CRISPR Medicine: Transforming Genetic Engineering
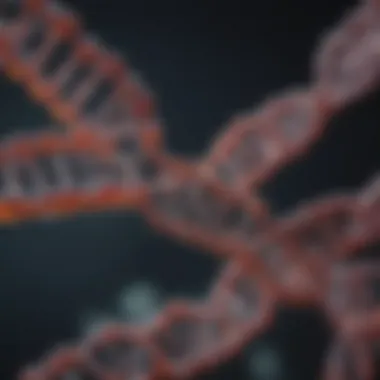
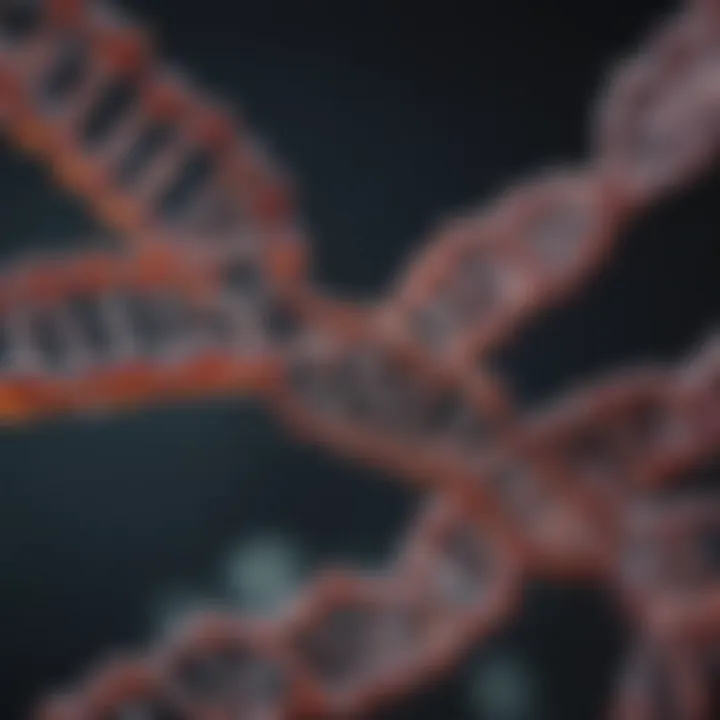
Intro
CRISPR technology represents a profound shift in our understanding of genetics, ushering in new possibilities for gene editing and therapeutic applications. It combines precision with efficiency, making it a powerful tool in modern medicine. This article meticulously details the landscape of CRISPR medicine, focusing on its advancements, applications, and the frameworks surrounding its use.
Overview of Research Topic
Brief Background and Context
CRISPR, an acronym for Clustered Regularly Interspaced Short Palindromic Repeats, is a revolutionary tool for editing genomes. Initially discovered as a natural defense mechanism in bacteria, it has evolved into a sophisticated method for making targeted changes to an organism’s DNA. The advent of CRISPR has led to significant breakthroughs in genetic research, sparking vital discussions about its potential applications in healing genetic disorders.
Importance in Current Scientific Landscape
The ongoing exploration of CRISPR technology is crucial. Current discussions involve not only its medical implications but also the ethical dimensions that arise. With the capacity to alter genetic sequences, CRISPR holds the potential to eradicate hereditary diseases, enhance crop resilience, and even target cancer cells. The implications of such advances require scrutiny, particularly in how they may shape future health policies and societal norms.
"CRISPR is not just a tool for genetic engineering; it is a window into the future of medicine."
Methodology
Research Design and Approach
Research into CRISPR's medical applications often employs a multidisciplinary approach. It integrates genomics, molecular biology, bioinformatics, and ethical studies. By weaving these disciplines together, researchers can develop comprehensive perspectives on CRISPR's potential.
Data Collection Techniques
Data related to CRISPR research is typically collected through various means, including:
- Laboratory experiments to assess gene editing efficacy.
- Clinical trials focused on human applications.
- Surveys and studies in ethics and policy to gauge societal responses.
