Crispr KO Screen: Unveiling Genomic Innovations
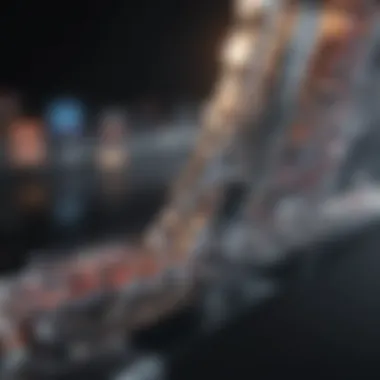
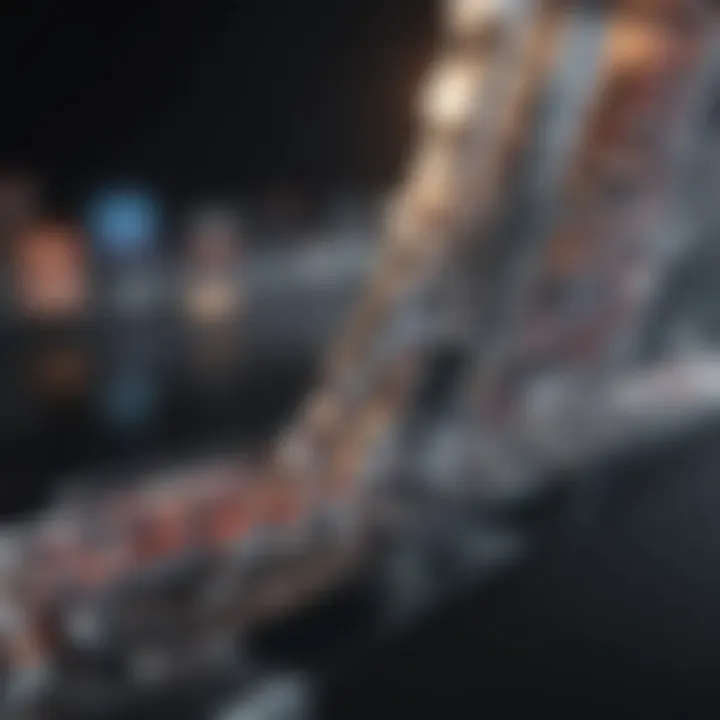
Overview of Research Topic
Brief Background and Context
CRISPR technology has revolutionized the field of genomics since its discovery. The system initially recognized for its role in bacterial immunity has pivoted into a powerful tool for genome editing in diverse organisms. One significant application of CRISPR is the knockout (KO) screen, which allows for the systematic analysis of gene function. This method involves disabling specific genes to observe resulting phenotypic changes, thus providing invaluable insights into gene roles in various biological processes.
Importance in Current Scientific Landscape
The significance of CRISPR KO screening lies in its capacity to enhance our understanding of gene functionality and its implications in diseases. In the contemporary scientific landscape, this technique is essential for identifying potential therapeutic targets in numerous conditions, including cancer and genetic disorders. With the advent of CRISPR, researchers can now perform high-throughput screenings that were previously time-consuming and resource-intensive. This efficiency offers a new level of precision in functional genomics, contributing to the ongoing quest for knowledge in molecular biology and medicine.
Methodology
Research Design and Approach
To conduct CRISPR KO screens, researchers typically design a library of guide RNAs (gRNAs) that correspond to target genes of interest. These gRNAs are introduced into a population of cells along with components necessary for the CRISPR-Cas9 system. The design is critical since it determines the effectiveness of gene disruption. Once integrated into the cells, the gRNAs direct the Cas9 endonuclease to the specific genomic loci, inducing double-strand breaks and leading to gene knockout.
Data Collection Techniques
After the knockout process, researchers employ various techniques to assess the impact on cellular functions. These can include:
- Next-Generation Sequencing (NGS): This method allows for the comprehensive analysis of the gRNA sequences present in the surviving cell population, providing insight into which genes were effectively knocked out.
- Flow Cytometry: Used for analyzing cell populations, this technique can help quantify phenotypic changes following gene disruption.
- Functional Assays: Assays tailored to measure specific cell behaviors, such as proliferation or apoptosis, further elucidate the biological significance of the knocked-out genes.
CRISPR KO screening is at the forefront of genomic research, unlocking new pathways to understand gene impacts in health and disease.
