzymatic treatments to facilitate lysis.
- Animal Cells: Animal cells are delicate and often require precise optimization of lysis conditions, as the membrane integrity can be easily compromised.
It is essential to adapt protocols for freeze-thaw lysis based on the organism being studied to achieve reliable and reproducible results.
Applications of Freeze-Thaw Lysis
The applications of freeze-thaw lysis are extensive and essential in modern biological and biochemical research. This technique allows researchers to manipulate cellular structures in a way that facilitates the extraction of various biological materials. Understanding the significance of freeze-thaw lysis not only highlights its practical uses but also informs best practices for implementation in diverse experimental settings.
Extraction of Cellular Components
Freeze-thaw lysis primarily serves as a method for extracting cellular components, including proteins, nucleic acids, and organelles. The process disrupts cell membranes by inducing physical stress, which causes them to become permeable.
This disruption is vital for obtaining high-quality cellular extracts. Some key points to consider include:
- Yield and Purity: Proper execution of freeze-thaw cycles can result in higher yields of extracted materials while maintaining purity essential for subsequent analyses.
- Protocol Variability: Different cell types respond uniquely to freeze-thaw lysis. Tailoring the protocol to the specific cell line or organism is important for optimal extraction efficiency.
- Storage Conditions: Following lysis, components must be stored under suitable conditions to preserve their stability, particularly proteins which can degrade if not handled correctly.
Impact on Protein Studies
In protein studies, freeze-thaw lysis plays a crucial role by allowing researchers to study proteins in a native state. The technique assists in releasing enzymes and other crucial proteins from within cells, enabling scientists to analyze these biomolecules in detail.
Some considerations include:
- Enzyme Functionality: Since freeze-thaw lysis can sometimes alter protein structures, it is important to evaluate enzyme activity post-lysis to ensure consistent results in experiments.
- Protein Stability: Proteins may be more susceptible to denaturation upon repeated freeze-thaw cycles. Limiting the number of cycles minimizes the risk of compromised function in downstream applications.
- Analytical Techniques: Extracted proteins can be subjected to various analyses such as Western blotting, mass spectrometry, and enzyme assays, allowing for in-depth studies of cellular processes.
Role in Genetic Material Isolation
Freeze-thaw lysis is also employed in the isolation of nucleic acids, including DNA and RNA. This method facilitates the release and purification of genetic material from cells, making it a common choice in molecular biology applications.
Key aspects include:
- Yield of Nucleic Acids: Effective lysis contributes directly to the yield of extracted genetic material. Protocol optimization is often necessary to ensure high recovery rates.
- Contamination Concerns: Proper lysis techniques can help mitigate contamination risks, resulting in cleaner isolations which are critical for successful downstream applications like PCR or sequencing.
- Interdisciplinary Applications: The ability to extract high-quality nucleic acids is crucial for various fields, from forensic analysis to genetic engineering, highlighting the versatile utility of freeze-thaw lysis in research.
The effectiveness of freeze-thaw lysis in extracting cellular components, studying proteins, and isolating genetic material reveals its importance in advancing scientific knowledge across diverse disciplines.
Challenges and Limitations
In every scientific process, challenges accompany methods, and freeze-thaw lysis is no exception. Understanding these challenges is crucial for users of this technique. Each potential limitation can impact outcomes, reproducibility, and the quality of the results. Being aware of these factors enables scientists to design better experiments and implement solutions to mitigate risks associated with freeze-thaw lysis.
Potential Cell Damage During Lysis
One of the main concerns with freeze-thaw lysis is potential cell damage. When cells freeze, ice crystals can form within the cellular structure. These crystals can puncture membranes and disrupt cellular integrity. Especially with delicate cellular types like neurons or some cultured cell lines, the extent of damage can be significant. Thus, scientists must carefully consider the freezing rate and thawing process. Cells should undergo controlled freezing to minimize harm. Using cryoprotectants can also aid in reducing cellular damage during this process.
