Electrophysiology Techniques: Research and Applications
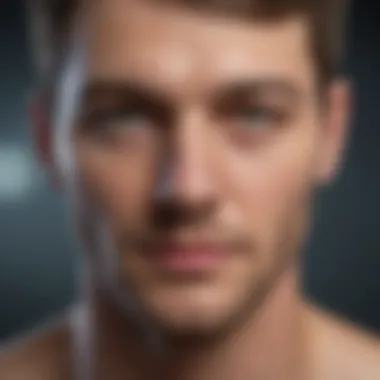
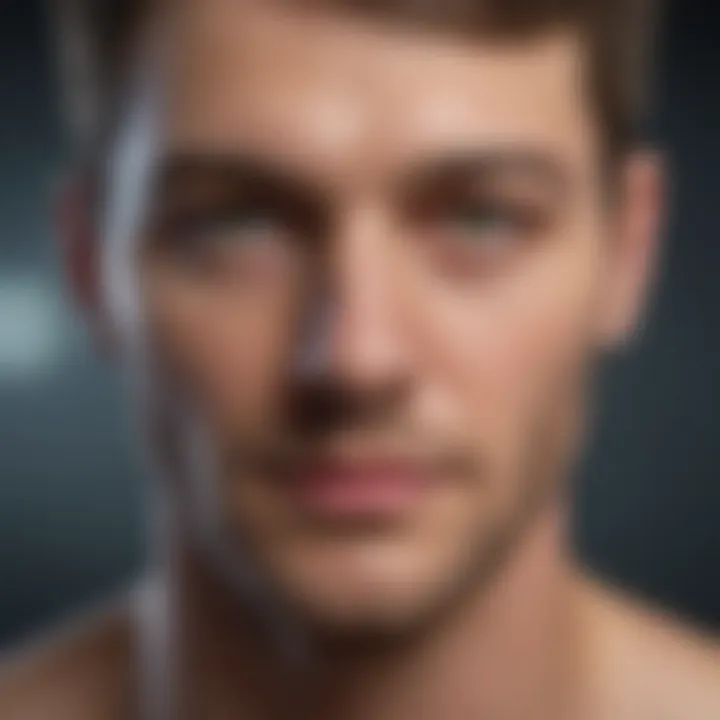
Intro
The field of electrophysiology is rapidly evolving, bringing together a diverse range of techniques used to investigate electrical properties of biological cells and tissues. These methodologies are essential for decoding various physiological mechanisms, especially in neuroscience and cardiology. As the complexity of biological systems increases, so do the techniques that attempt to elucidate their functions. Understanding these techniques is crucial for students, researchers, and professionals who are engaged in areas ranging from basic research to clinical applications.
Overview of Research Topic
Brief Background and Context
Electrophysiology has been a cornerstone of biological research since the late 19th century. Initially rooted in the analysis of action potentials in nerve cells, the field has expanded. Today, research utilizes advancements in technology, such as high-density electrode arrays and optogenetics, to explore intricate cellular dynamics and interactions. This enduring evolution highlights a unique convergence of biology and technology, leading to more sophisticated setups and dynamic analyses.
Importance in Current Scientific Landscape
The relevance of electrophysiology in contemporary scientific research cannot be overstated. The insights gained through these techniques inform not only basic biological principles but also advances in medical diagnoses and treatments. Understanding how cells communicate through electrical impulses helps unravel conditions like epilepsy and heart disease. Moreover, the integration of these techniques into therapeutic approaches, such as in cardiac pacing devices, underlines their significance in practical applications.
Methodology
Research Design and Approach
Investigating electrophysiology requires meticulous research designs tailored to the specific questions being addressed. Typical methodologies include in vitro experiments on cultured cells and in vivo recordings from living organisms. Each design choice is dictated by the nature of the inquiry—whether focused on cellular mechanisms or systemic implications.
Data Collection Techniques
Data collection in electrophysiology employs various techniques, including:
- Patch-Clamp Recording: This technique allows for the measurement of ionic currents flowing through individual ion channels in the cell membrane.
- Electroencephalography (EEG): EEG measures the electrical activity of the brain, providing real-time data on neuronal activity.
