FACS Cell Sorting: Principles and Innovations in Biology
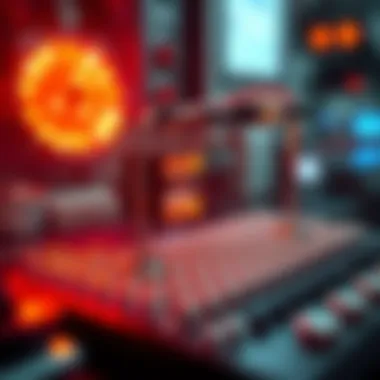
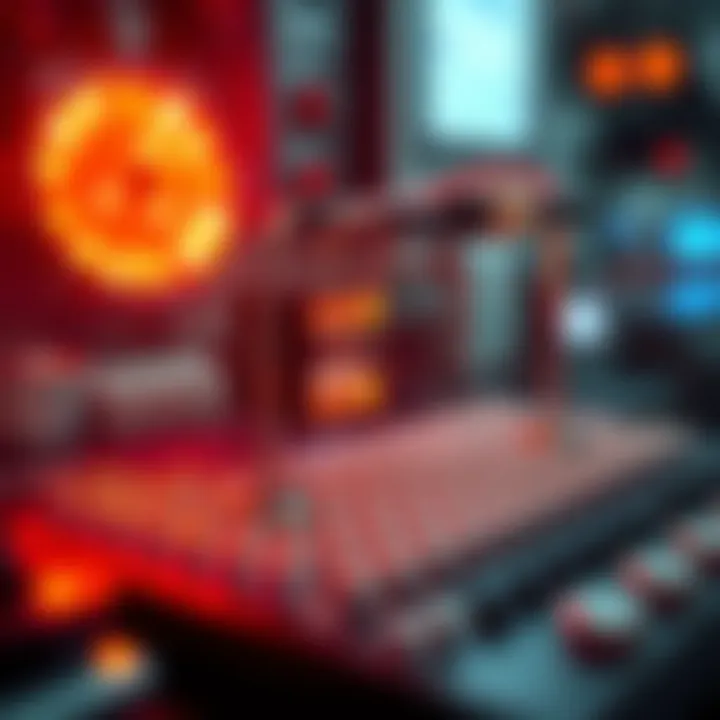
Overview of Research Topic
Fluorescence-Activated Cell Sorting (FACS) represents a cornerstone in cellular biology, enabling scientists to separate and analyze cells based on their unique properties. The technique leverages fluorescent labeling to sort and categorize a mixed population of cells, allowing detailed study of their functions, behaviors, and interactions. Understanding FACS necessitates a dive into its principles, methodologies, and the transformative role it plays in contemporary research and clinical applications.
Brief Background and Context
FACS emerged from the marriage of fluorescence microscopy and flow cytometry in the late 20th century. Initially developed for basic research, this tool has expanded into a multitude of fields, from immunology to cancer research. The integration of advanced laser technology and sophisticated software has propelled FACS into a realm where it can sort millions of cells per minute, opening doors to unprecedented insights into cell biology.
Importance in Current Scientific Landscape
The ability to analyze and purify specific cell types has profound implications. In immunology, for instance, researchers can dissect immune responses at a cellular level, identifying specific subsets of immune cells that are pivotal in disease processes. In clinical settings, FACS is instrumental in developing personalized therapies, such as CAR-T cell therapy, tailored to individual patient needs. This adaptability illustrates the significance of FACS in driving innovations in scientific research and therapeutic interventions.
Methodology
Research Design and Approach
Investigating FACS requires a multidisciplinary approach that encompasses elements of biology, chemistry, and engineering. A fusion of experimental design and computational analysis is essential to maximize the utility of FACS technology. Each study utilizing FACS typically begins with a clear hypothesis and systematically isolates the cell populations of interest.
Data Collection Techniques
The data collection process in FACS is intricate yet systematic. To understand this, consider the following steps:
- Sample Preparation: Cells are prepared by suspending them in a fluid medium, alongside fluorescent markers that bind to specific cellular targets.
- Flow Cytometry: As cells pass through a laser beam, they emit signals that are captured by detectors. The emitted fluorescence indicates the presence of various biomolecules.
- Sorting Mechanism: Depending on the fluorescence signal and pre-defined criteria, cells are segregated into different populations either by deflecting them into specific containers or allowing them to flow into culture plates for further study.
The combination of these techniques allows for effective sorting and subsequent analysis or experimentation, ultimately yielding significant insights into cellular roles and interactions.