This blend of qualitative and quantitative data ensures a well-rounded understanding of CRISPR technology and its implications.
Intro to CRISPR Medicine
CRISPR technology has significantly advanced the field of genetic engineering and its applications in medicine. Understanding the essence of CRISPR medicine is crucial to recognizing its potential. This topic opens up discussions about how CRISPR can alter genetic information, leading to innovative therapies for various diseases. Its influence permeates through concepts of precision medicine and tailored treatments that were once deemed unattainable.
The affordability and accessibility of CRISPR methods make it a foundational technology in research laboratories around the world. As researchers continue to perfect these techniques, the implications for human health are substantial. This section lays the groundwork for discussing the mechanics of CRISPR and its broad applications across medical disciplines.
Understanding CRISPR Technology
CRISPR stands for Clustered Regularly Interspaced Short Palindromic Repeats. Initially discovered in bacteria, it serves as an adaptive immune system allowing them to recognize and cut foreign DNA. This natural function has been intricately harnessed for precise genetic editing in organisms, including humans. At its core, CRISPR technology utilizes guide RNA to identify a specific DNA sequence, directing the Cas9 enzyme to cut the DNA at that location. This ability enables scientists to deactivate genes, insert new genetic material, or correct genetic mutations.
The simplicity and versatility of CRISPR have positioned it as a leading tool in genetic research. Furthermore, innovations, such as CRISPR-associated protein 9 (Cas9), have contributed to its appeal. This enzyme essentially acts as molecular scissors, allowing targeted disruption or modification of genes. As the technology evolves, so do its applications in addressing complex health challenges.
The Role of CRISPR in Modern Medicine
The role of CRISPR in modern medicine cannot be overstated. It offers pathways to address previously incurable genetic disorders. The technology provides researchers with the capability to edit genes associated with conditions like Sickle Cell Disease and Cystic Fibrosis, enhancing potential for effective treatments.
Additionally, CRISPR plays a role in cancer research by allowing targeted gene editing. This can result in the development of personalized therapies that cater to the individual genetic makeup of tumors. Its use in immunotherapy is also notable, as it assists in modifying immune cells to better combat cancer.
Furthermore, as we face global health challenges such as infectious diseases, CRISPR presents novel strategies for vaccine development and managing antibiotic resistance.
"CRISPR technology is reshaping the landscape of medical research, providing tools to tackle some of the most complex health issues today."
Overall, the role CRISPR plays in modern medicine presents exciting possibilities, warranting further exploration and understanding in research and clinical applications.
Mechanism of CRISPR-Cas9
The mechanism of CRISPR-Cas9 is essential in understanding how this gene-editing technology functions. This section delves into the core components and processes of CRISPR-Cas9, elucidating its capabilities and applications in genetic engineering. Knowledge of this mechanism provides insight into the precision and efficiency of gene editing, which is vital for advancing medical therapies.