- Multi-electrode Arrays (MEAs): These devices can record from numerous neurons simultaneously, offering comprehensive insights into neural network dynamics.
"Electrophysiology is not just about capturing electrical signals. It is about understanding the language of life at the cellular and organismal levels."
In summary, electrophysiology techniques form the foundation for a wealth of discoveries within both basic and applied sciences. They bridge fundamental biology with clinical necessity, making them indispensable for advancing our understanding of life. The ongoing advancements in technology promise even greater insights into the field's future.
Intro to Electrophysiology Techniques
Electrophysiology plays a crucial role in modern scientific research. As a discipline, it investigates the electrical properties of biological cells and tissues. This field has transformed our understanding of various physiological and pathological processes. By employing electrophysiology techniques, researchers can probe into the essence of life at a cellular level, revealing insights about nerve impulses, muscle contractions, and other vital functions. The importance of these techniques cannot be overstated, as they bridge the gap between theory and practical application in multiple areas, including neuroscience, cardiology, and muscle physiology.
One significant advantage of studying electrophysiology is its capability to elucidate the mechanisms underlying cellular behaviors. This encompasses how cells communicate, respond to stimuli, and maintain homeostasis. Moreover, researching electrical signals provides valuable data for developing new therapeutic strategies. As such, it is essential to recognize how these techniques contribute not only to academic knowledge but also to clinical advancements.
In this context, understanding various electrophysiology methods requires an appreciation for both their definitions and their historical evolution.
Defining Electrophysiology
Electrophysiology refers to the study of the electrical activity of biological cells and tissues. This area incorporates techniques that measure voltage changes and ionic currents across membranes. Electrical signals are pivotal in the operation of excitable tissues, such as neurons and muscle cells. At its core, electrophysiology explores how these signals govern physiological functions. Researchers employ a variety of methodologies to assess and interpret electrical activity, ultimately leading to a deeper insight into cellular mechanisms.
One of the fundamental aspects of electrophysiology is its dependency on the behavior of ions, especially sodium, potassium, calcium, and chloride. Their movement in and out of cells generates electrical signals critical for cellular functions like signaling, contraction, and neurotransmission. Through detailed recordings and analyses, scientists can gather information about the excitability of cells, their conduction properties, and the responses to pharmacological agents.
Historical Context
The study of electrophysiology has a rich history extending back to the early experiments in bioelectricity. Pioneering researchers such as Luigi Galvani, who uncovered the electrical implications in frog muscles, laid the groundwork for subsequent discoveries. The advent of the action potential concept in the 1950s was a major milestone, mainly through the work of Alan Hodgkin and Andrew Huxley, who experimentally described how neurons transmit electrical signals. Their introduction of the Hodgkin-Huxley model provided a mathematical framework that continues to influence current research.
Since those early days, electrophysiology has expanded significantly. The introduction of sophisticated techniques and technology, including the patch-clamp method, has allowed for more precise measurements. Over time, the field has amassed a wealth of knowledge, influencing other disciplines and offering insights into various diseases such as epilepsy, cardiac arrhythmias, and neuromuscular disorders. Today, this field remains a vital component of both basic and applied research, continually evolving with advancements in technology and methodology.
"The electrical activity of cells is a fundamental aspect that underpins physiological processes, from heartbeat regulation to muscle coordination."
In summary, the introduction to electrophysiology techniques is more than just a prelude to methods; it offers a framework for understanding how electrical activity shapes life itself.
Core Techniques in Electrophysiology
Core techniques in electrophysiology serve as foundational methods for studying electrical properties of biological cells. Their significance lies in their ability to unravel complex cellular mechanisms and physiological processes. Each technique offers unique insights into the function and behavior of excitable cells such as neurons and muscle fibers. By understanding these core techniques, researchers can deepen their comprehension of biological systems and develop better strategies for both experimentation and application.
Single-Cell Recording
Single-cell recording is a technique that allows for the measurement of electrical activity from individual cells. This is crucial in neuroscience, as it provides detailed data on neuronal firing patterns and synaptic activity. Researchers typically use microelectrodes inserted into single cells to detect action potentials and synaptic currents.