FACS is not just a tool; it's a gateway to understanding the fundamental behaviors of cells that can lead to groundbreaking therapies.
This overview underscores the vital roles that both methodology and innovative applications of FACS play in pushing the boundaries of what we know in cellular science.
Intro to FACS Cell Sorting
Fluorescence-Activated Cell Sorting, commonly known as FACS, represents a remarkable integration of biology with technology. This method is widely used for isolating and analyzing cells based on their specific properties, and its importance in the realm of molecular biology and immunology cannot be overstated. Whether it's conducting experiments in cell biology, evaluating immune responses, or developing targeted therapies, understanding FACS is essential for students, researchers, educators, and professionals alike.
One of the most significant benefits of FACS is its ability to provide a comprehensive analysis of cellular populations. Unlike older methods, FACS offers the precision needed to separate cells with high specificity. This allows for a detailed study of cell behavior and function, enabling researchers to glean insights that might remain uncharted with less advanced techniques. Not only does this technique facilitate research, but it also has profound implications in clinical settings where targeted therapies rely on the sorting of particular cell types.
Also, FACS can be seen as a vital bridge connecting the basic research to applied clinical studies. This technique does not just serve researchers in the lab but significantly shapes the outcomes of therapeutic interventions. By enabling the isolation of specific cell types, FACS plays a pivotal role in ensuring that therapies target their intended cells effectively, thus enhancing their efficacy and safety.
In addition to its practical applications, the evolution of FACS technology itself merits attention. Early versions of the technique, while groundbreaking at the time, have been continually refined. These innovations have allowed FACS to adapt to the ever-increasing demands of modern biology, making it more relevant today than ever.
This section lays the groundwork for our exploration by contextualizing FACS within both historical and functional paradigms. Understanding the essence of FACS and its historical journey sets the stage for delving deeper into its principles, applications, and innovations. In this way, we can appreciate not only what FACS accomplishes in the present but also envision where it is headed in the future.
Defining FACS and Its Relevance
FACS is fundamentally a form of flow cytometry, a technology used to measure the physical and chemical characteristics of cells as they flow in a stream through a laser beam. The ability to analyze multiple characteristics of individual cells at high speeds is vital for many research applications. One primary relevance stems from its use in immunophenotyping, where it is employed to identify specific cell types in immune responses, thus proving crucial for immunology studies.
Moreover, the procedure is not limited to just one discipline but crosses boundaries into various fields including oncology, microbiology, and genetics. For example, in cancer research, FACS is invaluable for isolating tumor-infiltrating lymphocytes, which can lead to breakthroughs in understanding tumor microenvironments. This widespread relevance illustrates not just the versatility of FACS but its integral role in advancing biological research across different domains.
Historical Context of FACS
To comprehend the significance of FACS today, it is essential to look back at its origins. The concept of flow cytometry was developed in the early 1960s, but it wasn't until the 1970s that the FACS technology emerged as a specific technique. This evolution illustrates a pivotal moment in scientific methodology, where biological understanding connected seamlessly with technological advancements.
The introduction of laser technology marked a turning point, helping researchers achieve new heights in cell analysis. Before FACS, cell sorting methods were labor-intensive and often inefficient. With FACS, researchers could now rapidly sort millions of cells in a relatively short time while simultaneously gathering data on various cellular characteristics.
In summary, the historical context of FACS not only highlights its revolutionary nature in cellular biology but also reflects the broader narrative of technological progress aligning with scientific inquiry. As our understanding of cellular functions grows, so too does the technology designed to explore these complexities.
Fundamental Principles of FACS
Understanding the fundamental principles of Fluorescence-Activated Cell Sorting (FACS) is crucial for grasping how this technique revolutionizes cell biology and immunology. FACS plays a pivotal role in the precise targeting and sorting of cells based on their fluorescent characteristics, enabling researchers to dissect complex biological phenomena. The importance of these principles lies in their ability to enhance scientific accuracy and efficiency, a necessity in modern research endeavors.
This section reflects on how these principles contribute to the robustness of FACS, highlighting the diverse methods and tools employed to sort cells effectively. By focusing on these foundational aspects, we can appreciate the intricate workings behind this powerful technique and its far-reaching implications in the scientific arena.
Basic Operational Workflow
At the heart of FACS lies its operational workflow, a well-orchestrated sequence of steps designed to deliver precise cell sorting. The initial phase starts with sample preparation, where cells are labeled with specific fluorescent markers. This labeling is what sets the stage for FACS to distinguish between different cell types.