The combination of these methodologies establishes a framework that not only improves the efficiency of genetic research but also expands the scope of functional genomics, paving the way for future innovations in biology and medicine.
Foreword to CRISPR Technology
CRISPR technology represents a transformative advancement in genetic editing. This section introduces the foundational principles of CRISPR, making it essential for understanding its application in knockout (KO) screening. Knowledge of CRISPR technology is vital for grasping the implications of gene editing in research, medicine, and beyond. The ability to edit genes with precision has far-reaching impacts on functional genomics and therapeutic interventions.
Overview of CRISPR Mechanism
CRISPR, which stands for Clustered Regularly Interspaced Short Palindromic Repeats, functions as an adaptive immune system in bacteria. Its mechanism centers around RNA-guided enzymes, particularly Cas9. The process begins with the formation of a guide RNA (gRNA) that identifies specific sequences in the genome. Once the gRNA pairs with the target DNA, the Cas9 enzyme introduces a double-strand break at the specified location. This targeted activity allows researchers to disrupt gene function or facilitate genome editing, creating the basis for various applications, including KO screens.
CRISPR technology simplifies gene editing, allowing for precise disruptions without altering surrounding sequences.
The significance of this mechanism lies in its specificity and efficiency compared to earlier gene-editing methods. It empowers scientists to explore gene functions systematically and elucidate biological pathways, fostering advancements in various fields such as oncology, neurology, and developmental biology.
Historical Context of CRISPR Development
The discovery of CRISPR technology dates back to the early 2000s. Initial research unveiled the complex structure of CRISPR sequences in bacterial genomes; however, its application for genetic editing would not emerge until landmark papers published in 2012. Jennifer Doudna and Emmanuelle Charpentier demonstrated that CRISPR can be repurposed as a gene-editing tool. This pivotal moment sparked an explosion of interest in gene editing, establishing a new era in molecular biology.
Since then, numerous studies have refined the techniques surrounding CRISPR technology. The evolution of applications has included not just KO screens but also transgenics and base editing. As CRISPR technology continues to develop, it presents an array of possibilities that challenge the boundaries of biological research and medicine.
The implications of CRISPR extend beyond laboratory exploration. They raise ethical considerations about gene editing in humans and the environment, emphasizing the need for responsible usage and regulatory measures as the technology progresses.
Understanding Knockout Screens
The topic of knockout screens plays a central role in the exploration of CRISPR technology. These screens allow researchers to systematically disrupt genes in order to study their functions. Understanding knockout screens is vital for several reasons. First, they enable scientists to unravel complex biological systems by identifying genes responsible for particular traits or functions. This approach is especially important in functional genomics, where the focus is on understanding gene function rather than simply sequencing genes.
Moreover, knockout screens facilitate the study of gene interactions and regulatory networks. By disrupting specific genes, researchers can observe the resulting changes in phenotypes, leading to insights about cellular pathways and disease mechanisms. This knowledge has potential applications in drug discovery and precision medicine, making knockout screens a cornerstone of modern molecular biology.
Definition and Purpose of Knockout Screens
Knockout screens are powerful experimental techniques used to identify the function of genes by completely disabling them. The primary purpose is to understand how the absence of a gene affects cellular functions and organismal traits. In a CRISPR knockout (KO) screen, guide RNAs target specific genes for disruption, allowing researchers to observe the resultant phenotypes or alterations in cellular behavior.
This technique is particularly useful in identifying genes associated with specific diseases, including cancer and genetic disorders. The ability to systematically knockout genes in a high-throughput manner accelerates the discovery process, providing critical information for both basic and applied research.
Comparative Techniques
While CRISPR technology is a leading method for conducting knockout screens, it is essential to understand other comparative techniques that have been developed over the years.
Zinc Finger Nucleases
Zinc Finger Nucleases (ZFNs) are an earlier genome-editing tool that serves as a forerunner to CRISPR. These proteins combine a zinc finger DNA-binding domain with a nuclease that introduces double-strand breaks in specific DNA sequences. Their main advantage is precision in targeting, which is crucial for reliable gene editing. However, the design of ZFNs is often complex and time-consuming, which can limit their practical use in high-throughput settings.