The benefits of this method include:
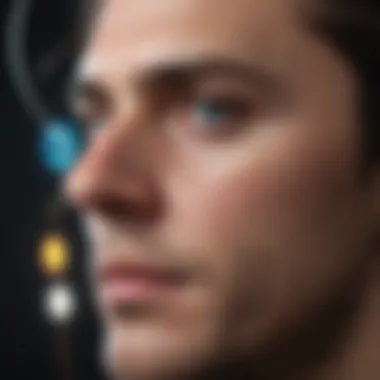
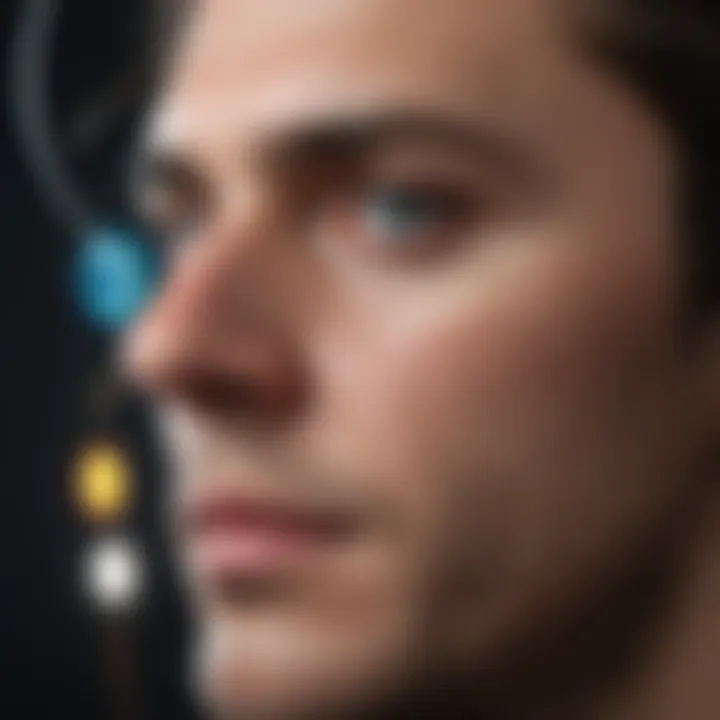
- High temporal resolution: Allows for the capture of rapid changes in electrical activity.
- Specificity: Targets individual cells, providing detailed data without interference from surrounding cells.
However, there are considerations. The technique is labor-intensive and requires skilled practitioners. Also, it may not fully represent the behavior of cells within their native environments.
Patch-Clamp Methodology
Patch-clamp methodology is a refined technique that focuses on the currents flowing through individual ion channels in the cell membrane. This method involves sealing a glass pipette onto a tiny patch of membrane, resulting in precise control and recording of ion flow.
The significance of patch-clamping includes:
- Versatility: Can be used for various types of cells and conditions.
- Quantification: Allows for the detailed analysis of ionic currents and channel activity under different voltage conditions.
Nevertheless, it also has challenges. Maintaining the integrity of the patch can be difficult, and specialized equipment is often needed, which can be costly. These factors may limit accessibility to some researchers.
Multi-Electrode Array Technology
Multi-electrode array technology enables simultaneous recording from multiple cells, allowing for a network-level understanding of cellular behavior. This technique uses a grid of electrodes laid out to capture electrical signals from many cells at once, facilitating the study of complex interactions within cell populations.
Key advantages include:
- High throughput: Can record from dozens to hundreds of cells simultaneously.
- Network dynamics: Supports the study of synchronization and communication between cells.
However, it is important to mention that data interpretation can be more complex due to the large volume of information collected. Signal crosstalk among adjacent electrodes can further complicate analysis.
Understanding these core techniques is essential for advancing research in electrophysiology. Each brings unique capabilities and challenges, reinforcing the complexity of cellular behavior.
By leveraging these core techniques, researchers can uncover vital aspects of physiological functions, contributing to the broader landscape of electrophysiological research.