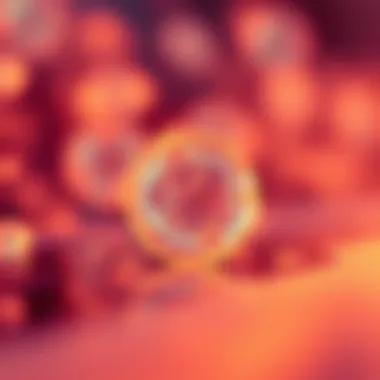
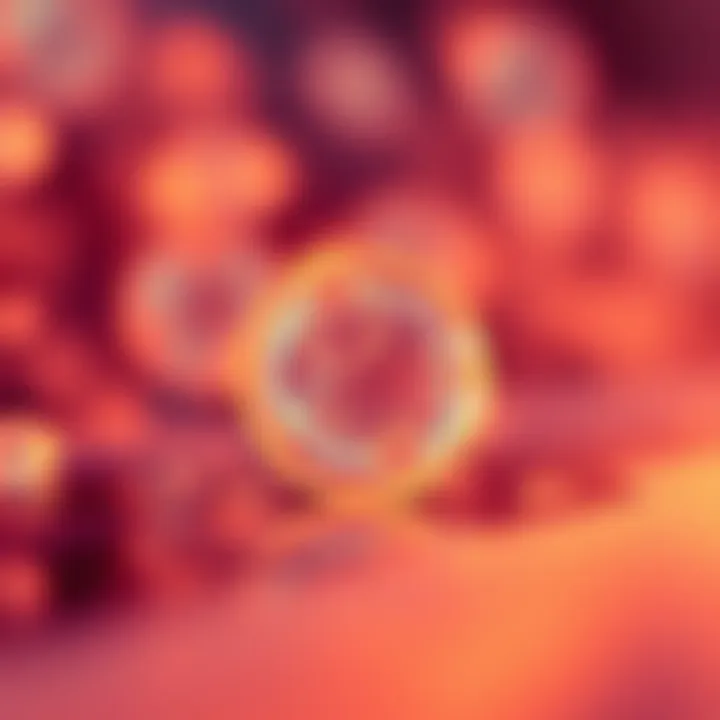
Once prepared, the sample is introduced into the flow cytometer. Here, cells flow in a single file through a laser beam. As the cells pass through this beam, the fluorescent dye emits light at various wavelengths. Detectors then capture this emitted light, allowing analysis of the cells’ characteristics in real time. This precision in detecting different properties underpins the workflow.
Key steps to remember in this basic operational workflow include:
- Sample Preparation: Labeling cells with fluorochromes.
- Focusing Stream: Ensuring a single-cell stream for accurate detection.
- Data Acquisition: Capturing emitted light to analyze cell properties.
Mechanics of Cell Detection
The mechanics behind cell detection are what give FACS its edge. As cells travel through the laser, they scatter light and emit fluorescence, which allows for various dimensions of data to be collected. This process involves distinguishing between different types of signals, such as forward scatter (FSC) and side scatter (SSC), which provide insights into cell size and granularity.
Using these signals, FACS can assess multiple parameters simultaneously. The ability to analyze cell characteristics in real-time, like viability or surface markers, is significant. These capabilities enable researchers to sort a specific cell population from a heterogeneous sample efficiently.
The mechanics include:
- Forward Scatter (FSC): Indicates the size of the cell.
- Side Scatter (SSC): Reveals internal complexity, like granularity.
- Fluorescent Emission: Analysis of fluorochromes for specific markers.
"The real-time analysis coupled with sorting capabilities has made FACS a vital tool in biomedical research and therapeutic advancements."
Sorting Mechanisms and Techniques
Sorting in FACS is more than simply separating cells; it employs sophisticated mechanisms to achieve high precision. The most common technique involves using electrostatic deflection to direct cells into discrete collection tubes based on their fluorescent signals. This capability allows researchers to isolate specific cell types, aiding in downstream applications such as further analysis or culturing.
There are several sorting techniques utilized in FACS, including:
- Bulk Sorting: For high-volume applications focusing on a larger population of cells.
- Single-Cell Sorting: Isolation of individual cells for single-cell analyses or cloning.
- Magnetic Sorting Integration: Enhancing sorting efficiency by using magnetic beads for certain applications.
The choice of sorting technique significantly impacts the outcomes of the research, as each method caters to different needs and throughput requirements. This underscores the adaptability and versatility of FACS technology in addressing diverse research questions in cell biology.