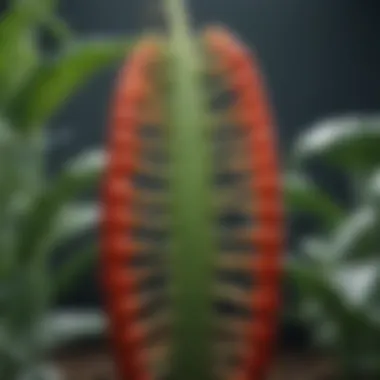
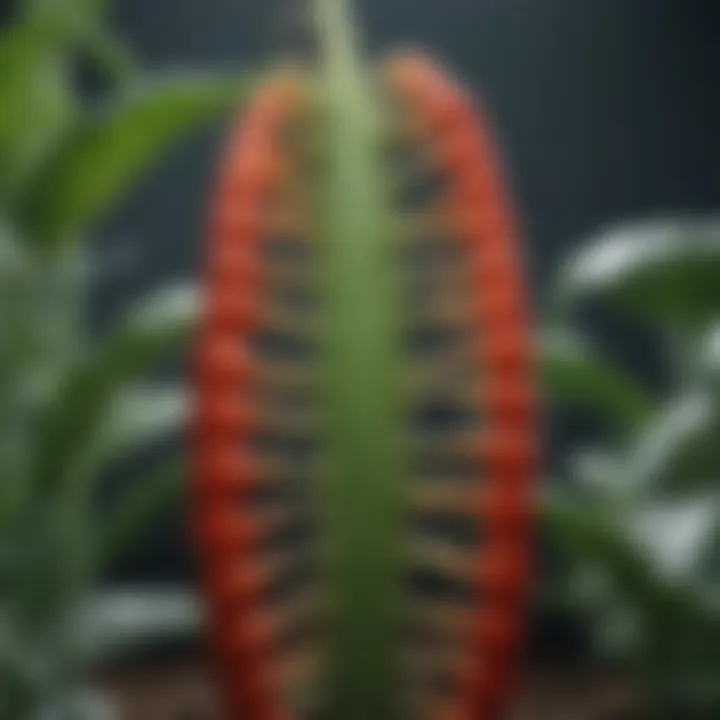
Components of the CRISPR System
Guide RNA
Guide RNA is a fundamental component of the CRISPR-Cas9 system. It serves as a guide that directs the Cas9 enzyme to the specific location of the target DNA. This specificity is achieved through the complementary base pairing between the guide RNA and the target sequence. The key characteristic of guide RNA is its ability to be designed for different target sequences, making it a versatile choice in genetic engineering. Its design flexibility allows researchers to target multiple genes with high precision, which can lead to effective therapies for various genetic disorders.
However, the efficacy of guide RNA can be influenced by factors such as secondary structures, which may affect its binding capability. Sometimes guide RNAs may have off-target effects, where they bind to unintended sites. This can lead to unpredicted consequences in gene editing, highlighting the need for careful design and validation of guide sequences to minimize potential downsides.
Cas9 Enzyme
The Cas9 enzyme is another critical element of the CRISPR system. Its function is to introduce a cut in the DNA at the target site defined by the guide RNA. The key feature of Cas9 is its ability to create double-strand breaks in the DNA helix, which is fundamental for gene editing. It is widely regarded as a beneficial choice due to its efficiency and precision in targeting DNA sequences. Cas9 can work in concert with various types of guide RNAs, enhancing its functionality.
A unique aspect of Cas9 is its requirement for a promoter sequence adjacent to the target DNA, known as a protospacer adjacent motif (PAM). This PAM sequence is essential for Cas9 to recognize and engage the target DNA. While Cas9 offers powerful editing capabilities, there are considerations to be aware of, such as potential immune responses in mammalian systems when utilizing bacterial proteins. Understanding these factors is crucial for optimizing CRISPR applications.
Target DNA
Target DNA forms the basis for the CRISPR-Cas9 system's application. It is the DNA sequence that the system aims to modify or edit. The key property of the target DNA is its specificity; only the sequences that match the guide RNA will be affected by the Cas9 enzyme. This characteristic ensures that the CRISPR system can edit precise regions, crucial for effective genetic therapies.
One advantage of targeting specific DNA sequences is the ability to disrupt harmful genes or introduce beneficial edits. However, the exact identification of target DNA is critical, as unintended edits to off-target locations can lead to unwanted mutations. Continued research is necessary to further understand how to maximize specificity while minimizing potential risks in gene editing applications.
How CRISPR-Cas9 Works
Understanding how CRISPR-Cas9 works provides insight into its mechanics and operational steps. The ability to identify and modify target DNA is a crucial aspect of using this technology in various applications, including research and clinical settings.
Target Identification
Target identification is a crucial step in the CRISPR-Cas9 process. It involves recognizing the specific sequence of target DNA that the guide RNA will bind to. This characteristic of precise target identification is essential for ensuring that modifications occur at the intended location. The ability to design guide RNAs that match the target sequence enhances the effectiveness of CRISPR in gene editing.
