Examining the Cas9 Protein: Structure, Function, and Impact
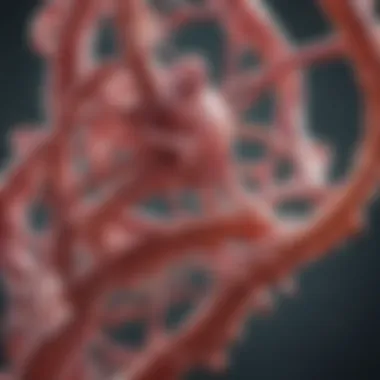
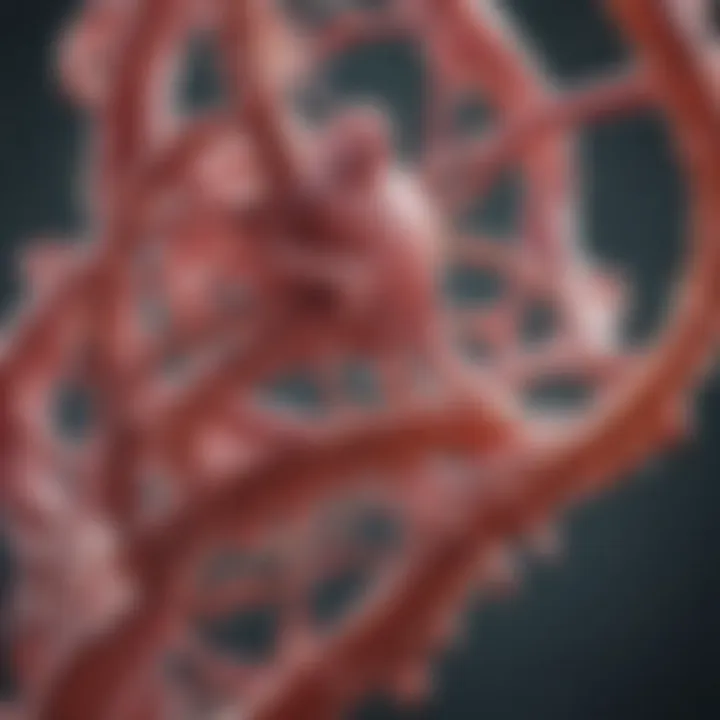
Intro
The Cas9 protein is a cornerstone of contemporary genetic engineering, notably within the CRISPR-Cas9 system. Understanding its properties is essential for anyone delving into the world of molecular biology and therapeutics. With its molecular weight often measured in kilodaltons (kDa), the Cas9 protein serves as a practical lens through which we can explore not just its functionality, but the broader implications for genetic manipulation.
This article sets out to dissect the Cas9 protein, presenting a thorough examination of its structure, significance, and diverse applications. With the rapid advancement of gene editing technologies, grasping the nuances of Cas9 becomes crucial for researchers, educators, and anyone keenly interested in the frontiers of genetic science.
Overview of Research Topic
Brief Background and Context
The discovery of the Cas9 protein was a remarkable twist in the tale of genetic engineering. Cas9 is derived from the bacterial immune system and serves as a tool to precisely edit genes in various organisms. Essentially, it operates by recognizing specific DNA sequences and making targeted cuts. The molecular weight of Cas9, around 160 kDa, plays a role in its biochemical interactions, stability, and functionality, making it a subject of keen study.
Historically, gene editing faced limitations due to the lack of precise tools, but the advent of CRISPR technology has revolutionized this field. By understanding the Cas9 protein more deeply, we can better appreciate how it contributes not just to research but also to potential therapeutic applications.
Importance in Current Scientific Landscape
In today’s scientific arena, the relevance of the Cas9 protein cannot be overstated. It has opened doors for advancements in medicine, agriculture, and biotechnology. For instance, researchers have utilized Cas9 in efforts to combat genetic disorders like sickle cell anemia and muscular dystrophy. The ability to make precision edits to DNA holds promise for curing diseases that were once deemed incurable.
Moreover, its implications for agriculture, such as improving crop resilience and nutritional content, highlight the broader impact of this protein on societal health and sustainability. Therefore, understanding the intricate details of Cas9 is paramount for scientists and industry professionals alike.
The CRISPR-Cas9 system could redefine how we approach not only disease treatment but also our understanding of genetics itself.