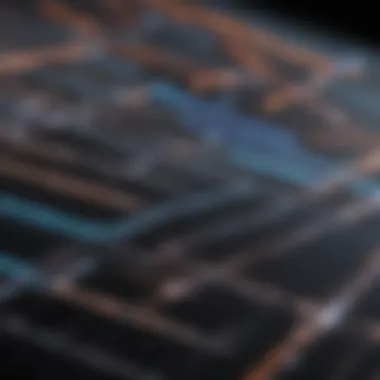
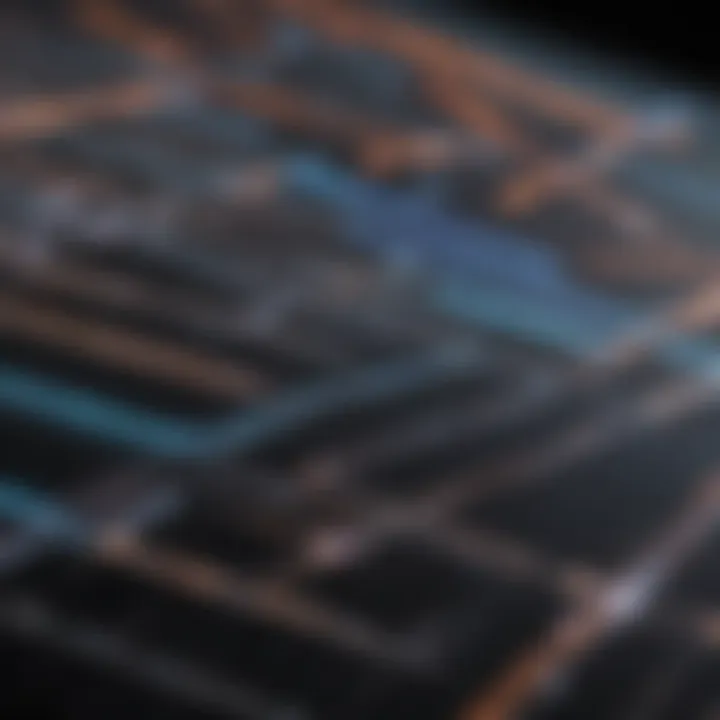
ZFNs can elicit off-target effects, but their specificity can be increased with careful design, making them a reliable choice for knockout applications when optimized.
TALENs
Transcription Activator-Like Effector Nucleases (TALENs) are another genome-editing tool utilized for knockout screens. They work similarly to ZFNs, but their design is based on TALE proteins that can recognize specific DNA sequences. TALENs are often praised for their flexibility in targeting various sequences, making them user-friendly for researchers with diverse needs.
However, creating TALENs can also be labor-intensive and might not always achieve the efficiency seen with CRISPR. Despite their limitations, TALENs remain a valuable tool for gene manipulation, providing a robust method for knockout initiatives.
In summary, while CRISPR is becoming the preferred technique for knockout screens, understanding the advantages and disadvantages of ZFNs and TALENs enriches researchers' toolkit for gene editing approaches.
Designing a CRISPR KO Screen
Designing a CRISPR knockout (KO) screen is a critical step that shapes the outcomes of genomic research. This phase involves careful consideration of various elements, which directly influence the effectiveness, accuracy, and relevance of the experiment. A well-designed CRISPR KO screen allows researchers to pinpoint specific gene functions and interactions. Thus, the importance of this topic cannot be overstated. It lays the foundation for achieving meaningful and reproducible results within functional genomics.
Selection of Target Genes
The selection of target genes is a pivotal element in the design of a CRISPR KO screen. Researchers must choose genes that are relevant to the biological questions they seek to answer. The initial approach often involves literature review and bioinformatics analysis to identify genes that may have a specific role in a disease pathway or biological process.
Consider the following factors when selecting target genes:
- Biological Relevance: The chosen genes should have known connections to the phenotype or process of interest.
- Gene Accessibility: Genes should have suitable targeting options available without causing off-target effects.
- Viability Considerations: Prioritize genes for which knockout does not lead to lethality or severe fitness loss, unless specifically studying essential genes.
Additionally, developing a comprehensive list involves cross-referencing various datasets, including gene expression profiles and existing KO studies. This practice enhances the chances of success in downstream analyses.
Guide RNA Design Considerations
Guide RNA (gRNA) design is another critical component. The design process involves generating specific sequences that direct the CRISPR system to the intended genetic locations. Good gRNA design can significantly enhance the efficiency of gene editing.
Key considerations include:
- Target Sequence Specificity: The gRNA should ideally match perfectly with the target DNA sequence. Any mismatch can reduce the efficiency.