One benefit of accurate target identification is the reduced chance of off-target effects, which can lead to unanticipated outcomes in gene modification. However, the development of high-fidelity guide RNAs requires considerable effort in design and screening to achieve reliable results.
DNA Cutting
DNA cutting is the action where the Cas9 enzyme induces a break at the identified target site. The ability to perform precise cuts is what makes CRISPR-Cas9 a revolutionary tool in genetic engineering. This characteristic allows scientists to know exactly where to edit the genome.
The effectiveness of DNA cutting can lead to various repair pathways being triggered in the cell. There may be challenges related to the efficiency of the cut, as some target sequences can be hard to access due to chromatin structure. Variations in cellular context can also affect the outcomes of the cutting step, making it an area of ongoing research in the field.
Repair Mechanisms
The repair mechanisms that follow the DNA cutting play a significant role in determining the outcome of CRISPR-Cas9 interventions. After the double-strand break is introduced, the cell attempts to repair the damage. This step can lead to various results, such as gene knockout or the introduction of new genetic material.
A key feature of repair mechanisms includes pathways like Non-Homologous End Joining (NHEJ) and Homology Directed Repair (HDR). NHEJ is often quicker but may result in imprecise edits due to insertions or deletions. On the other hand, HDR can allow precise repairs if a donor template is provided.
However, the reliance on the repair mechanisms can vary significantly based on the type of cells and the conditions present. Errors in the repair process can have significant implications, emphasizing the need for methods to optimize these outcomes for successful gene editing.
Applications of CRISPR in Medicine
CRISPR technology has opened new frontiers in medical science. Its applications range from treating genetic disorders to combatting cancer and managing infectious diseases. The power of CRISPR lies in its ability to target and modify specific genes, potentially correcting mutations that cause diseases. This section will delve deep into sector-specific applications, outlining key examples and their implications for patient care and public health.
Genetic Disorder Therapies
Genetic disorders pose a significant challenge to healthcare systems worldwide. These conditions, often lifelong, stem from mutations in genes that disrupt normal cellular function. CRISPR's precision enables targeted interventions that can reverse these genetic defects.
Sickle Cell Disease
Sickle cell disease is a hereditary blood disorder characterized by malformed red blood cells. These cells can become stuck in small blood vessels, causing pain and organ damage. Current treatments mainly focus on pain management and blood transfusions. CRISPR presents a promising option by enabling gene editing that could correct the defective gene responsible for this condition. This capability showcases CRISPR's potential to alter the life course of patients. The main advantage is its ability to offer a more permanent resolution as opposed to merely managing symptoms.
Cystic Fibrosis
Cystic fibrosis is another genetic disorder where the mutation affects lung function, leading to severe respiratory complications. Research using CRISPR aims to correct the CFTR gene mutation, which is fundamental for lung health. By repairing the faulty gene, the chances of normal lung function can significantly increase. The uniqueness of this application lies in its potential to directly address the root cause of the disease rather than just alleviating symptoms. However, ethical considerations are also paramount in ensuring that any gene modification carried out is safe and beneficial long-term.