Methodology
Research Design and Approach
To yield a comprehensive understanding of the Cas9 protein, various research methodologies can be employed. A mix of structural analysis, computational biology, and laboratory experiments can elucidate how Cas9 interacts with DNA at a molecular level. By combining in vitro and in vivo studies, researchers can develop a more holistic view of Cas9's mechanisms and functions.
Data Collection Techniques
Data on Cas9 can be accumulated through several techniques, including:
- X-ray Crystallography: Used to determine the 3D structure of Cas9, providing insight into its functional mechanisms.
- Next-Generation Sequencing: This sheds light on how effectively Cas9 can edit genes in various contexts, highlighting its potential and limitations.
- Bioinformatics Tools: Leveraging these allows for the analysis of large datasets related to Cas9’s efficacy and efficiency across different organisms.
The integration of these techniques enables researchers to grasp the complexities involved with Cas9 and helps in overcoming the challenges faced in its application for therapeutic purposes.
Prelims to Cas9 Protein
The Cas9 protein has emerged as a linchpin in the field of genetic engineering, particularly due to its pivotal role in the CRISPR-Cas system. Understanding this protein is essential not only for its scientific applications but also for its broader implications in areas like medicine, agriculture, and biotechnology. By comprehensively examining the Cas9 protein, we can appreciate its structure, functional relevance, and the applications arising from its unique characteristics.
Overview of CRISPR-Cas Systems
CRISPR-Cas systems are a remarkable natural defense mechanism found in many bacteria. The system utilizes an array of genetic elements, enabling bacteria to recognize and eliminate foreign genetic material, such as that from viruses.
- Key Components: At the heart of these systems are two main components: CRISPR RNA (crRNA) and the Cas proteins, such as Cas9. The crRNA serves as a guide, ensuring that Cas9 accurately targets specific DNA sequences.
- Mechanism: When a bacterium encounters a virus, it integrates a segment of the viral DNA into its own genome, forming a part of the CRISPR array. Upon subsequent encounters with the same virus, the bacterium expresses the corresponding crRNA, leading Cas9 to the matching DNA sequence for cleavage. This mechanism effectively inhibits viral replication and protects the bacterial host.
The versatility of the CRISPR-Cas system has sparked intense interest among researchers. It opens new avenues for precise genetic editing by harnessing this natural immune response. The adaptability and effectiveness of Cas9 make it a focal point for various biotechnological innovations.
Significance of Cas9 in Genome Editing
The significance of Cas9 in genome editing cannot be overstated. This protein has revolutionized genetics, allowing scientists to make precise edits to DNA with unprecedented ease and accuracy.
- Precision: Unlike traditional gene-editing strategies, which can result in numerous off-target effects, Cas9 enables precise cuts at specific locations in the genome. This characteristic is essential for applications where even a minor error can lead to significant consequences.
- Applications: Cas9's ability to create targeted modifications has broad implications in fields such as:
- Medicine: From gene therapy to treating genetic disorders, Cas9 can potentially rectify mutations causing diseases.
- Agriculture: Breeders are utilizing Cas9 to introduce desirable traits into crops, enhancing yield, resistance to pests and diseases, and nutritional value.
- Research: The ability to knock out genes or introduce specific mutations aids in functional genomics, helping elucidate gene functions and interactions.
In summary, the Cas9 protein represents a monumental shift in genetic research and applications. As we delve deeper into its physical characteristics and functional mechanics, it becomes increasingly clear how crucial this protein is to shaping the future of genetic technology.
"The potential of Cas9 in shaping genetic engineering is equivalent to discovering electricity for the first time—transformative and full of possibilities."
Understanding the nuances of Cas9 is essential as we navigate the challenges and opportunities associated with its use in various sectors.