Technological Components of FACS
Fluorescence-Activated Cell Sorting (FACS) stands at the forefront of cell biology, largely due to its sophisticated technological components. These elements combine to form an intricate system that not only identifies cellular properties but also segregates cells with precision unparalleled in other methodologies. Understanding each component enhances our appreciation of FACS's capabilities and its transformation of biological research.
Laser Systems and Their Functionality
At the heart of FACS technology lie the laser systems, which play a pivotal role in the excitation of fluorophores. These lasers emit specific wavelengths, essential for energizing the dye molecules linked to the antibodies on the cell surfaces. The excitation leads to the release of light that can be detected and analyzed.
Functionality Considerations:
- Types of Lasers: Common types used in FACS include Argon, HeNe, and solid-state lasers, each suited to excite specific fluorochromes.
- Tuning and Stability: The tunability and stability of laser beams are crucial, as fluctuations can lead to inaccurate sorting.
- Power Output: Greater power outputs facilitate stronger signal detection, allowing researchers to work with lower concentrations of cells.
Inadequate laser systems can severely undermine the efficacy of cell sorting. High-quality lasers ensure both efficiency and reliability in cell analysis, directly impacting the integrity of biological conclusions drawn from the experiments.
Fluorochromes and Antibody Applications
The interaction between fluorochromes and antibodies is instrumental in determining cell types. Antibodies, tagged with fluorescent dyes, bind specifically to particular cell markers, enabling identification based on fluorescence during sorting. In turn, fluorochromes provide visual signals that facilitate detection by the FACS apparatus.
Key Considerations:
- Choice of Fluorochromes: Selecting appropriate dyes is crucial, as their excitation and emission spectra must align with the laser systems in use. Common choices include FITC, PE, and APC.
- Multiplexing Capabilities: By employing multiple fluorochromes, researchers can analyze several markers simultaneously, enhancing the data richness.
- Photobleaching: A downside to consider is photobleaching, where fluorochromes may lose their ability to fluoresce when exposed to light for prolonged periods.
Understanding these elements helps researchers interpret results more effectively, enabling advancements in understanding complex biological systems.
Data Acquisition Systems
Once the cells are sorted, collecting and interpreting the resultant data becomes paramount. The data acquisition systems in FACS are designed to capture the emitted light from the fluorescent markers, converting it into quantifiable data. This process involves complex software and computational tools to analyze the information accurately.
Important Aspects:
- Signal Processing: Advanced algorithms filter out background noise and enhance the signals corresponding to the fluorescent markers, ensuring the accuracy of the data collected.
- Real-time Analysis: The ability to perform real-time analysis allows for immediate sorting decisions, increasing productivity in labs.
- Data Storage and Management: Handling large datasets necessitates effective data management systems, which are essential for subsequent analysis and interpretation.
Data acquisition in FACS is not just about collection, but the accurate interpretation of complex cellular information, laying the groundwork for informed scientific breakthroughs.
In summary, the technological components of FACS are instrumental in pushing boundaries in research. A robust understanding of laser systems, fluorochromes, and data acquisition not only enhances the capabilities of FACS but also underpins its significance in advancing cellular and molecular biology. These components collectively contribute to the innovative application of FACS across a range of studies, reinforcing its importance in modern science.
Types of FACS Sorting Techniques
When diving into the realm of Fluorescence-Activated Cell Sorting (FACS), one cannot overlook the broad category of sorting techniques available. These techniques serve as the backbone for how FACS can be utilized in a variety of applications, including research and therapeutic endeavors. Understanding the various types is paramount for researchers and practitioners alike, as each technique offers unique advantages, challenges, and specialized applications that can significantly impact experimental outcomes.
