ATP Cofactors: Energy's Essential Partners
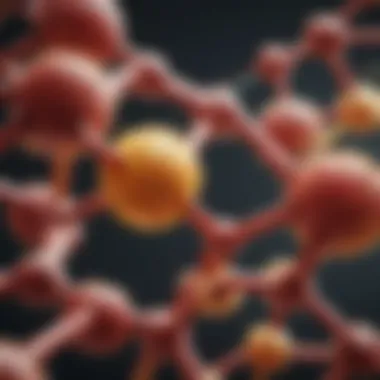
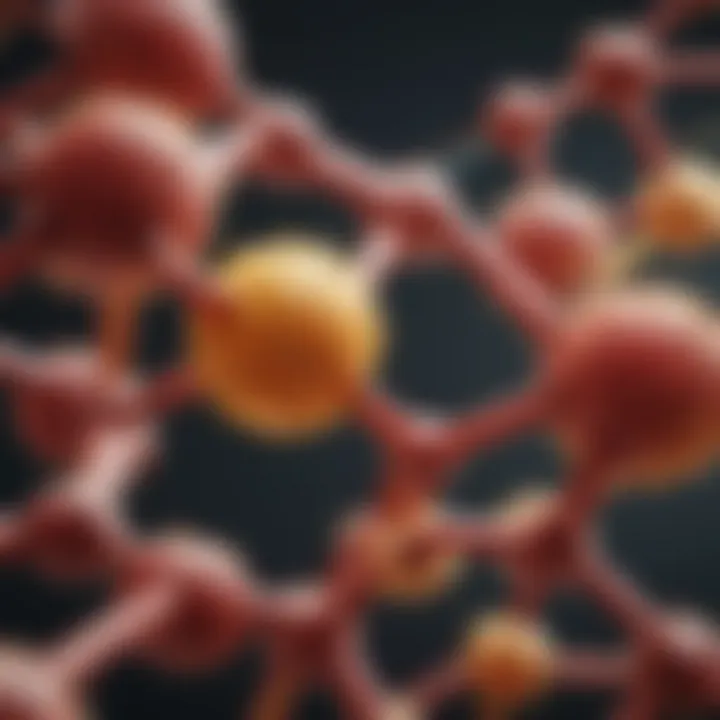
Intro
Adenosine triphosphate, or ATP, is often termed as the energy currency of biological systems. It’s what keeps the wheels of life turning. But ATP doesn't work alone. That’s where cofactors come into play, working behind the scenes to ensure that ATP can perform its crucial functions in the cell.
Before diving deeper, it's essential to set the stage and understand what cofactors are in the context of ATP. These auxiliary molecules can be metal ions or organic compounds. In many cases, they help enzymes catalyze biochemical reactions that are essential for life. Without these cofactors, processes such as metabolism and cellular energy transfer would falter, leaving cells unable to perform their necessary roles.
The presence of cofactors amplifies the efficiency and scope of ATP's capabilities, linking it to myriad cellular functions. Their role is not just supplementary; it is integral. Thus, our exploration of ATP cofactors is crucial for a thorough understanding of physiological processes, cellular respiration, and even the implications in health and disease.
Overview of Research Topic
Brief Background and Context
As we peel back the layers on ATP cofactors, it's worth noting that much of the research surrounding them has emerged in tandem with advances in biochemistry and molecular biology. Historically, ATP has been the rock star of energy metabolism—everyone talks about it, but the supporting cast has often been overlooked. This has started to change as scientists reveal more about not only how ATP functions but also the vital helpers that make its interactions possible.
Importance in Current Scientific Landscape
Understanding ATP cofactors is particularly relevant today. With the rise of interest in metabolic disorders, bioenergetics, and the role of micronutrients in health, the function and significance of cofactors are gradually coming into focus. For instance, when examining conditions like diabetes or mitochondrial diseases, researchers are realizing that deficiencies in certain cofactors may disrupt how effectively ATP is utilized, leading to significant metabolic consequences. The intricate dance between ATP and cofactors is becoming more than just a curiosity in a textbook; it’s swiftly climbing the agenda in contemporary biomedical research.
"The interplay between ATP and its cofactors is not just a biochemical curiosity; it's central to understanding metabolic health and disease."
Methodology
Research Design and Approach
In studying ATP cofactors, a multi-pronged research design is often adopted. This typically combines laboratory techniques and computational models to evaluate how various cofactors interact with ATP in both isolated systems and cellular environments. Understanding these interactions requires a synthesis of experimental and theoretical findings.