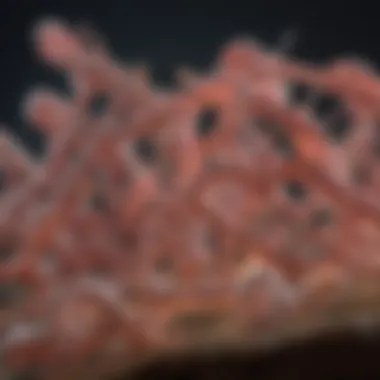
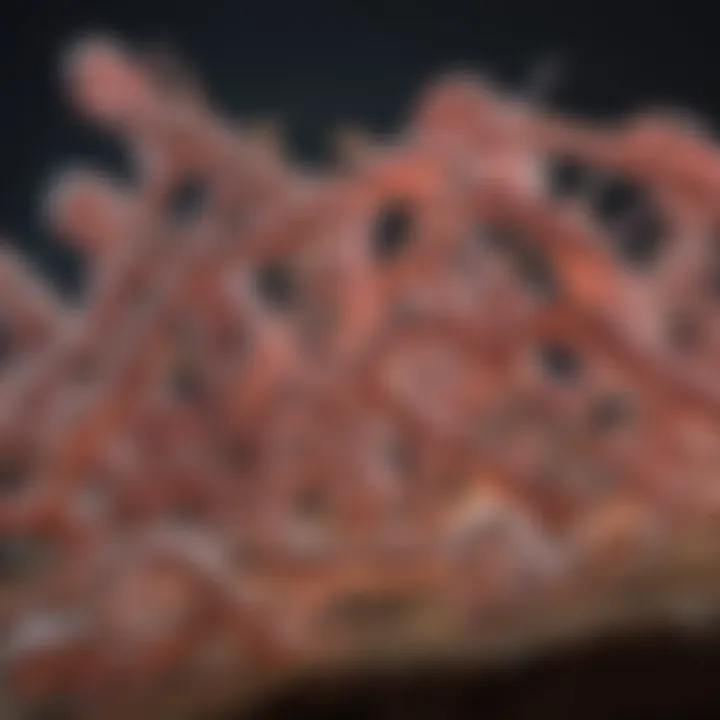
Physical Characteristics of Cas9 Protein
Understanding the physical characteristics of the Cas9 protein is vital. It not only helps in grasping the protein's potential functions but also sheds light on its mechanisms in gene editing. Cas9's size, shape, and structural domains dictate how it interacts with DNA, making this segment fundamentally important for researchers and professionals in the biology and biotechnology fields.
Molecular Weight and Its Measurement
Molecular weight is often expressed in kilodaltons (kDa), which is a standard unit for representing biomolecular sizes. Cas9 typically weighs around 160 kDa. This measurement is significant because it influences how the protein is designed and utilized in various applications. It guides decisions regarding the delivery methods, as heavier proteins may require more sophisticated strategies for cellular uptake.
There are several techniques utilized to measure the molecular weight of Cas9, such as:
- SDS-PAGE (Sodium Dodecyl Sulfate Polyacrylamide Gel Electrophoresis): This method separates proteins based on their size, allowing scientists to estimate the molecular weight of Cas9 by comparing its position on the gel against a standard ladder.
- Mass Spectrometry: This precise technique can provide detailed information about the molecular composition of Cas9, enabling a more accurate molecular weight determination.
Knowing the exact molecular weight allows researchers to better understand the protein's dynamics in vivo, such as its interactions with other biomolecules and its stability under various conditions.
Three-dimensional Structure
The three-dimensional (3D) structure of Cas9 reveals its functional capabilities. At its core, this structure allows for the effective binding and cleavage of target DNA sequences. Cas9 resembles a lopsided 'L,' with the two distinct lobes forming a cleft that accommodates the guide RNA and target DNA.
Using techniques like X-ray crystallography and cryo-electron microscopy, scientists have visualized the Cas9 structure in great detail. Here are some notable features:
- Recognition Lobe: The recognition lobe contains the specific regions that interact with the guide RNA, ensuring that Cas9 can accurately locate the target sequence.
- Nuclease Domain: This is where the actual cutting happens. The two important nuclease domains, RuvC and HNH, have specific roles in cleaving the strands of DNA.
A well-defined 3D structure not only clarifies its function in the CRISPR mechanism but also aids in engineering efforts, allowing the design of tailored variants optimized for specific applications.
Key Domains of Cas9
Diving deeper into Cas9, understanding its key domains adds to comprehending its functionality in genome editing. Here's a closer look at its primary domains:
- Target Recognition Domain (TRD): This part is crucial for determining specificity. Here is where Cas9 binds to the guide RNA, which in turn identifies the exact DNA sequence to be edited.
- Nuclease Domains:
- RuvC Domain: Particularly important for generating blunt ends, the RuvC nuclease cuts one strand of the DNA.
- HNH Domain: This domain is pivotal in cleaving the other strand, ensuring that Cas9 acts swiftly and accurately.
The unique architecture of these domains enables them to function cohesively, making nucleic acid manipulation both effective and efficient. As researchers pursue further innovations, the domains of Cas9 will likely continue to evolve, offering new opportunities in fields such as synthetic biology and gene therapy.
In summary, the physical characteristics of the Cas9 protein—from its molecular weight to its intricate 3D structure and critical domains—play a fundamentally important role in its function as a genome-editing tool. These details matter greatly in understanding how to harness Cas9's potential effectively.