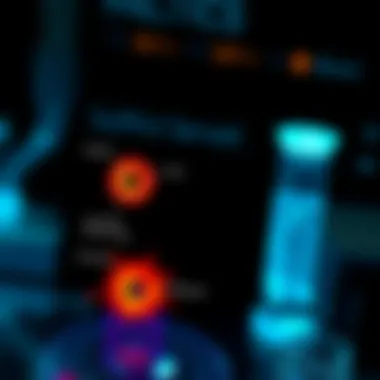
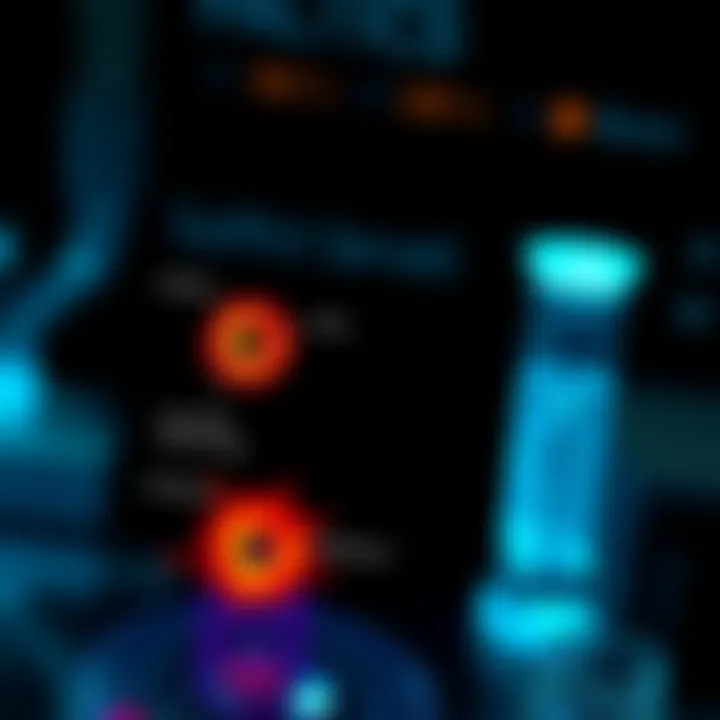
Cell Sorting Types: High-speed vs. Low-speed
Sorting cells can broadly be categorized into high-speed and low-speed methods, each having its own distinct applications and limitations.
- High-speed sorting is particularly beneficial for scenarios requiring rapid processing of a large number of cells. This technique utilizes advanced technology that enables the isolation of thousands or even millions of cells per second. It’s invaluable in applications like large-scale screening assays, where quick results are crucial. However, the downside is that high-speed sorting might generate heat which could potentially affect the viability of certain sensitive cell types.
- Low-speed sorting, on the other hand, offers a different set of advantages. Generally, it operates at a slower rate, focusing on preserving cell functionality and viability. This technique is especially useful when working with delicate cell types or those that need extensive downstream analysis, where the utmost care is essential. The slower pace allows for meticulous sorting, resulting in a highly purified population of cells. This method, while not suitable for all experiments, is often essential for precision work in immunology or developmental biology, where cell integrity is paramount.
"Choosing between high-speed and low-speed sorting ultimately comes down to the specific needs of your research objectives as well as the nature of the cell types involved."
Multi-Parameter Sorting Strategy
The multi-parameter sorting strategy takes FACS to the next level by allowing simultaneous analysis of multiple characteristics of the same cells. This is a significant advancement over single-parameter analyses as it enables researchers to discern more complex profiles of cellular populations. For instance, it can identify not just whether a cell expresses a specific marker, but also measure the intensity of that marker along with several others—such as size, granularity, and additional fluorescent tags.
With this approach, scientists can construct detailed maps of cellular heterogeneity in various environments, which is especially useful in cancer research and immunology. This strategy can lead to breakthroughs in understanding tumor microenvironments or immune responses under different conditions.
Using a multi-parameter sorting strategy helps in:
- Enhanced specificity in identifying rare cell populations.
- Improved data richness, providing a fuller context for understanding cell behavior and interaction.
- More accurate cell sorting, minimizing misclassification errors.
This method, while powerful, does present certain challenges. One must carefully consider the selection of appropriate fluorochromes to avoid overlap, as well as ensure the sorting instrument can handle the increased data load effectively.
Single-Cell Sorting Techniques
Single-cell sorting techniques are a game changer in cellular research, allowing for the isolation and analysis of individual cells rather than larger populations. This level of precision has immense implications for research areas including stem cell biology, cancer studies, and even personalized medicine.
- Advancements in single-cell technologies allow researchers to explore cellular functions like gene expression and metabolic activity with remarkable granularity. Each cell's unique profile can lead to insights that were previously hidden in bulk analyses.
- Applications in systems biology further highlight the importance of single-cell sorting, as it enables the exploration of cellular interactions within their native context, potentially revealing new therapeutic targets.
However, working at the single-cell level also comes with its own set of challenges. The technical complexities involve not only the sorting devices but also analytical methods for processing the resulting data, which can be massively high-dimensional due to the individual-specific characteristics being assessed.