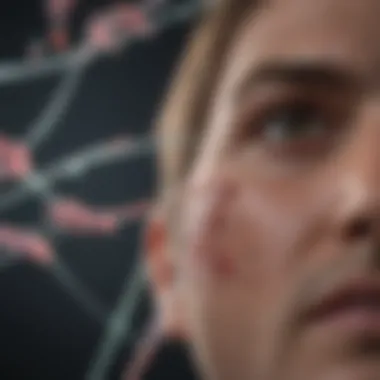
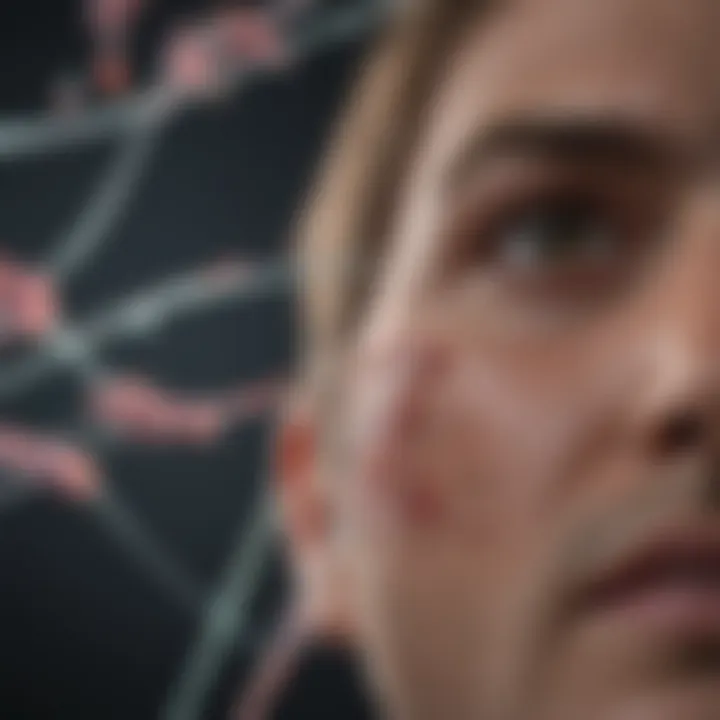
Muscular Dystrophy
Muscular dystrophy comprises a group of genetic disorders that lead to progressive weakness and loss of muscle mass. Certain forms, such as Duchenne muscular dystrophy, are caused by mutations in the dystrophin gene. CRISPR's ability to modify this gene can lead to increased production of dystrophin in muscle tissues, potentially altering the disease's trajectory. This therapy holds substantial promise as it targets the specific gene responsible for the condition, offering hope of improved quality of life for patients. Nevertheless, challenges remain in terms of delivery methods and ensuring effective editing of all affected cells.
Cancer Research and Treatment
Cancer remains one of the most formidable challenges in modern medicine. The heterogeneity of tumors complicates treatment, making targeted therapies particularly appealing.
Targeted Gene Editing
Targeted gene editing using CRISPR allows for the modification of cancer cells specifically. By identifying pathways and mutations that contribute to tumor growth, researchers can design treatments that directly attack these vulnerabilities. This approach is a significant advancement in personalized medicine, improving efficacy while reducing collateral damage to healthy cells. Its benefits are numerous; however, careful consideration of off-target effects and the long-term consequences of such modifications is critical.
Immunotherapy Enhancements
Immunotherapy has transformed cancer treatment by harnessing the body's immune system to combat tumors. CRISPR can enhance this field by enabling more effective modifications to CAR T-cell therapies. By utilizing CRISPR to refine the characteristics of T-cells, researchers can increase their efficiency in targeting cancer cells. This synergy between CRISPR and immunotherapy embodies a promising intersection that might lead to better patient outcomes. On the downside, it requires rigorous testing and validation to ensure safety and effectiveness before widespread use.
Infectious Disease Management
Infectious diseases pose a constant threat to global health. CRISPR's role extends to fighting viral infections and antibiotic resistance, representing a crucial frontier in medical research.
Viral Infections
Viral infections remain a leading cause of morbidity worldwide. CRISPR technology can be tailored to target and cut specific viral DNA within infected cells. This method could lead to innovative antiviral strategies, effectively eradicating viruses such as HIV or HPV from the body. One major advantage is its precision; however, considerations regarding delivery mechanisms to effectively reach infected cells must be addressed.
Antibiotic Resistance
Antibiotic resistance has emerged as one of the critical issues confronting contemporary medicine. It renders conventional treatments ineffective, leading to higher rates of mortality. Through CRISPR, researchers are exploring modifications that could potentially revive the efficacy of existing antibiotics by targeting specific resistance genes in bacteria. The unique feature of CRISPR in this context is its capacity to edit genes responsible for resistance, thus restoring the effectiveness of antibiotics. That said, it must be stressed that thorough investigation is required to prevent unintended consequences on the microbial ecosystem.