Function of Cas9 Protein
The Cas9 protein serves as a critical player in the realm of genome editing, a cutting-edge scientific field that has immense implications for agriculture, medicine, and biotechnology. Understanding the function of Cas9 enriches our knowledge about how genetic modifications occur at a molecular level. Through this lens, several aspects shine through, including the mechanics of DNA cleavage, the role of RNA in guiding this process, and its evolutionary significance in bacterial defense.
Mechanism of DNA Cleavage
At its core, the action of the Cas9 protein is centered around its ability to cleave DNA strands—essentially, it acts like a pair of molecular scissors. This mechanism involves several key steps. When Cas9 is introduced into a cell, it binds to its accompanying guide RNA (gRNA), which has been designed to complement a specific DNA sequence in the target genome.
Once this complex is formed, the Cas9 enzyme scans the genome to locate the precise matching sequence. Upon finding it, the protein undergoes a conformational change to activate its endonuclease function. It then introduces a double-strand break in the DNA, which triggers the cell's repair mechanisms. This break is crucial for research and therapeutic applications, as it allows scientists to insert or delete genes at will.
"The double-strand break induced by Cas9 not only opens doors for gene editing but also provides a canvas for precision in genetic engineering."
Role in RNA-guided Endonuclease Activity
The ability of Cas9 to execute precise genetic alterations hinges on its RNA-guided endonuclease activity. The gRNA serves not just as a marker but as a navigation tool, ensuring that Cas9 reaches its intended target. The interaction between the gRNA and the target DNA is a kind of molecular recognize-and-seek game, where the gRNA must perfectly base-pair with the complementary DNA sequence. The accuracy of this pairing is paramount, as any mismatch can lead to off-target effects.
Furthermore, with the advent of advancements in gRNA design, researchers can now create guide sequences that boost the specificity of Cas9, optimizing its performance for targeted disruptions. The RNA component makes the Cas9 mechanism incredibly versatile, allowing swift modifications based on the user’s needs in diverse biological contexts.
Contribution to Adaptive Immunity in Bacteria
Interestingly, the origins of Cas9’s functionalities arise from the immune systems of bacteria. In nature, Cas9 is part of the CRISPR-Cas system, which provides adaptive immunity against bacteriophages. When a bacterium is attacked by a virus, fragments of the viral DNA are incorporated into its own genome, serving as a memory of the invader.
Later, if the same virus attacks again, the bacteria utilize this memory to create a specific gRNA targeting the viral DNA. Cas9 then employs its cutting function to disable the invader’s genome, safeguarding the bacterial cell. This evolutionary mechanism showcases not only why Cas9 is so effective but also its significance beyond laboratory settings, highlighting the inherent ingenuity of nature in crafting tools for survival.
Applications of Cas9 Protein in Research
The Cas9 protein is not just a tool in the genetic toolkit; it's a cornerstone in contemporary research strategies aimed at genetic manipulation. Its versatile applications span across various fields, making it crucial for scientists looking to tweak the genome. The significance of Cas9 lies in its ability to cut DNA precisely, which opens doors to numerous research possibilities. The future of medicine, agriculture, and our understanding of biological systems hinges on the effective application of this protein. Here are some key domains where Cas9 is making its mark:
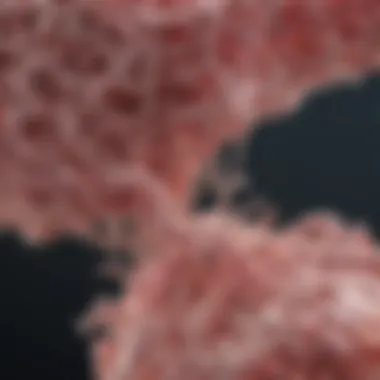
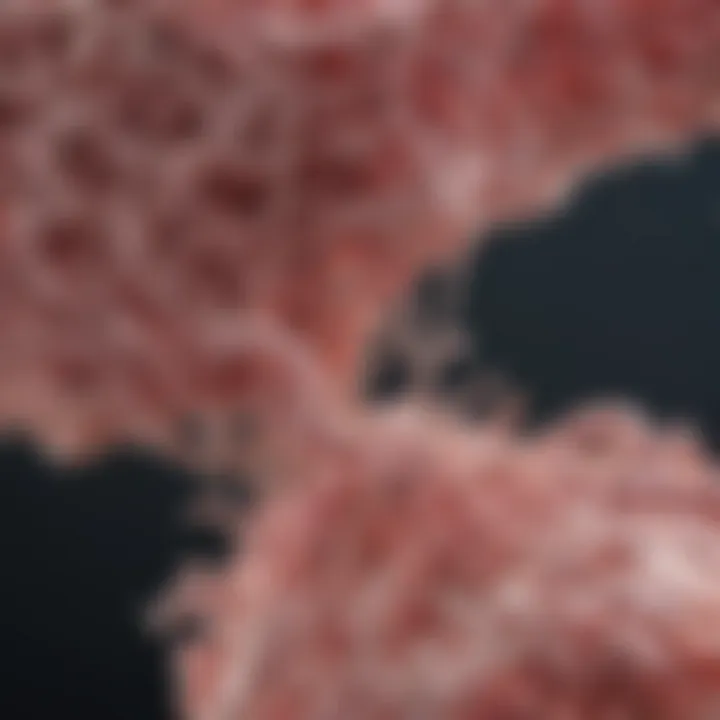
Gene Editing in Model Organisms