- PAM Sequence Availability: The presence of a protospacer adjacent motif (PAM) is crucial for Cas9 binding. Select sequences that are followed by a suitable PAM (e.g., NGG for SpCas9).
- Minimizing Off-Target Activities: Design gRNAs that minimize the potential for off-target effects. Computational tools can help evaluate and predict nucleases’ specificities.
Incorporating insights from various design tools, along with empirical validation, ensures more successful KO screens. A well-thought-out selection process of target genes and gRNA designs will form the backbone of a robust CRISPR KO screen.
Execution of CRISPR KO Screens
The execution of CRISPR knockout (KO) screens is a critical phase in the application of CRISPR technology for genetic studies. This part of the article focuses on the methodologies involved in performing these screens and how they contribute to achieving accurate and reproducible results. Understanding the nuances of transfection and the validation of knockouts is essential for researchers looking to leverage CRISPR technology effectively. It also helps delineate the advantages offered by this method compared to traditional techniques.
Transfection Protocols
Transfection protocols are fundamental for successfully delivering CRISPR components into target cells. The efficiency of transfection directly impacts the knockout's rate and the overall success of the experiment. Different methods exist, including chemical, viral, and physical approaches.
- Chemical methods typically utilize lipofection, where lipid carriers facilitate the uptake of CRISPR components. The process is relatively straightforward and suitable for various cell types.
- Viral methods, such as lentiviral vectors, are renowned for their high efficiency, especially in hard-to-transfect cells. They enable the stable incorporation of guide RNAs into the genome.
- Physical methods, like electroporation, apply electrical pulses to increase cell membrane permeability, allowing CRISPR components to enter.
Careful consideration must be given to cell line choice and the specific goals of the experiment to select the appropriate transfection method. Optimizing conditions, such as reagent concentrations and incubation times, can further enhance the transfection efficiency. Importantly, monitoring the expression of CRISPR components post-transfection helps verify successful delivery and functionality.
Selection and Validation of Knockouts
The process of selecting and validating knockouts is integral to confirming the effectiveness of a given CRISPR KO screen. After transfection, researchers must screen the cells to identify which had successful modifications.
First, selection markers can be used. These are genes inserted alongside the CRISPR components that confer survival advantages under specific conditions. By applying selective pressures, such as antibiotic treatment, only successfully modified cells survive, simplifying the identification process.
Following selection, validation is crucial. This step typically involves several techniques:
- PCR-based methods assess whether target genes have been successfully disrupted.
- Sanger sequencing confirms specific mutations caused by CRISPR.
- Functional assays can provide additional evidence. For instance, if the goal was to knock out a gene responsible for enzyme production, reduced enzyme activity would support the validation of the knockout.
Validation enhances confidence in the results and ensures that downstream applications, such as functional genomics studies, are based on accurate genetic modifications. Thus, thoughtful implementation of selection strategies and robust validation techniques are key to successful CRISPR KO screens.
Data Analysis in CRISPR KO Screens
Data analysis is a critical step in CRISPR knockout screening, serving as the bridge between experimental data collection and meaningful biological interpretation. Through this analysis, researchers can derive insights about gene function, identify essential pathways, and understand cellular responses to edits made by CRISPR. The significance lies not only in sorting through vast amounts of data but also in ensuring that the findings are both robust and reproducible. As genomic screens often produce complex datasets, the clarity of data analysis can ultimately determine the success of the study.