In summary, the applications of CRISPR in medicine are vast and versatile. From genetic disorders to cancer therapies and infectious disease management, the potential for transformative change underscores the necessity for further research and ethical considerations in implementing these technologies.
Ethical Implications of CRISPR
The advent of CRISPR technology heralds a new era in genetic engineering, especially within medicine. However, with such revolutionary power comes significant ethical implications. Understanding these implications is critical to shape the future of CRISPR applications. The considerations envelop a broad spectrum of factors, affecting not only scientific research but also societal norms and moral frameworks.
Concerns Over Genetic Modification
One primary concern regarding CRISPR technology is genetic modification. This raises questions about the extent to which scientists should alter human genes. The potential for creating so-called “designer babies” is particularly contentious. While some propose that parents could genetically enhance their children, others warn of unintended consequences. Genetic modifications can lead to unforeseen health effects that may follow through generations.
Furthermore, there is the issue of equity. Access to genetic modifications could be limited to those with financial resources, creating a socio-economic divide in healthcare outcomes. This disparity ignites debates on justice and fairness within society.
Some ethical frameworks advocate for caution due to the complexity of genetic interactions. Every gene interacts with numerous other genes and environmental factors. A single modification can alter an individual's overall health in unpredictable ways. Understanding these interactions is essential before making any permanent changes to the human genome.
Societal and Moral Challenges
The societal implications of CRISPR cannot be understated. Public perception of genetic engineering plays a vital role in its acceptance. Many individuals express anxiety about potential misuse of the technology. This includes fears regarding bioengineering and eugenics. These fears stem from historical abuses of genetic science and the potential for CRISPR to be exploited for unethical purposes.
Moreover, moral dilemmas arise surrounding consent, particularly for germline editing. Since these changes affect future generations, gaining informed consent becomes problematic. Future offspring cannot voice their opinion regarding genetic modifications made before their existence.
In addition, there is a risk of commodifying human life. If genetic traits can be purchased, it raises questions about the value of individual lives and the potential loss of diversity. Emphasizing certain traits over others could lead to cultural homogenization.
"Genetic engineering represents the intersection of limitless possibilities and ethical boundaries."
Thus, while CRISPR technology offers extraordinary potential, a balanced dialogue surrounding ethical considerations is essential. Societal engagement and rigorous examination of moral principles are necessary to ensure the responsible advancement of CRISPR applications in medicine. Future policies must consider various perspectives to foster an ethical framework guiding this powerful technology.
Regulatory Frameworks Surrounding CRISPR
Regulatory frameworks surrounding CRISPR are crucial in structuring the safe and ethical application of gene editing technologies. As the capabilities of CRISPR expand, so does the need for stringent oversight. These regulations help ensure that actions taken in the name of science do not adversely affect human health or biodiversity. The balance between innovation and caution must be maintained to support public trust in gene editing applications.
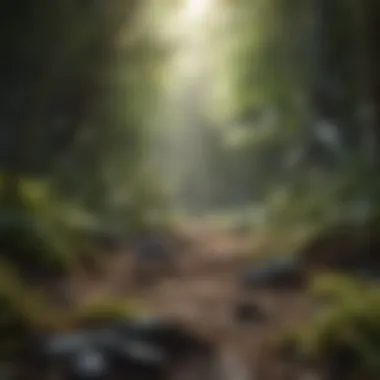
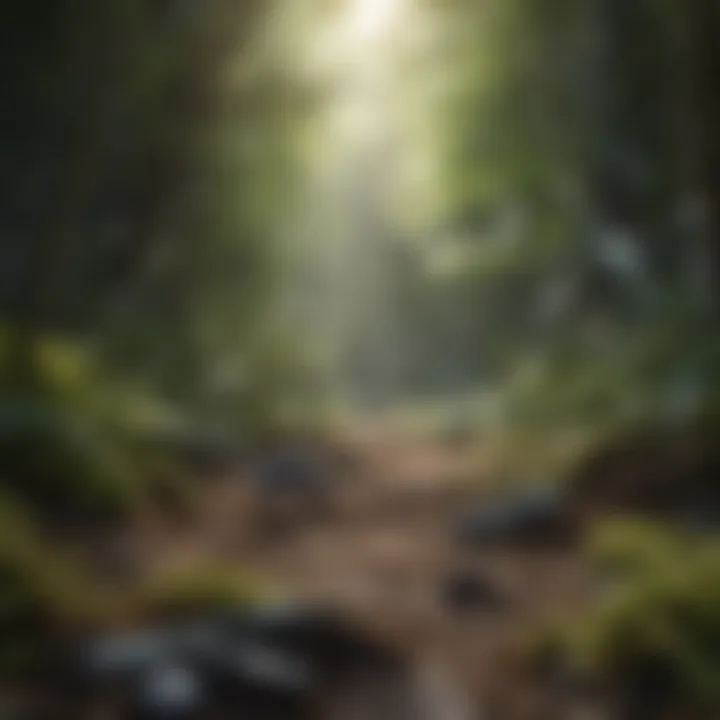