Understanding genetics often begins with model organisms like Drosophila melanogaster (fruit flies), Caenorhabditis elegans (roundworms), and Mus musculus (mice). These organisms have served as excellent proxies for human diseases. The application of Cas9 in these model organisms has revolutionized the field of developmental biology and disease research.
- Precise Modifications: By using the CRISPR-Cas9 system, researchers can introduce or eliminate specific genes, allowing them to study gene function meticulously. For example, altering the PTEN gene in mice has provided insights into tumorigenesis.
- Predictive Models for Human Conditions: By modifying genes that are also implicated in human diseases, scientists create models that help in studying the progression of ailments like Alzheimer's and cancer, ultimately aiding drug discovery.
- Rapid Generational Studies: With the speed of CRISPR technology, researchers can observe phenotypic changes across generations, providing a richer dataset for analysis when compared to older methods that were time-consuming and less efficient.
Human Therapeutics and Disease Models
The journey of translating lab findings into real-world therapies often involves tackling human diseases. Cas9's role here is paramount.
- Gene Therapy: Utilizing Cas9 to precisely edit genes in somatic cells holds the potential to treat genetic disorders. For instance, correcting the β-globin gene can alleviate conditions like sickle cell anemia.
- Base Editing: Advances in Cas9 technology led to the development of base editors, which can convert one DNA base into another without causing double-strand breaks. This enhances the precision of genomic edits, crucial for therapeutic applications.
- Disease Models: By pairing Cas9 with patient-derived cell lines, researchers create models that better mimic the genetic landscape of human diseases, improving the efficacy of potential drugs by tailoring treatments based on specific mutations.
Agricultural Biotechnology Applications
As the world's population grows, agriculture faces the challenge of feeding millions. The application of Cas9 in agriculture promises enhanced yield and resilience in crops.
- Crop Improvement: The ability to edit genes that control traits like drought resistance or pest susceptibility means that crops can be optimized for performance. For example, modifying the OsSWEET12 gene in rice has shown improved resilience against bacterial blight.
- Disease Resistance: By targeting pathogens at the genomic level, Cas9 allows for the development of crops that can fend off diseases, which is crucial in maintaining food security.
- Sustainability: Engineering crops to use resources like water and fertilizer more efficiently is another area where Cas9 can play a pivotal role, allowing for environmentally sustainable agricultural practices.
"The advancement of CRISPR technology, particularly the application of Cas9, could reshape the landscape of food production and population health alike."
These applications share a common thread: the transformative impact of Cas9-related innovations. The possibilities are immense, not just for current research applications but also for future endeavors across health and agriculture that could benefit society at large.
Challenges and Limitations of Using Cas9
The Cas9 protein, while revolutionary in the realm of gene editing, brings with it a handful of challenges and limitations that researchers must navigate. Fully understanding these hurdles is crucial for enhancing the efficacy of gene-editing technologies and ensuring their safe application in a variety of contexts. In this segment, we will dive into specific issues, including off-target effects, delivery mechanisms, and the immunogenicity of Cas9, each of which presents unique considerations that demand careful attention.
Off-target Effects in Genome Editing
One major concern with Cas9 technology is the occurrence of off-target effects. This phenomenon occurs when Cas9 mistakenly edits the wrong part of the genome due to sequence similarity. Despite the high precision that CRISPR-Cas9 is touted for, off-target activity can lead to unintended genetic modifications, raising both safety concerns and ethical implications.