In summary, the landscape of FACS sorting techniques branches out into various approaches, from high-speed to low-speed, to multi-parameter strategies and single-cell applications. Each one plays a crucial role in advancing our understanding of cellular behaviors and paves the way for new discoveries across numerous fields.
Applications of FACS in Research
The applications of Fluorescence-Activated Cell Sorting (FACS) in research play a pivotal role in advancing various biological sciences. By leveraging the unique properties of cells, FACS has transformed the way researchers can isolate and analyze cellular populations. With this method, researchers can dive deeper into understanding cellular functions, interactions, and responses—a leap that is invaluable not just in academic settings but also in clinical applications. The benefits of FACS extend across multiple disciplines such as immunology, oncology, and stem cell research, making it a cornerstone technique in modern biological and biomedical research.
FACS in Immunology
In immunology, FACS serves as a crucial tool in unraveling the complexities of the immune system. It enables the identification and characterization of various immune cell types based on their surface markers. This precise sorting capability allows researchers to track the activation states of T cells or determine the populations of different B cell subsets. Beyond just isolation, FACS also assists in understanding the dynamics of immune responses in health and disease.
- FACS contributes to:
- Vaccine development: By isolating immune cells, researchers can better gauge how vaccines stimulate responses.
- Autoimmunity studies: Analyzing autoantibody-producing cells enhances the understanding of conditions like lupus.
- Infectious disease research: Identifying the immune cells that combat various pathogens provides insights into effective treatment strategies.
In essence, FACS is not just about sorting cells; it's about fostering a deeper understanding of how these cells behave, which is fundamental to immunological research.
FACS in Cancer Research
When it comes to cancer research, FACS plays a pivotal role in identifying and isolating cancer cells. The precision of FACS aids in distinguishing malignant cells from their normal counterparts. This is particularly significant when studying tumors, where heterogeneity among cancerous cells can lead to varying responses to therapies. FACS facilitates the investigation of cancer stem cells, which are often resistant to conventional treatments and contribute to tumor recurrence.
Key applications in cancer research include:
- Tumor immunology: By sorting immune cells infiltrating tumors, scientists can discover how tumors evade immune detection.
- Drug resistance studies: Analyzing cells that survive treatment reveals mechanisms behind cancer resistance.
- Biomarker development: Identifying specific cell surface markers enables the creation of targeted therapies and diagnostic tools.
The depth and breadth of information gleaned from FACS in this field can directly correlate with prognosis and treatment outcomes, illustrating its indispensable role in advancing cancer research.
FACS Applications in Stem Cell Research
In the realm of stem cell research, FACS offers a gateway to isolating specific stem cell populations for further analysis. Given that stem cells can differentiate into various specialized cells, being able to sort these effectively is vital for both basic research and therapeutic applications. For instance, researchers can use FACS to isolate hematopoietic stem cells for studying blood disorders or to characterize pluripotent stem cells used in regenerative medicine.
- FACS enables:
- Single-cell analysis: Allows for the study of gene expression profiles of individual stem cells.
- Characterization of cell surface markers: Identifies subpopulations of stem cells with potential therapeutic properties.
- Tracking differentiation: Researchers can monitor how stem cells convert into different cell types under various conditions.
The precision FACS offers is crucial for refining protocols for stem cell therapy, pushing boundaries in regenerative medicine.
Other Emerging Applications
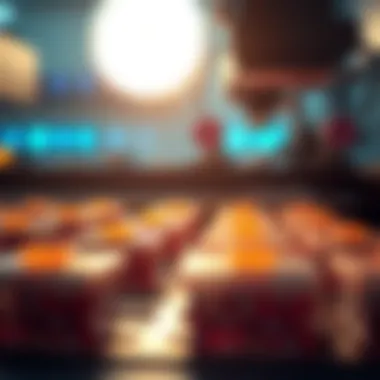
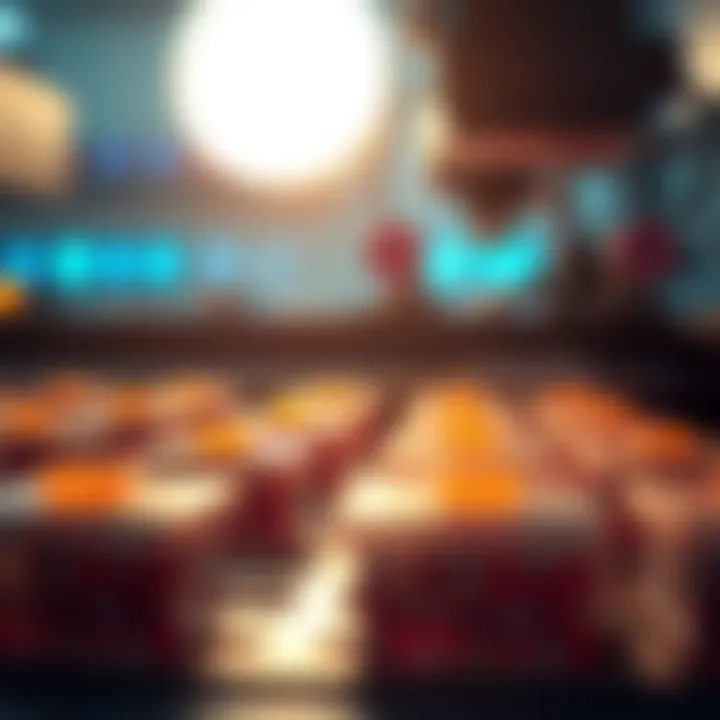
Beyond traditional fields, FACS is seeing emerging applications in other areas of research. For instance, environmental biology has started utilizing FACS to study microbial populations in complex ecosystems. Moreover, its role in genetically modified organism (GMO) research for sorting modified cells shows the adaptability of this technology. Some of the noteworthy applications include:
- Microbial ecology: Enables real-time analysis of specific microbial communities.