Current regulations often focus on two main areas: safety and efficacy. They aim to establish guidelines that govern how gene editing can be performed, both in laboratories and in clinical settings. Specific regulations may differ significantly across regions and countries, highlighting the necessity for a coherent approach internationally. Given that CRISPR can alter germline cells, which may affect future generations, the stakes are particularly high.
Current Regulations in Gene Editing
In the United States, the Food and Drug Administration (FDA) plays a pivotal role in regulating gene editing technologies. The agency evaluates the safety and effectiveness of any new therapies before they can be approved for human use. Similarly, the National Institutes of Health (NIH) oversees federally funded research involving human subjects, ensuring compliance with ethical standards.
In Europe, regulations are generally more stringent. The European Medicines Agency governs the approval process for gene therapies. The principles of the EU's General Data Protection Regulation (GDPR) also apply, especially concerning data privacy when human genomics is involved.
"Gene editing poses unique ethical questions concerning the limits of human intervention in natural processes."
Moreover, many countries restrict germline editing entirely. Some frameworks prioritize transparency, requiring all research involving CRISPR technologies to be published and made accessible. This is essential to foster understanding and accountability in the scientific community and to the public.
International Perspectives
The global landscape of CRISPR regulations varies widely. In countries like China, there has been rapid advancement in gene editing technology without a comprehensive regulatory framework. This has led to ethical concerns, especially regarding the use of CRISPR in humans. On the other hand, nations like Germany impose a complete ban on germline editing, reflecting a conservative approach towards biotechnology.
Collaborations across borders are becoming increasingly important for establishing common standards and regulations. The World Health Organization and other international bodies are working on guidelines that may assist in harmonizing regulations. The importance of international cooperation cannot be overstated, as gene editing consequences do not respect national boundaries.
Ultimately, the regulatory landscape must evolve continually to meet the challenges posed by advancing technologies. Scientists, policymakers, and ethicists need to work together to adapt existing frameworks, ensuring that the benefits of CRISPR are harnessed responsibly while safeguarding societal values.
Future Directions for CRISPR Research
The future of CRISPR research holds immense potential, shaping how we approach genetic medicine. As the technology continues to advance, its implications extend beyond the lab, affecting healthcare, ethics, and regulatory policies. Understanding these future directions is vital for students, researchers, and healthcare professionals. This section explores emerging technologies, the anticipated long-term impact on healthcare, and the key collaborations that will drive innovations in CRISPR applications.
Emerging Technologies in Gene Editing
Emerging technologies in gene editing are enhancing the capabilities of CRISPR. New systems and techniques, such as CRISPR/Cas12 and CRISPR/Cas13, provide more precise and efficient gene modifications. These systems react differently, allowing for more targeted interventions in various genetic diseases. For example, Cas12 exhibits greater robustness and specificity, reducing off-target effects that have limited earlier versions of CRISPR.
- Another critical advancement is the development of base editing, which allows for the direct conversion of one DNA base to another without creating double-strand breaks. This advancement minimizes potential errors and enhances safety in gene therapy applications.