- Consequences of Off-target Effects: Unwanted edits can disrupt essential genes or regulatory regions. This might potentially create health issues or impact the organism's overall function. For example, in therapeutic settings, such unintended modifications could lead to new diseases rather than curing existing ones.
- Measurement of Off-target Effects: Researchers employ various techniques to identify off-targets, including whole-genome sequencing and targeted deep sequencing, enabling them to assess the scope and impact of unintentional edits. However, these methods often come with high costs and time investments.
It's evident that while Cas9 holds immense potential for precise gene editing, prioritizing accuracy and minimizing off-target effects are vital for its responsible application in both research and clinical settings.
Delivery Mechanisms for Cas9
Another aspect that complicates the use of Cas9 is its delivery into target cells. Efficient delivery is paramount for ensuring that the desired edits occur in the right location. Several methods exist, though each comes with its own set of advantages and disadvantages.
- Viral Vectors: These are often used due to their high efficiency in delivering Cas9 and guide RNA into cells. However, concerns about immunogenicity and the potential for insertional mutagenesis persist.
- Lipid Nanoparticles: These offer a non-viral alternative that is relatively easier to produce and can encapsulate nucleic acids effectively. Yet, they might not achieve the same level of delivery efficiency as viral systems.
- Physical Methods: Techniques such as electroporation and microinjection are also employed, particularly in research settings. While they can be quite effective, they may cause cellular stress or damage, particularly in sensitive cell types.
Finding the ideal delivery mechanism hinges on balancing efficiency and safety. As the field evolves, novel delivery systems are under investigation, seeking to improve the precision and efficacy of Cas9 delivery to target cells.
Immunogenicity of Cas9 in Therapeutics
The immunogenic nature of Cas9 poses a significant barrier, especially in therapeutic applications. Since Cas9 originates from bacterial species, the human immune system may recognize it as foreign, leading to immune responses that could hinder its effectiveness in gene editing.
- Potential Reactions: While some individuals may exhibit minimal reactions, others could experience heightened immune responses. This variability complicates the predictability and safety of Cas9-related therapies.
- Strategies to Mitigate Immunogenicity: Several strategies have been explored, including using Cas9 variants or engineering modifications that reduce its immunogenic profile.
"The path to precision gene editing is paved with challenges and complexities that demand ingenuity and persistence."
Navigating these obstacles is essential not only to harness the potential of Cas9 but to do it responsibly and effectively.
Advancements in Biotechnological Research on Cas9
The examination of advancements in biotechnological research on Cas9 protein is not just a passing interest; it serves as the bedrock of ongoing innovations in genetic engineering. As scientists continually explore ways to harness the capabilities of the Cas9 protein, we witness a shift that not only enhances the efficiency of gene editing but also ensures precision in various applications. The evolution of this research delivers insights into how modifications to Cas9 can lead to breakthroughs in medical therapies, agricultural improvements, and fundamental biological research.
Engineering High-efficiency Variants
In recent years, there has been a tangible focus on engineering variants of Cas9 that exhibit higher efficiency in gene editing. The traditional Cas9 protein from Streptococcus pyogenes has proven invaluable; however, its limitations in certain contexts—like specificity and efficiency—prompted researchers to optimize it.
Scientists have introduced engineered variants, such as SpCas9-HF1, which is designed to minimize off-target effects while maintaining robust targeting capabilities. The advancements in molecular biology techniques facilitate straightforward modifications to the Cas9 protein, making it possible to tailor its activity to meet specific needs.