- Synthetic biology: Aids in isolating genetically engineered cells to study metabolic pathways.
- Therapeutic monitoring: Used in clinical settings to monitor cellular responses to treatments in real time.
FACS continues to evolve, opening doors to novel research avenues and enhancing our understanding across diverse biological landscapes.
In summary, the applications of FACS are extensive and significantly impact various fields, shaping the future of biomedical research and therapeutic developments.
Challenges and Limitations of FACS
FACS, while widely recognized as a powerful tool for cell sorting and analysis, does not come without its challenges and limitations. Understanding these hurdles is crucial for researchers aiming to maximize the efficacy of this technique. Acknowledging these elements allows for a more informed application of FACS in various scientific endeavors, thereby enhancing its contributions to research in cell biology, immunology, and beyond.
Technical Limitations
In the realm of technical limitations, several concerns can significantly impact the FACS process. For one, hardware constraints often play a major role. High-speed sorting systems can demonstrate suboptimal performance due to limitations in laser intensity and detector sensitivity. If the innards of these machines are not optimized or frequently recalibrated, researchers might find their results yielding inconsistencies.
Moreover, optical limitations present another challenge. FACS systems rely on specific wavelengths of light to excite fluorochromes. If a researcher wishes to use a novel fluorescent marker that falls outside the available laser range on a standard machine, this could hinder the experiment's success. Additionally, the complexity of running multiple parameter analyses can increase both the operating time and the risk of errors during sample preparation.
"No matter how sophisticated the technology, it's only as good as the operator using it."
Another often overlooked factor is the sample viability, particularly when dealing with delicate cell types. Long exposure to lasers can generate heat, potentially damaging cells before analysis even occurs. Furthermore, the sorted cells, especially in high-throughput scenarios, may require specialized conditions that aren't always feasible, posing challenges for post-sort applications such as cultures or further analysis.
Biological Complexity and Variability
The biological complexity and variability that cells exhibit add a layer of difficulty that cannot be ignored. Cells do not exist in a vacuum; they are influenced by numerous factors including their physiological conditions, surrounding microenvironments, and even their interactions with other cells. As a result, the heterogeneity of cell populations can complicate the sorting process. For instance, in immune cells, different subsets may share common markers, thus making separation based solely on fluorescence more challenging.
Additionally, the behavior of cells can fluctuate based on their state at the time of sorting. Factors such as cell health, stage of the cell cycle, or even environmental conditions can cause cells to express markers differently, which further complicates the reliability of FACS. It’s worth noting that multiplying factors like these can lead to significant experimental discrepancies.
Lastly, researchers often need to grapple with the reproducibility of results. Variations in experimental setups or even slight deviations in sample handling can yield entirely different outcomes, adding to the complexities of biological research. For example, two labs might perform FACS on identical samples but obtain varied results due to discrepancies in calibration, environmental conditions or operator technique.
In summary, while FACS is an indispensable methodology in modern science, its technical limitations and inherent biological complexities present notable challenges that researchers must navigate. By being aware of these limitations, scientists can better plan their experiments and interpret their findings with the necessary caution, ensuring that the valuable insights provided by FACS are understood within the context of these challenges.