Analytical Techniques in Electrophysiology
The analytical techniques used in electrophysiology are critical for understanding the electrical properties of biological cells. These methods help researchers elucidate how cells communicate, process information, and respond to various stimuli. By employing precise measurements, analytical techniques can reveal intricate details about cellular excitability and synaptic interactions. This section delves into three key analytical approaches: voltage-clamp techniques, current-clamp approaches, and optogenetics. Each method serves distinct purposes yet contributes significantly to the field of electrophysiology.
Voltage-Clamp Techniques
Voltage-clamp techniques are essential in measuring ionic currents across the cell membrane while controlling the membrane potential. This method allows researchers to achieve a stable voltage and to record how the cell responds to changes in ion concentration or channel behavior.
The primary advantages of voltage-clamp techniques include:
- Precise Control: Researchers can control the membrane potential very accurately, allowing them to study specific ion channel activity in detail.
- Isolation of Ionic Currents: This technique facilitates the isolation of individual ionic currents, thus providing specific insights into the function of different ion channels.
- Contribution to Drug Testing: Voltage-clamp is often used in pharmacological studies to understand how drugs affect ionic currents.
Considerations include:
- Complex Setup: The equipment required can be complex and often demands significant expertise.
- Limitations with Whole-Cell Configuration: In some cases, achieving a proper whole-cell configuration can be challenging, which can affect data quality.
Current-Clamp Approaches
Current-clamp techniques differ significantly from voltage-clamp techniques. Here, current is injected into the cell, and the resulting membrane potential changes are monitored. This method is particularly useful for studying the action potentials of neurons.
The benefits of current-clamp approaches are notable:
- Dynamic Activity Measurement: Researchers can observe how neurons behave under physiological conditions, especially during action potential firing.
- Real-Time Insights: It allows for real-time analysis of neuronal dynamics and synaptic transmission.
- Evaluate Effects of Ion Channels: By manipulating the injected current, one can assess the contributions of various ion channels to the overall membrane potential.
However, some considerations must be taken into account:
- Influence of Passive Properties: The passive electrical properties of the cell can affect the current injections and readings.
- Technical Challenges: Maintaining stable current injection over prolonged periods can also present technical difficulties.
Optogenetics in Electrophysiology
Optogenetics represents a revolutionary technique in the field of electrophysiology. This method uses light to control neurons that have been genetically modified to express light-sensitive ion channels. By shining light on these cells, researchers can modulate neuronal activity with remarkable precision.
Key aspects of optogenetics include:
- Specificity: It provides the ability to target specific cell types within complex tissues, which is often challenging with traditional methods.
- Temporal Precision: Optogenetic control is extremely precise in timing, enabling robust studies of neuronal circuits in real time.
- Integration with Other Techniques: It can be combined with other electrophysiological techniques to enhance experimental design and data collection.
Considerations for using optogenetics:
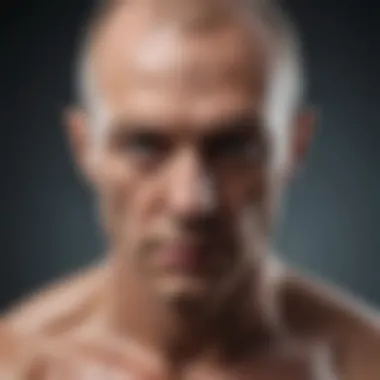
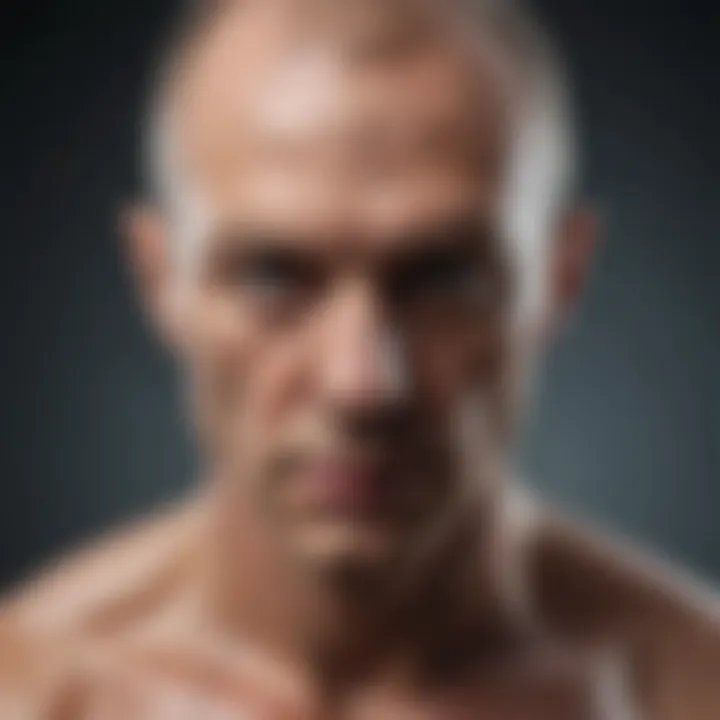
- Genetic Manipulations Required: This approach requires the transfection of specific genes into targeted cells, which can complicate experimental setups.