Quantitative Analysis Techniques
Quantitative analysis techniques play a pivotal role in evaluating CRISPR KO screening data, and these techniques are designed to convert raw data into quantifiable metrics that can drive interpretation. Common methods include:
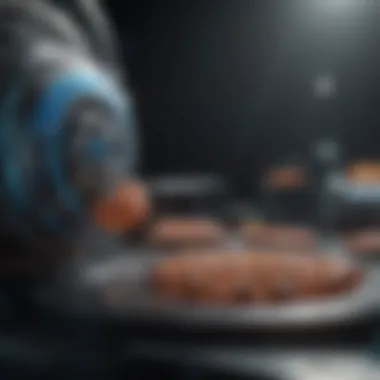
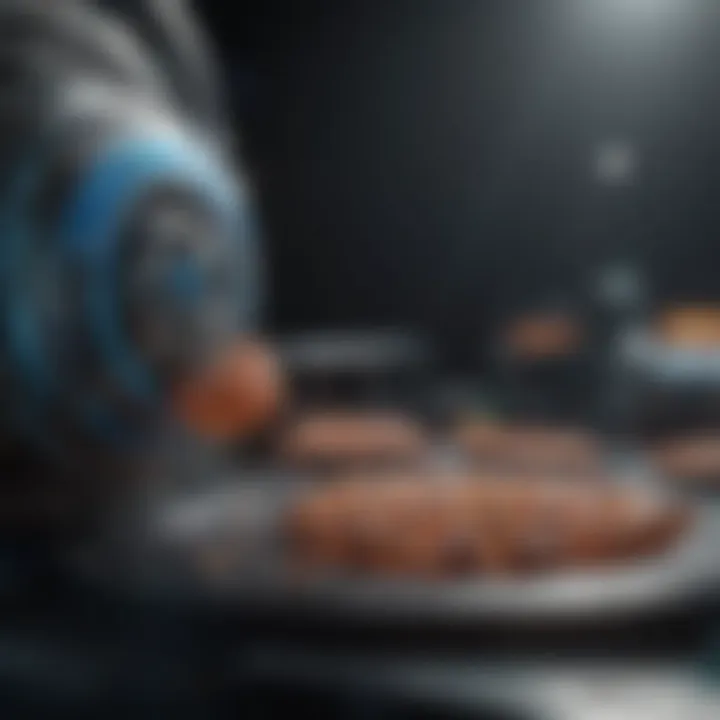
- Next-Generation Sequencing (NGS): This technique allows for high-throughput sequencing of the genomic regions targeted by CRISPR. By comparing knockout outcomes to control samples, researchers can determine the effectiveness of the guide RNAs.
- RNA Sequencing: This method can assess changes in gene expression following the knockout. By measuring the levels of mRNA, one can infer the functional impact of specific gene disruptions.
- qPCR (Quantitative Polymerase Chain Reaction): This technique offers an efficient way to validate the results obtained via NGS and RNA sequencing. It quantifies the specific mRNA levels post-knockout, thus confirming gene expression changes.
Each of these approaches provides quantitative data that not only support the detection of successful knockouts but also inform subsequent biological inquiries about the relevance of specific genes in various pathways.
Interpreting Screening Results
Interpreting screening results from CRISPR KO screens is vital to translating findings into biological insights. It involves a careful assessment of the quantitative data derived from previous techniques. Researchers typically focus on several factors such as:
- Statistical Robustness: Statistical methods are applied to determine whether the observed effects are significant. This often requires using tools like linear models or other statistical analyses to ensure the findings are not due to chance.
- Biological Context: Results must be analyzed within the broader biological context. It is essential to consider how the knockout affects cellular behavior in relation to existing knowledge about biological pathways and processes.
- Replicability: Results should be validated by replicating experiments. Replicability provides confidence that the observed phenotypes are indeed a result of the gene knockout.
To emphasize, the interpretation phase is paramount. A single false assumption during this stage can skew future investigations and lead to erroneous conclusions.
"Proper data analysis and interpretation are not mere technical requirements; they are foundational to the advancement of meaningful genomic research."
By focusing on thorough data analysis techniques and rigorous interpretation processes, researchers can turn high-volume data into concise, actionable insights that enhance our understanding of gene functions in various biological contexts.
Applications of CRISPR KO Screening
CRISPR knockout (KO) screening is a powerful tool with far-reaching implications in various domains. Its ability to disrupt specific genes allows researchers to uncover biological functions and understand complex pathways. The relevance of CRISPR KO screening extends into numerous fields, with significant contributions to functional genomics and drug development. By examining specific applications, this section illustrates how the CRISPR technique enhances our understanding of biology and medicine.
Functional Genomics Research
Functional genomics aims to understand the role of genes and their interactions within biological systems. CRISPR KO screening has fundamentally transformed this field by enabling targeted gene disruptions. With its high efficiency and precision, scientists can systematically study gene functions at a genome-wide scale.
In a typical functional genomics study using CRISPR, researchers introduce guide RNAs to create double-strand breaks in target genes. This disruption can lead to loss of function, allowing researchers to observe phenotypic changes. The resulting data provide insights into essential biological pathways, cellular responses, and disease mechanisms.
- Key Benefits of CRISPR KO in Functional Genomics:
- High throughput capability allows large-scale studies.