Future Directions in FACS Technology
As the landscape of cell sorting continues to evolve, the future directions in Fluorescence-Activated Cell Sorting (FACS) technologies present a compelling horizon filled with potential advancements. This section highlights the promising avenues of development, which not only enhances the efficiency of cell sorting but also deepens the understanding of complex biological systems. The importance of these innovations cannot be overstated, as they set the stage for breakthroughs in various fields such as immunology, cancer research, and regenerative medicine.
Innovations in Sorting Technologies
In recent years, the FACS field has seen several groundbreaking innovations that leverage cutting-edge technologies to improve sorting efficacy and accuracy. The advent of novel sorts of lasers, for instance, has increased the detection sensitivity, allowing researchers to sort cells based on a broader spectrum of fluorescent markers. Here are some notable advancements worth mentioning:
- High-throughput Sorting Systems: New sorting systems that utilize advanced optics and fluidics allow separation at unprecedented speeds. For example, systems can now sort thousands of cells per second while maintaining sample integrity.
- Miniaturized Platforms: Researchers are exploring the potential of microfluidic chips that can facilitate cell sorting at a smaller scale. This reduces sample volume requirements and opens up possibilities for point-of-care applications.
- Integration with Artificial Intelligence: The merging of machine learning algorithms with FACS technologies enhances data processing and sorting accuracy. By training models on historical sorting data, AI can predict complex cell behaviors and inform better sorting strategies.
These innovations not only make procedures more efficient but can also lead to finer nuances in research by providing a more comprehensive analysis of cellular populations.
Integration with Genomic Techniques
The cross-pollination between FACS and genomic technologies signifies a transformative leap forward, enabling a more holistic approach to biology. Integrating FACS with genomics opens new avenues for understanding cellular diversity and function. A few elements worth considering include:
- Single-Cell Genomics: As FACS allows researchers to isolate single cells with precision, integrating this with genomic techniques yields unprecedented insights into the genetic expression of individual cells. This approach illuminates heterogeneity within populations and can reveal how cells respond to therapies or environmental changes.
- Multi-Omics Integration: The combination of genomic, transcriptomic, and proteomic data derived from sorted populations provides a multi-faceted view of cellular dynamics. Such holistic datasets can inform on pathways involved in diseases or development.
- Barcoding Techniques: Implementing barcoding in FACS can simultaneously trace multiple cellular characteristics. This assists in associating genotypic information with phenotypic expressions—an invaluable tool in research environments.
In summary, the future of FACS technology is gearing up to be more interdisciplinary, sharper, and efficient. Combining innovations in sorting methodologies with genomic integration fosters a deeper understanding of complex biological phenomena. Researchers need to stay ahead of these developments to harness the full potential of FACS within their studies.
"The journey of science is ever-evolving, and with it, tools like FACS must evolve to not only keep pace but to lead in discovery."
These advancements highlight not just the rapid technical progress but also the shifting paradigms in biological research that demand new methods for cellular analysis.
Ending
In summing up, the exploration of FACS cell sorting has revealed its notable significance and multifaceted roles in the realms of molecular biology and immunology. The advances in this technology are not just stepping stones; they are pivotal in shaping the future of medical research and applications. The ability to sort cells based on specific characteristics provides an unprecedented level of precision that was unimaginable some decades ago.
Summary of Key Points
- FACS operates on principles of fluorescence, using labeled antibodies to identify and isolate distinct cell populations.
- The technology's development over the years signifies a continuous enhancement in sorting efficiency and accuracy.
- Key applications span immunology, oncology, and stem cell research, each benefitting from tailored solutions provided by FACS.
- Emerging innovations, such as integration with genomic analysis, are pushing the boundaries of what FACS can achieve, making it indispensable in contemporary research settings.
It's crucial for researchers and professionals in the field to stay abreast of these advancements as they directly impact therapeutic strategies and experimental methodologies.
The Importance of FACS in Modern Science
FACS has become a cornerstone in modern biological research. Its importance stems not only from its application capabilities but also from the fundamental insights it provides into cellular behavior and interactions.
- Precision: Enables high-throughput analysis of cell populations, allowing for detailed examination of rare cell types.
- Versatility: Applicable in various fields including but not limited to immunology and cancer research, adapting to diverse experimental needs.
- Integration: The synergy with other techniques, such as CRISPR and next-generation sequencing, enhances its utility, enabling groundbreaking discoveries.
"FACS does not merely facilitate cell sorting; it drives the understanding of complex biological systems, allowing scientists to make educated strides toward innovative therapies."