- Potential Phototoxic Effects: Overexposure to light can lead to phototoxic damage, which can influence data integrity.
"The combination of these analytical techniques revolutionizes how we understand cellular activity in electrophysiology. Each method provides unique insights, ultimately enriching our knowledge of complex biological systems."
Utilizing these analytical techniques in electrophysiology not only enhances experimental understanding but also pushes the boundaries of research in fields such as neuroscience and cardiac physiology.
Applications of Electrophysiology Techniques
Electrophysiology techniques are pivotal in diverse fields of research and application. Understanding their practical implications enhances not only research outcomes but also clinical practices. The ability to record and manipulate electrical signals within biological tissues allows scientists and medical professionals to decipher complex physiological phenomena. From studying nervous system disorders to investigating heart conditions, the applications of electrophysiology are both extensive and profound.
Neuroscience Research
Neuroscience is a principal domain where electrophysiology techniques flourish. These methods enable researchers to explore the intricate workings of neuronal networks. Techniques like single-cell recording provide insights into the firing patterns of individual neurons, revealing how information is processed within the brain. This understanding is crucial in identifying dysfunctions in neurological disorders such as epilepsy, schizophrenia, and Alzheimer’s disease. The intricate details of how specific neurons react to synaptic inputs can lead to the development of targeted therapies. Moreover, advancements like optogenetics offer the opportunity to manipulate neuronal activity using light, facilitating a deeper understanding of circuits that govern behavior.
Cardiac Electrophysiology
Cardiac electrophysiology focuses on the electrical properties of the heart. Techniques in this field are essential for diagnosing and treating arrhythmias and other cardiac conditions. By mapping the electrical activity of the heart using multi-electrode arrays, clinicians can pinpoint irregularities in heart rhythms. The patch-clamp technique also plays a vital role by allowing for precise measurements of ionic currents through individual ion channels in cardiac cells. This detail aids in understanding how different medications affect heart function. The continuous monitoring of electrical signals leads to improving implantable devices like pacemakers, contributing to better patient outcomes and management of cardiac health.
Muscle Physiology Studies
Muscle physiology studies benefit immensely from electrophysiology techniques. By analyzing the electrical signals associated with muscle contractions, researchers can assess muscle health and function. Techniques including current-clamp approaches allow for the observation of action potentials in muscle fibers. This is essential for understanding muscle diseases such as muscular dystrophy. Furthermore, evaluating the electrical activity during various forms of exercise provides insights into muscle adaptation and performance. Such knowledge can guide rehabilitation protocols and athletic training, optimizing performance while reducing injury risks.
In summary, the applications of electrophysiology techniques are integral to advancing multiple scientific and medical fields. The insights garnered from these methods not only enhance scientific knowledge but also pave the way for innovative therapies and enhanced clinical practices. Through rigorous exploration, the true potential of these techniques continues to unfold.
Emerging Trends in Electrophysiology
The field of electrophysiology is undergoing significant transformation driven by advances in technology and innovative methodologies. As researchers strive to enhance their understanding of complex physiological and neurological processes, emerging trends are becoming increasingly influential. These trends not only impact the effectiveness of current techniques but also offer new avenues for research and applications in the future. Understanding these trends is essential for advancing electrophysiological science and maximizing its potential.