- Prime editing is another innovative approach that improves accuracy in gene editing. It employs a catalytically inactive Cas9 combined with an engineered reverse transcriptase to insert or delete specific sequences with remarkable precision.
These technologies demonstrate the trend towards increasing precision and versatility in gene editing. This progress not only increases the potential applications in medicine but also makes the technology safer and more reliable for clinical use.
Long-Term Impact on Healthcare
The long-term impact of CRISPR on healthcare is profound. Gene editing could revolutionize treatment options for genetic disorders, providing potential cures rather than symptomatic treatments. Many diseases that currently have no effective therapies may become manageable through these advanced techniques.
- Genetic disorders like sickle cell disease and muscular dystrophy may be treated at the genetic level, addressing the root cause rather than merely alleviating symptoms. This could lead to significant improvements in patients’ quality of life.
- In addition, CRISPR's role in personalized medicine is increasingly recognized. Tailoring treatments based on individual genetic profiles may lead to more effective and targeted therapies, ultimately improving patient outcomes.
- The potential of CRISPR in cancer treatment is equally notable. Research into how gene editing can modify immune responses to better fight tumors could redefine cancer therapy as we know it.
However, this transformative potential comes with challenges. Ethical considerations and regulatory approval processes will need to evolve alongside the technology, ensuring that safety and efficacy are prioritized.
Collaborations and Innovations
Collaborations are crucial for advancing CRISPR research. When academic institutions partner with biotech companies, the potential for innovation increases significantly. This synergy fosters the development of new therapies and expedites the translation of research from the lab to clinical settings.
- Collaborative efforts can leverage diverse expertise, combining knowledge in genetics, bioinformatics, and regulatory affairs to harness CRISPR's full potential.
- Global collaborations are equally essential. Sharing research findings and technologies across international borders can accelerate the pace of discovery while promoting ethical standards in gene editing.
"Partnerships in the scientific community can drive progress like never before, enabling breakthroughs that would be difficult to achieve in isolation."
Furthermore, initiatives focusing on public engagement and education about gene editing can also facilitate informed discussions, paving the way for acceptance and integration of these technologies into healthcare frameworks. Research institutions and companies should focus on building trust with the public to ensure responsible and ethical deployment of CRISPR solutions.
End
The importance of the conclusion within this article lies in its capacity to encapsulate the main findings regarding CRISPR technology and its implications for medicine. It offers a succinct overview, reaffirming the expectation that CRISPR has the potential to reshape the landscape of genetic engineering. This section reinforces the critical place CRISPR occupies in both current medical research and future applications.
Summary of CRISPR's Impact
In recent years, CRISPR technology has emerged as a powerful tool for genetic modification. Its ability to edit genes precisely allows for groundbreaking applications in medicine. This includes developing therapies for genetic disorders like sickle cell disease and cystic fibrosis. CRISPR is also enhancing cancer treatment through targeted gene editing and the improvement of immunotherapies. The impact is not limited to inherited conditions; CRISPR also addresses infectious diseases and tackles challenges like antibiotic resistance.
The efficiency and versatility of the CRISPR-Cas9 system distinguish it from previous gene-editing methods. With a clearer understanding of gene functions, CRISPR paves the way for innovative medical solutions.
The Path Ahead for CRISPR Medicine
Looking forward, the future of CRISPR medicine appears promising yet complex. Emerging technologies, such as CRISPR 2.0, show potential for improved gene editing accuracy. Research is continuously evolving, suggesting that CRISPR could lead to advanced treatments and potentially cure previously untreatable diseases. However, this progress does not come without challenges. Ethical considerations will remain at the forefront of discussions as the technology advances.
There is an urgent need for collaboration among researchers, regulatory bodies, and ethicists. These partnerships will be essential to navigate the complexities of gene editing responsibly. As CRISPR continues to transform the healthcare landscape, the importance of adhering to ethical principles cannot be overstated. The advancements in CRISPR have the potential to revolutionize medicine, but the path ahead must be tread carefully.