- Specificity in gene targeting minimizes off-target effects.
- Flexibility in modifying multiple genes simultaneously.
A significant example can be seen in cancer research, where CRISPR KO screens identify potential tumor suppressor genes or oncogenes. This information can lead to new therapeutic targets and better cancer treatment strategies. Moreover, researchers can explore gene function in developmental processes, metabolic pathways, and immune responses, pushing the boundaries of our current knowledge in genetics.
Target Identification in Drug Development
The path from discovery to effective therapeutics is complex and often arduous. CRISPR KO screening plays a substantial role in identifying and validating drug targets. By knocking out genes that contribute to disease, researchers can determine which molecular pathways are critical for disease progression.
In the context of drug development, the CRISPR KO approach allows scientists to prioritize targets based on their role in disease phenotypes. This strategy reduces the risk associated with pursuing ineffective targets, ultimately saving time and resources.
- Roles of CRISPR KO Screening in Drug Development:
- Identifying genetic vulnerabilities in disease models.
- Assessing the impact of potential drug candidates on gene expression.
- Evaluating resistance mechanisms related to therapies.
For example, in antiviral research, CRISPR KO screens have facilitated the identification of host factors that viruses depend on for entry or replication. Targeting these factors can lead to the development of innovative antiviral strategies. Additionally, by understanding resistance pathways in cancer treatments, researchers can design combinatorial therapies that enhance drug efficacy.
"CRISPR KO screening is not just advancing gene editing; it is revolutionizing drug discovery and functional genomics research, offering insight into the genetic basis of disease."
The implications of CRISPR KO screening for target identification in drug development are profound. Each application reinforces the importance of this technology in advancing our comprehension of biological systems and enhancing human health. The ongoing evolution of CRISPR techniques suggests further innovations will come, opening new avenues in functional genomics and therapeutic development.
Challenges in CRISPR KO Screening
CRISPR knockout screening offers powerful tools for gene editing and functional genomics. However, navigating the challenges that arise in these screens is crucial for successful outcomes. Recognizing and addressing these challenges helps in enhancing the accuracy of results and the integrity of the findings. This section explores the significant challenges faced when performing CRISPR KO screening, specifically focusing on off-target effects and biological variability.
Off-Target Effects
One of the most critical challenges is off-target effects. These unintended edits can occur when the CRISPR system binds to sites in the genome that are not the intended targets. As a result, off-target effects can lead to false interpretations or misleading data about genetic functions. The implications are significant, especially in therapeutic settings, where accuracy is paramount.
To minimize off-target activity, researchers may consider several approaches:
- Optimizing Guide RNA Design: Using advanced algorithms can improve the specificity of guide RNAs.
- Increased Use of High-Fidelity Nucleases: Utilization of engineered Cas9 variants that have enhanced specificity.
- Validation Through Deep Sequencing: Rigorous testing of potential off-targets using techniques such as next-generation sequencing.
It's essential for researchers to not only recognize the possibility of off-target effects but also to implement robust methods for their detection and minimization. This vigilance ensures that the results generated are reliable and reflective of true biological outcomes.
Biological Variability
Another considerable challenge in CRISPR KO screening is biological variability. This refers to the differences observed in cellular responses to CRISPR interventions among different biological systems or even within the same biological system over time. Variability can stem from several factors including genetic diversity, environmental influences, and the inherent characteristics of the cell types used in screens.