Advancements in Imaging Techniques
Recent advancements in imaging techniques play a crucial role in electrophysiology. High-resolution imaging has enabled scientists to visualize cellular activities with unprecedented clarity. This is particularly important for understanding action potentials and synaptic transmissions at the single-cell level. Techniques like two-photon microscopy, for example, allow multiple signals to be recorded simultaneously from various regions within a neuron.
This innovation leads to various benefits:
- Improved spatial resolution: Enhanced imaging quality helps in identifying subcellular structures.
- Real-time monitoring: Allows observation of dynamic changes in response to stimuli, offering insights into physiological processes.
- Multi-modal integration: Combining imaging with electrophysiological recordings provides a holistic view of cellular functions.
As these imaging methods evolve, researchers can unravel the complexities of cellular interactions more effectively, leading to better outcomes in studies related to neuroscience and cardiac health.
Integration of Machine Learning
The integration of machine learning in electrophysiology is revolutionizing data analysis and interpretation. With large datasets generated from electrophysiological experiments, traditional methods of data handling often fall short. Machine learning algorithms can analyze these complex datasets more rapidly and accurately.
Some advantages of this integration include:
- Pattern recognition: Machine learning can identify subtle patterns in electrophysiological signals that may go unnoticed through manual analysis.
- Predictive modeling: Algorithms can predict outcomes based on existing data, enhancing the understanding of modulatory mechanisms in biological systems.
- Improved classification: Automated classification of cell types or signal characteristics increases efficiency in data processing.
Ultimately, the combination of machine learning with electrophysiological techniques is poised to enhance the research landscape, enabling finer analyses and quicker advancements in understanding various biological phenomena.
Challenges Facing Electrophysiology Research
Electrophysiology research sits at a pivotal intersection of advanced technology and fundamental biological inquiry. While there are numerous advantages to employing electrophysiology techniques, the field is not devoid of hurdles. Understanding these challenges is essential for researchers seeking to navigate the complexities of electrophysiological studies. These challenges can hinder progress and affect the applicability of findings in both basic and applied science contexts.
Technical Limitations
Several technical limitations exist in electrophysiology research that can impact results and interpretations. First, the precision of measurements can vary significantly across different techniques. For example, single-cell recordings may produce high-resolution data but may not be scalable to larger tissues or systems. In contrast, multi-electrode array technology allows for simultaneous measurements from many cells, but at the cost of lower spatial resolution.
Moreover, the materials used for electrodes can also affect data accuracy. For instance, glass pipettes used in the patch-clamp method can be sensitive to contamination and may alter the ionic composition of the target cell. Furthermore, the development of artifacts in recordings can occur due to electrical noise from external sources or the biological environment.
Lastly, there are limitations related to the biological systems themselves. Different species or even individual cells can exhibit varied responses to electrical stimuli, complicating the generalization of findings.
"Technical limitations in electrophysiology can lead to inconsistencies that challenge the reproducibility of research outcomes."
Interpretative Complexity
Interpreting data derived from electrophysiological studies involves multifaceted considerations. Firstly, the relationship between voltage changes and cellular behavior is not always linear. This complexity arises due to various factors, such as varied ion channel kinetics and the influence of intracellular signaling pathways.