- Benefits of High-efficiency Variants:
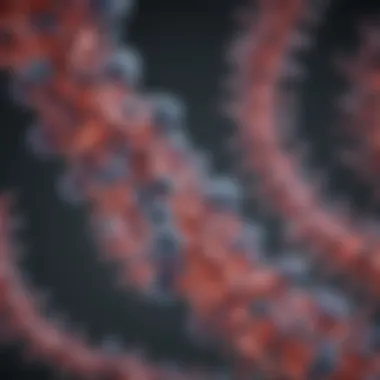
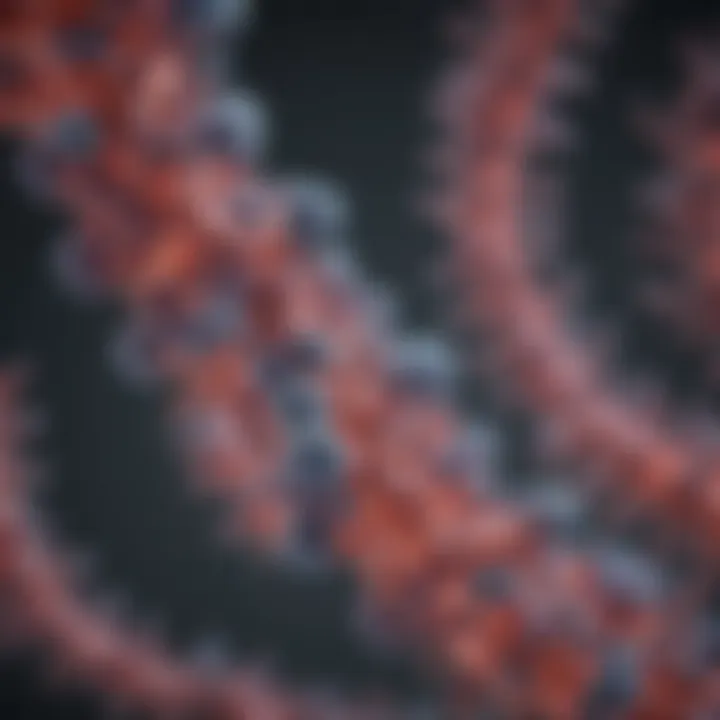
- Reduced Off-Target Effects: Target mutations are less likely to occur in unintended areas of the genome.
- Increased Targeting Range: More effective in targeting difficult regions of the genome, which traditional Cas9 might overlook.
- Enhanced Compatibility: Better integration with various delivery systems, extending the technology's applicability in therapeutics.
"The innovation doesn't stop at creating variations of Cas9; it's about redefining the boundaries of genetic engineering itself."
Improved Specificity Through Mutations
In the relentless quest for precision, the idea of introducing specific mutations to enhance the selectivity of the Cas9 protein has garnered significant interest. Refinements in the protein's sequence can lead to dramatic alterations in how it interacts with the DNA.
For instance, mutations in the CRISPR-associated cleavage domains can result in a selective suite of Cas9 proteins geared towards specific genomic locations. Researchers have employed an approach known as RNP (ribonucleoprotein) delivery directly into cells, which not only bolsters specificity but also mitigates potential immunogenic responses.
- Key Considerations:
- Tailoring for Different Organisms: Species-specific designs help in effective genome editing of diverse life forms.
- Preventing Unpredictable Outcomes: Better specificity reduces the risks associated with unintended genetic changes.
- Expanding the Toolkit: Development of mutant Cas9 proteins broadens the scope of CRISPR applications in various fields.
Alternative Cas Proteins in Genome Editing
While Cas9 from S. pyogenes has become synonymous with CRISPR, there lies a multitude of alternative Cas proteins that are emerging as powerful contenders in the landscape of genome editing protocols. These alternative proteins are especially useful for addressing limitations presented by Cas9, such as size and specific DNA target preferences.
For instance, Cas12 and Cas13 proteins offer unique mechanisms for interaction with DNA and RNA, respectively. Their structural characteristics facilitate a wide range of applications, from diagnostic methods to targeted genetic modifications in a more precise manner. Similarly, CasX and CasY proteins are being explored for their compact size, which enhances the feasibility of delivery into certain cells.
- Key Advantages of Alternative Cas Proteins:
- Broader Target Range: Many alternative Cas proteins can recognize distinct PAM (protospacer adjacent motif) sequences, making them applicable to a wider array of genomic loci.
- Distinct Modes of Action: Cas12 has a unique ability to create staggered DNA ends, useful for certain types of modifications.
- Minimized Delivery Challenges: Smaller Cas proteins like CasX can easily fit into viral vectors for delivery into patients or experimental models.
Future Directions in Cas9 Research
The exploration of Cas9 protein is an ever-evolving field with numerous possibilities for advancing genomic science. Future directions in Cas9 research are pivotal for amplifying its capabilities and addressing its constraints. As scientists grow ever more curious about the inherent possibilities tied to this protein, new avenues of inquiry emerge, driving innovation and refinement in genomic technologies.
Innovative Delivery Systems
One of the fundamental hurdles facing the successful application of the Cas9 protein is getting it into the target cells effectively. Traditional methods involve using viral vectors, but these come with drawbacks, such as potential immunogenicity and safety concerns. Innovative delivery systems are emerging, and they promise to enhance the precision and efficacy of gene editing.
- Lipid Nanoparticles: This method encapsulates the Cas9 protein and guide RNA, making it easier for cells to absorb them.