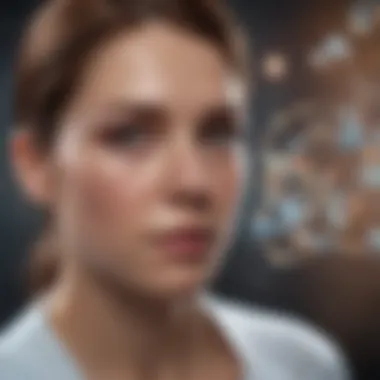
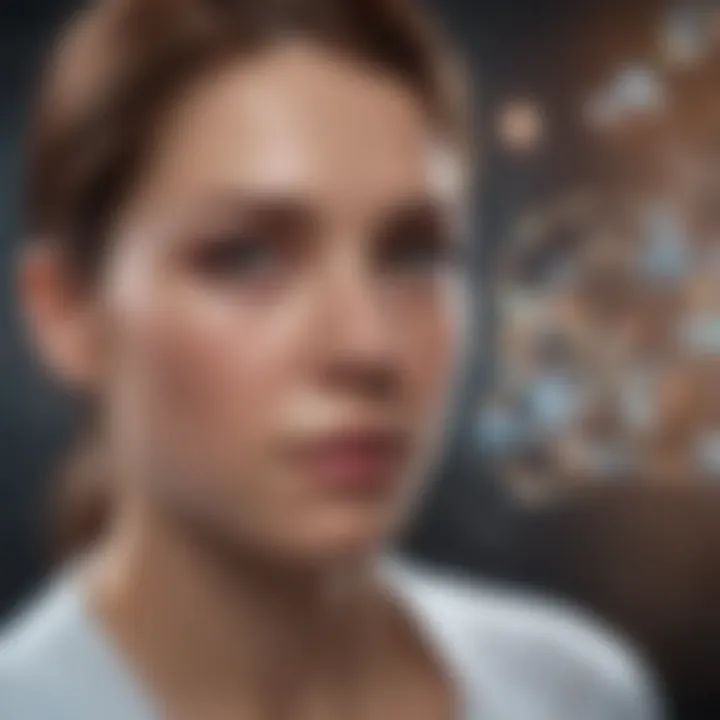
To address this, researchers can adopt the following strategies:
- Pilot Studies: Conducting preliminary experiments can help assess the degree of variability within a specific context.
- Standardizing Experimental Protocols: Consistency in protocols and methodologies can help reduce variability in results.
- Utilizing Biological Replicates: Including multiple biological samples in experimental design can yield more reliable data.
Focusing on biological variability is vital, as uncontrolled variation can distort study outcomes and complicate interpretations. Understanding these challenges aids researchers in creating more robust CRISPR KO screening studies that yield meaningful biological insights.
"The success of CRISPR KO screening significantly hinges on the ability to manage these challenges effectively, ensuring that findings are both reproducible and applicable in broader contexts."
In summary, addressing the challenges of off-target effects and biological variability is paramount in the quest to harness the full potential of CRISPR knockout screening. With ongoing advancements in technology and methodologies, researchers are better equipped to tackle these issues, contributing to the overall progress of genomic research.
Ethical Considerations in CRISPR Research
CRISPR technology, while revolutionary, is not free from ethical concerns. It is essential to explore these considerations because they influence the direction of research and public acceptance. Ethical discussions range from the implications of gene editing in humans to regulatory frameworks that govern these technologies.
Gene Editing and Regulation
Gene editing using CRISPR brings about significant advantages in medical and agricultural fields. However, it also raises key ethical questions regarding the scope and limitations of its application. Regulatory bodies are tasked with creating guidelines that ensure the responsible use of gene-editing technologies. This involves evaluating the long-term consequences of editing genetic material, especially in heritable forms.
Countries are approaching legislation differently. For example, in the United States, the regulatory landscape is less stringent compared to Europe, where regulations impose stricter limits on genetic modifications. There is a pressing need for a cohesive global approach to regulation. This would prevent individuals or organizations from exploiting gaps in regulation for unethical practices. Some practical steps that could be taken include:
- Establishing clear protocols for gene editing.
- Ensuring transparency in research.
- Involving ethicists in the discussions around gene editing projects.
By addressing these points, the scientific community can work towards a framework that balances innovation with ethical responsibility.
Public Perception and Acceptance
Public perception plays a critical role in the advancement of CRISPR technologies. Acceptance among the public can either foster or hinder scientific progress. People tend to have mixed feelings about gene editing, often influenced by media portrayals and lack of understanding.
The narrative around CRISPR is often shaped by sensationalist reports. These can amplify fears that overshadow potential benefits. Misconceptions about gene editing, especially in humans, can lead to opposition against scientific research. Education becomes crucial in this context. Providing clear, factual information can enhance public understanding and acceptance of CRISPR technology. Initiatives might include:
- Public forums to discuss gene editing technologies.
- Educational workshops targeting various age groups.
- Collaborative efforts between scientists and communicators to simplify complex ideas.
Engaging with the community and addressing their concerns openly can foster a more favorable attitude towards genetic research. Ultimately, the success of CRISPR technology is tied to its societal acceptance, making ethical considerations an ever-relevant aspect of genomic research.