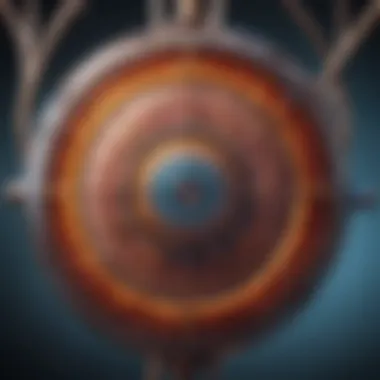
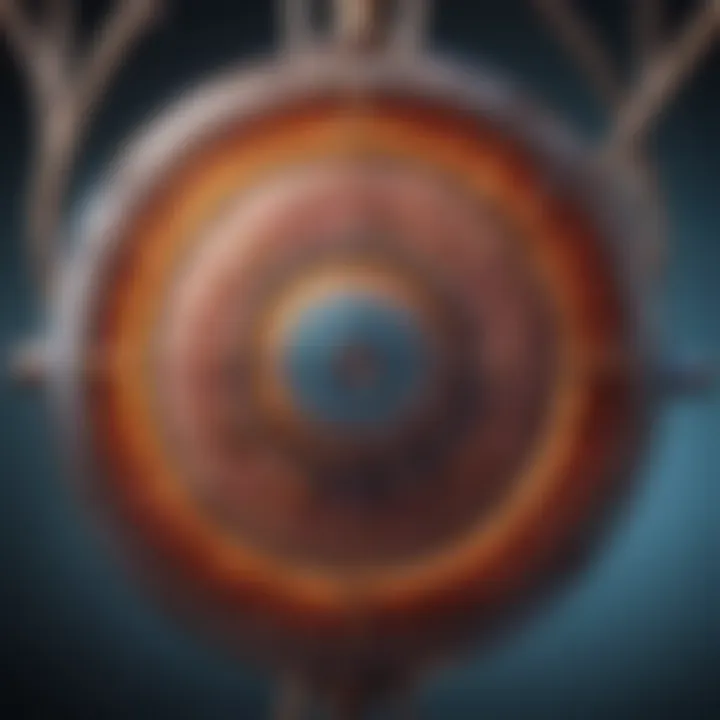
Additionally, researchers often face challenges in distinguishing between correlational and causational relationships. A change in electrical activity may correlate with, but not necessarily cause, alterations in functionality. This requires a comprehensive approach, merging electrophysiology with techniques from other fields such as biochemistry and genetics.
Furthermore, the integration of computational modeling in electrophysiology adds another layer of complexity. While models can simulate biological phenomena, inaccuracies in model assumptions can lead to erroneous conclusions. Thus, researchers must be cautious and critically evaluate their interpretations against empirical data.
In summary, the challenges of technical limitations and interpretative complexity are significant in the realm of electrophysiology research. Addressing these issues requires continued advancements in methodology, as well as a collaborative approach that incorporates insights from multiple disciplines.
Ethical Considerations in Electrophysiology
The study of electrophysiology offers valuable insights into the mechanisms of biological systems. However, it is intertwined with a multitude of ethical considerations that deserve careful examination. These ethics revolve around the treatment of living subjects, including animals and humans, involved in research. Understanding these ethical dimensions is imperative as they shape the approaches and methodologies adopted within the field. The implications range from ensuring the integrity of scientific inquiry to safeguarding the welfare of subjects involved in studies.
Addressing ethical issues is not merely a regulatory obligation but a cornerstone that guides researchers toward responsible conduct. By recognizing these ethical nuances, the scientific community can foster trust from the public while enhancing the quality of research performed in electrophysiology.
Animal Research Ethics
Animal research is a foundational aspect of many electrophysiology studies, particularly in the early phases of experimentation. As researchers seek to understand complex neural and physiological processes, animal models, such as rodents and non-human primates, are often employed. The ethical considerations here include ensuring humane treatment and minimizing pain or distress in these subjects.
To address these concerns, various regulations and guidelines have been established, such as the Three Rs: Replacement, Reduction, and Refinement. This framework encourages researchers to:
- Replacement: Seek alternatives to animal use whenever possible.
- Reduction: Use fewer animals to achieve scientifically valid results.
- Refinement: Employ methods that enhance animal welfare and minimize suffering.
Additionally, Institutional Animal Care and Use Committees (IACUCs) play a critical role by reviewing proposed research protocols. They ensure compliance with ethical standards. By doing so, they aim to balance scientific inquiry with the responsibility to treat animals ethically. This balance is crucial for advancing the field while respecting the inherent value of all living beings.
"The ethical dimension in research transcends mere compliance; it instills a moral responsibility among scientists for their subjects."
Human Subject Research Ethics
When studying electrophysiological principles involving human subjects, ethical considerations become even more pronounced. The complexity of human physiology and the ethical obligation to protect individual rights necessitate strict adherence to ethical guidelines. Informed consent emerges as a critical component.
Participants in human electrophysiology research must be fully informed about the nature, risks, and potential benefits of the study. They should also be free to withdraw consent at any time without facing penalties or adverse consequences. Institutional Review Boards (IRBs) oversee research protocols involving human subjects, ensuring that ethical standards are maintained.