Contamination Risks
Contamination is another vital issue. During lysis, cellular contents, including proteins and nucleic acids, risk exposure to the external environment. This exposure can lead to contamination from microbes or other undesired molecules present in the surroundings. Proper handling techniques are crucial to mitigate these risks. Ensuring that all equipment used is sterile and that work is conducted in controlled environments, such as laminar flow hoods, is paramount. Regular monitoring for contamination should be implemented as an ongoing practice.
Reproducibility Issues
Reproducibility poses challenges as well. Variability in experimental conditions can lead to inconsistent results. Factors such as the freezer's temperature, lysis duration, and the specific protocol followed contribute to this variability. Special care must be taken to standardize procedures. Keeping detailed documentation of protocols is imperative for any research group. This way, other scientists can replicate the experiment accurately. Addressing these reproducibility issues promotes confidence in findings, thus advancing the discipline.
Effective solutions to challenges in freeze-thaw lysis require ongoing research and awareness of best practices.
Advancements in Freeze-Thaw Lysis Techniques
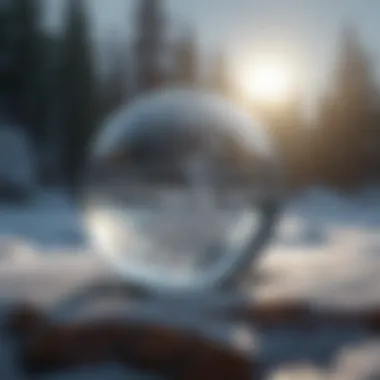
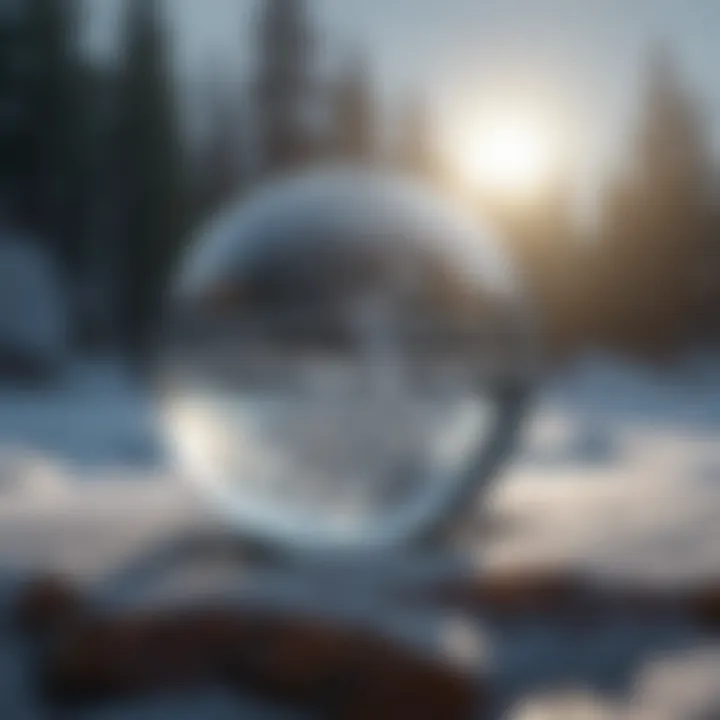
Advancements in freeze-thaw lysis techniques play a pivotal role in enhancing the efficacy and quality of scientific research. This section aims to dissect these innovations by investigating newer equipment and automation processes that address previous challenges faced in freeze-thaw lysis methodologies.
Innovations in Equipment
In recent years, significant progress in laboratory equipment tailored for freeze-thaw lysis has emerged. Improved cryogenic containers allow for better control over the freezing and thawing rates. These advances mitigate the risk of cellular damage, which is crucial for preserving the integrity of extracted components.
Further, specialized lysis buffers are now available that are optimized for use with modern equipment. The refinement of these buffers enhances the rate of lysis, thereby maximizing yield.
Some notable innovations include:
- Programmable Freezers: These devices enable precise temperature control and timed cycles, which minimize thermal shock to cells.
- Multi-sample Thawing Systems: Such systems allow for simultaneous thawing of multiple samples, ensuring uniformity in the lysis process.
- Real-time Monitoring Technologies: With sensors and analytics, researchers can now monitor cellular integrity and lysis efficiency during experiments.