Future Directions of CRISPR KO Screening
The field of CRISPR knockout (KO) screening is rapidly evolving, reflecting significant advancements in technology and methodology. Understanding the future directions of CRISPR KO screening can provide insights into its potential impacts on genomic research and related fields. As researchers continue to explore and refine this technique, several key elements emerge that highlight the promise and challenges of upcoming innovations.
Advancements in Technology
Recent advancements in CRISPR technology have taken the form of improved systems for gene editing, leading to more efficient and precise knockout screens. One notable development is the emergence of the CRISPR/Cas9 system, which has seen modifications aimed at increasing its specificity and reducing off-target effects. Innovations such as CRISPR/Cas12 and CRISPR/Cas13 offer enhanced precision for targeting genes, which may result in higher success rates for knockout screens.
Furthermore, new delivery mechanisms are being developed to facilitate the introduction of CRISPR components into various cell types. These include the use of viral vectors, nanoparticles, and electroporation techniques. Each method presents unique advantages and challenges, as they differ in efficiency, safety, and the ability to target specific cell types. In addition, multi-omics approaches that integrate genomic, transcriptomic, and proteomic data are becoming more commonplace. This integration enriches the analysis, providing a more comprehensive picture of how CRISPR-induced changes affect cellular functions.
In summary, advancements in technology not only enhance the effectiveness of CRISPR KO screenings but also broaden their applications across various biological systems. This evolution marks a shift towards more sophisticated research capabilities in the realm of genomics.
Potential for Multi-Target Screening
The potential for multi-target screening in CRISPR KO studies is another exciting avenue for future exploration. Traditional knockout screens often focus on single genes. However, the ability to knock out multiple genes simultaneously opens new possibilities for understanding complex biological networks and pathways. This approach mimics the interplay of genes more accurately, shedding light on functional redundancy and epistatic interactions within cellular systems.
Multi-target knockout screening can facilitate the identification of genetic interactions that are crucial for pathways involved in disease. Researchers can design experiments targeting various combinations of genes to assess their collective impact on cellular functions. This could be particularly useful in cancer research, where multiple genetic alterations often occur. By studying these interactions, scientists may uncover novel therapeutic targets or combinations, paving the way for more effective treatments.
Implementation of this multi-target approach, however, poses technical challenges. Designing guide RNAs that are effective across multiple targets without inducing off-target effects requires substantial computational and empirical strategies. As tools improve and knowledge deepens, the feasibility and efficiency of multi-target knockout screens should increase.
The strategic application of multi-target screening can push the boundaries of our current genomic research capabilities, significantly enhancing the understanding of genetic contributions to various diseases and traits.
The future landscape of CRISPR KO screening promises an exciting blend of technological advancements and multi-target methodologies, setting the stage for groundbreaking discoveries in genomics.
The End
The examination of CRISPR knockout (KO) screening in this article highlights its vital role in advancing genomic research. CRISPR technology itself has been transformative, enabling precise gene editing, which is critical for functional genomics. The significance of CRISPR KO screens in various research settings cannot be overstated. They facilitate the identification and characterization of gene functions, thereby enhancing our understanding of complex biological processes.
Summary of Key Points
- CRISPR Mechanism: The CRISPR system relies on specific guide RNAs to induce double-strand breaks in target DNA. This mechanism is central to the knockout process.
- Knockout Screen Design: Effectively designing a CRISPR KO screen involves careful selection of target genes and appropriate guide RNA constructs to maximize knockout efficiency.
- Data Analysis: Robust methodologies for data analysis are necessary to interpret the results of knockout screens, enabling researchers to draw meaningful conclusions.
- Applications: These screens have been used extensively in functional genomics, drug development, and even in the identification of therapeutic targets for diseases.
- Challenges: Researchers must navigate potential off-target effects and biological variabilities that may confound results.
- Ethical Considerations: As the power of CRISPR technology grows, so too must our deliberation over its ethical implications, especially regarding gene editing.
Implications for Future Research
The trajectory of CRISPR KO screening holds promise for further advancements in genomics and biotechnology. The continual refinement of CRISPR methods and tools is essential. Future research may likely focus on improving specificity to minimize off-target effects. Moreover, as technology improves, the potential for multi-target screening will expand, enabling comprehensive investigations into gene networks and their functions.
These developments not only enhance our understanding of genetic interactions but also open avenues for groundbreaking treatments in genomics-related fields. The future of CRISPR KO screening stands at a crossroads of technological innovation and ethical responsibility, requiring researchers to balance scientific exploration with societal implications.