- Electroporation: A technique that uses electrical pulses to increase cell permeability and facilitate the entry of Cas9 components.
- Microinjection: Directly introduces Cas9 into the cytoplasm of a cell, leading to efficient gene editing in embryos, particularly in model organisms.
These methods can significantly elevate the efficiency of gene editing, enabling researchers to tackle complex genetic challenges with more finesse. Developing user-friendly and cost-effective delivery systems remains a priority, ensuring a broader range of laboratories can harness these cutting-edge techniques.
Integration with Other Genomic Technologies
The fusion of Cas9 with other genomic technologies stands as another fertile ground for exploration. Technologies such as CRISPR-Cas12 and various RNA-based approaches are ripe for integration with the current CRISPR-Cas9 framework. Such combinations can enhance specificity and accommodate jobs that require subtler manipulations than what Cas9 typically offers.
- Base Editing: This next-gen technique enables the conversion of single nucleotide variants without introducing double-strand breaks. Combining base editing with Cas9 expands its versatility in making precise changes without the risk of larger mutations.
- Prime Editing: Billed as a "search and replace" tool for DNA, prime editing can introduce targeted changes with unprecedented accuracy, potentially minimizing off-target effects associated with traditional Cas9 editing.
Having a toolset that integrates various approaches allows for a comprehensive strategy in tackling genetic disorders, thereby enriching our understanding and manipulation of genes in diverse settings—from agriculture to therapeutics.
Ethical Considerations in Cas9 Applications
As we dive deeper into the technical advancements surrounding Cas9, a magnifying glass is placed on the ethical implications that arise. The power to edit genomes carries significant responsibility. Ethical considerations are multifaceted and must be attuned to the repercussions of deploying these technologies.
- Germline Editing: Modifying the genetic makeup of embryos raises questions about consent, ownership, and the risk of unforeseen consequences in lineages.
- Potential for Misuse: With great power comes the potential for abuse. Tools like Cas9 might be employed inappropriately, leading to bioethical dilemmas concerning designer organisms or unintended ecological impacts.
- Equity in Access: As biotechnological advancements progress quickly, the disparity in access to these tools can lead to broader societal inequality. Ensuring equitable access will be a challenge, particularly in regions with limited resources.
Addressing these ethical challenges requires a concerted effort from scientists, ethicists, and policymakers to sculpt a framework that governs the responsible use of Cas9 technology. The dialogue must remain open and proactive as we tread these transformative waters.
The End
The discussion around the Cas9 protein is not just a passing fad; it underlines an era in biomedicine and genetics that beckons significant evolution in research methodologies and therapeutic approaches. Understanding the structure, function, and applications of this pivotal protein illuminates pathways we can take towards innovative scientific endeavors. Its molecular weight measured in kilodaltons gives insights into the protein’s capabilities, while its highly adaptive structure allows for precision that was previously unattainable. These insights push the boundaries of gene editing as we know it.
Summary of Key Insights
Several crucial points arise throughout the exploration of Cas9:
- Structural Dynamics: The Cas9 protein is integral to the CRISPR-Cas9 system as a whole, acting as both a guide and a scalpel in genome editing tasks.
- Functional Versatility: From bacteria to humans, its diverse operational roles demonstrate its capacity to affect profound changes in various biological systems.
- Applications Across Fields: Whether in agricultural biotechnology or revolutionary human therapeutics, its applications show the potential to address some of the pressing issues of our time, such as food security and disease eradication.
- Challenges on the Horizon: While the benefits are significant, challenges such as off-target effects and delivery mechanisms must be reconciled before Cas9 can be universally relied on.
Final Thoughts on Cas9's Role in Science
Cas9 has positioned itself as a beacon for innovation within the field of genetic engineering. Its capability to precisely alter DNA has fostered a new wave of scientific inquiry and transformative technologies. However, with great power comes immense responsibility. Ethical considerations around gene editing, particularly concerning its applications in humans, must be at the forefront of discussions moving forward. This balance between innovation and ethics will ultimately shape the future trajectory of biotechnological advancements.
"We stand on the shoulders of giants; how we proceed from here will define our place in history."
The story of Cas9 is a testament to ambition in science and the relentless pursuit of knowledge. While it’s a complex narrative replete with ethical dilemmas and scientific challenges, the journey has only just begun. As researchers continue to unveil the capabilities of this remarkable protein, its role in science will inevitably grow and adapt, reflecting the very essence of discovery itself.