These equipment innovations not only increase efficiency but also improve the reproducibility of the freeze-thaw lysis process across experiments.
Automation in Lysis Processes
Automation is becoming integral in implementing freeze-thaw lysis techniques. Automated systems reduce human error and variability, which have been significant barriers to standardization in research.
New robotic systems can perform freeze-thaw cycles with precision. Researchers can program these machines to follow exact protocols, which mitigates the risk of operator inconsistency. Some key benefits of automation in this context include:
- Increased Throughput: High-throughput automation allows labs to process numerous samples simultaneously, making the research process more efficient.
- Improved Consistency: Automated processes produce more consistent results than manual techniques, which is vital in reproducibility.
- Reduced Labor Costs: With fewer labor resources required for lysis, researchers can allocate personnel to more cognitively demanding tasks.
"The integration of advanced equipment and automation in freeze-thaw lysis techniques is a crucial development that enhances research outcomes and drives scientific discovery."
Future directions in this area hold promise for further refinement and broader applications across various scientific disciplines.
Future Directions in Research
The field of freeze-thaw lysis continues to evolve, marked by advancements that offer new perspectives on its mechanisms and applications. As research progresses, understanding these future directions can benefit scientists across various disciplines. It is important to anticipate how developments can enhance methodologies and broaden scientific inquiry.
Emerging Technologies Related to Freeze-Thaw Lysis
Recent innovations in technology have improved the efficiency and effectiveness of freeze-thaw lysis procedures. One significant area of development involves the refinement of cryopreservation techniques, which ensure cellular integrity during the freeze-thaw cycle. Technologies like automated freezers and precise temperature control systems minimize variability in sample handling. These innovations not only facilitate reproducibility but also allow for more consistent lysis performance.
Another emerging technology is the integration of advanced imaging methods. Techniques such as cryo-electron microscopy provide insights into the structural changes of cells during freezing and thawing. This can help researchers understand how different conditions affect ice crystal formation and cell viability, leading to improved lysis protocols.
Potential Interdisciplinary Applications
The implications of freeze-thaw lysis extend beyond traditional life sciences. Its principles can be adapted across various fields, including biotechnology and pharmaceutical development. For instance, in drug formulation, understanding how freeze-thaw cycles influence the stability of vaccines can lead to enhanced preservation methods.
In environmental science, freeze-thaw lysis can be utilized to study microbial communities in polar regions. Researchers can explore how these organisms adapt to extreme conditions, contributing to our knowledge of ecological resilience. Likewise, agricultural sciences may benefit from insights into plant cell lysis, enabling improvements in crop resilience against climate fluctuations.
"The future of freeze-thaw lysis lies in its versatility, allowing for interdisciplinary collaboration that advances our understanding of both cellular and environmental dynamics."
The landscape of freeze-thaw lysis research is dynamic, with numerous opportunities for exploration. Emphasizing the necessity of interdisciplinary approaches will drive innovation and deepen scientific understanding.
End
Summary of Key Findings
Several important findings emerge from this exploration of freeze-thaw lysis:
- Mechanisms of Action: The freeze-thaw process exploits ice crystal formation and subsequent rupture, which is fundamental to cell lysis.
- Applications: The technique has diverse applications ranging from protein extraction to genetic material isolation, proving critical in various research fields.
- Challenges: Potential issues such as cellular damage and contamination must be carefully managed to ensure reproducible results.
- Technological Advancements: Innovations in equipment and methods continue to improve the efficiency and effectiveness of freeze-thaw lysis protocols.
Overall Importance of Freeze-Thaw Lysis in Scientific Research
The relevance of freeze-thaw lysis extends beyond its immediate applications. It provides a gateway to significant insights in cell biology and molecular research. By facilitating the extraction of cellular components, it aids scientists in unraveling complex biological processes. Furthermore, ongoing advancements in this area signify that it will remain an integral part of scientific methodology.
As research continues to evolve, the methodologies surrounding freeze-thaw lysis will adapt, further enhancing its utility in scientific exploration. This adaptability suggests a promising future for this technique, underscoring its fundamental role in both current and future research landscapes.