Moreover, researchers must prioritize the confidentiality and privacy of participant data. This ethical obligation not only protects individual rights but also builds trust between researchers and the communities they serve. Consequently, it enhances public willingness to engage in future studies, paving the way for advancements in the discipline.
Future Directions in Electrophysiology
The field of electrophysiology is evolving rapidly, with innovative techniques and applications emerging regularly. Future directions in this area not only provide insights into the current state of research, but also pave the way for advancements that can significantly influence medical and scientific practices. As we look ahead, key elements stand out: interdisciplinary approaches and potential clinical applications.
Interdisciplinary Approaches
Electrophysiology does not exist in isolation. Its future will increasingly depend on collaboration between various disciplines. The integration of fields such as engineering, computer science, and biology will enhance our understanding and capabilities. Here are some aspects to consider:
- Computational Modeling: Utilizing simulation tools can predict the behavior of electrical activities in cells. This can save time and resources in experimental research.
- Bioengineering: Development of new materials and devices will improve recording techniques. For instance, flexible electrodes can provide greater stability with less tissue damage.
- Data Science: Large datasets generated from experiments necessitate advanced analytics. Employing machine learning algorithms can identify trends and insights that may escape traditional analysis methods.
Such interdisciplinary collaborations will result in innovative solutions to complex problems in the realm of electrophysiology.
Potential Clinical Applications
The future of electrophysiology extends into numerous clinical domains, suggesting that its techniques will play an integral role in diagnostics and treatment. The observation and manipulation of electrical signals in the body can lead to significant breakthroughs. Here are some potential applications:
- Cardiac Monitoring: Enhanced electrophysiological methods can improve real-time heart monitoring, resulting in better management of cardiac conditions.
- Neurological Interventions: Techniques like deep brain stimulation may see refined methodologies for treating conditions such as Parkinson's disease or epilepsy.
- Rehabilitation: Understanding muscle electrical activity can improve therapeutic approaches in rehabilitation settings for patients recovering from injuries.
Integrating electrophysiology with clinical practices offers a pathway to enhance patient outcomes
This synchronization between research advancements and clinical needs is vital for the future of healthcare. As these directions unfold, continued adaptation and exploration will be crucial for maximizing the potential of electrophysiology.
Ending
The conclusion serves as a critical capstone to the exploration of electrophysiology techniques. It synthesizes the intricate narratives woven throughout the article, providing a cohesive summary of key insights and implications. By revisiting the core themes, the conclusion enhances the reader's understanding of how these techniques have advanced research and application within various scientific domains. It also highlights the ongoing evolution in the field and the need for continuous adaptation to technological innovations.
Recapitulation of Key Points
In summary, the discussion has brought to light several foundational aspects of electrophysiology techniques:
- Defining Electrophysiology: This involved both its core principles and historical development.
- Core Techniques: Techniques like single-cell recording, patch-clamp methodology, and multi-electrode arrays are crucial for understanding electrical properties of cells.
- Analytical Approaches: Voltage-clamp and current-clamp methods, along with the integration of optogenetics, signify major advancements in data collection and analysis.
- Applications: Key areas such as neuroscience research, cardiac studies, and muscle physiology elucidated the practical significance of these techniques.
- Emerging Trends: The convergence of advanced imaging and machine learning showcases the future direction in this field.
- Challenges: Addressing technical limitations and interpretative complexities remains vital for future studies.
- Ethics: Essential considerations for animal and human subject research ensure compliance with ethical standards.
- Future Directions: Emphasis on interdisciplinary approaches and potential clinical applications points to a forward-looking perspective in electrophysiology.
This recapitulation solidifies understanding and reinforces the transformative impact electrophysiology holds in scientific research.
Looking Ahead
As we consider the future, it is clear that electrophysiology will continue to shape biological sciences. The integration of interdisciplinary methods represents a promising frontier. Collaborations across fields such as biotechnology, computational modeling, and bioengineering can enhance the effectiveness of research. New methodologies will likely emerge, leading to more accurate and nuanced findings.
Furthermore, clinical applications of electrophysiology hold potential for profound impacts on medical practices. Personalized medicine may benefit significantly from insights gained through electrophysiological studies, especially in understanding individual variations in disease mechanisms.