Data Collection Techniques
Data collection in this realm might involve techniques such as spectrophotometry, chromatography, and electrophoresis to identify and quantify the roles of specific cofactors in ATP-related reactions. Additionally, genetic and metabolic profiling techniques, including CRISPR gene-editing and mass spectrometry, help elucidate the relationships between cofactors and cellular energy dynamics.
The ongoing studies are pivotal for bridging the gap in our understanding of energy metabolism that has existed for far too long. Hitting the books, diving into the lab, or getting behind the screen—there’s no shortage of resources to help unravel this fascinating topic.
Preamble to ATP and Its Functions
Adenosine triphosphate, or ATP, is a molecule that plays a vital role in the functioning of all living cells. Understanding ATP and its functions provides insight not just into energy transfer but also into the very mechanism of life itself. ATP acts as a conveyor belt of energy, continually facilitating biochemical reactions that are essential for various cellular processes. In this article, we shall explore the indispensable nature of ATP in detail. By diving deep into its functions, we aim to clarify the broader implications of ATP, especially when considering the role cofactors play in enhancing its efficiency and significance in biological systems.
Defining ATP
To understand ATP’s role in biological systems, one must start with its definition. ATP is a nucleotide composed of three key components: a nitrogenous base (adenine), a ribose sugar, and three phosphate groups. The intricate structure is what allows ATP to store energy. The bonds between the phosphate groups are high-energy links, meaning when these bonds are broken—specifically when ATP is converted to adenosine diphosphate (ADP)—a significant amount of energy is released. This energy is harnessed by cells to perform a range of functions, from muscle contraction to active transport across membranes.
Role of ATP in Cellular Processes
ATP is often dubbed the "molecular unit of currency" for its role in powering numerous biological processes. But what does this mean in practical terms? Several functions illustrate ATP's significance:
- Energy expenditure: ATP powers almost every reaction that requires energy within the cell, ensuring metabolism proceeds effectively.
- Regulation of enzyme activity: Many enzymes require ATP to function, as it serves as a substrate that can activate or inhibit their activities, thereby controlling metabolic pathways.
- Communication: ATP plays a role in cell signaling. It's involved in transmitting messages throughout the cellular landscape, ensuring that responses to environmental changes are swift and precise.
"ATP is to the cell what cash is to an economy — it's the currency that enables growth, movement, and communication at the molecular level."
In summary, ATP is much more than a simple energy molecule; it is central to the kinetic tapestry of life. Understanding ATP — its composition, function, and mechanism — lays the groundwork for the critical exploration of ATP cofactors that enhance its abilities and deal with health implications. As we progress through this guide, illumination will be shed on how co-factors amplify ATP’s effectiveness, their sources, and their significance in overall physiology.
Cofactors: A Brief Overview
Cofactors play a pivotal role in biological systems, acting as essential accomplices to enzymes and a variety of biochemical reactions. Without these helpers, the myriad of processes that sustain life would encounter significant hurdles. Their involvement is not merely supplementary; rather, they are intricately involved in the fine-tuning and effectiveness of enzyme activity, which in turn influences metabolism and overall cellular function.
In the context of ATP, cofactors enhance the stability and availability of this vital molecule, enabling it to fulfill its role as the energy currency of the cell. They not only aid in the synthesis of ATP, but their influence extends throughout metabolic pathways, affecting how energy is utilized and replenished within biological systems.
Among the various types of cofactors, we can discern several classifications which provide insight into their diverse functions. Thus, a deep understanding of cofactors is not only beneficial, but essential for grasping the dynamics of cellular processes.
Classification of Cofactors
Cofactors can essentially be classified into two main categories: metal ions and organic cofactors.
- Metal Ions: These are positively charged ions that can interact with enzyme active sites. Common metal cofactors include:
- Organic Cofactors (Coenzymes): These tend to be larger molecules, often derived from vitamins. Key examples include:
- Zinc: Often found in enzymes like carbonic anhydrase and DNA polymerase, playing crucial roles in catalysis and structural integrity.
- Magnesium: Well-known for its role in stabilizing ATP, it is critical for a host of enzymatic reactions involving phosphate transfers.
- Iron: Integral to various blood proteins like hemoglobin; it participates in electron transport and redox reactions in the body.
- NADH: A nucleotide that plays a significant role in cellular respiration and energy production.
- FAD: Functions similarly to NADH, crucial for the mitochondrial electron transport chain.
- Coenzyme A: Vital for fatty acid metabolism and the citric acid cycle.
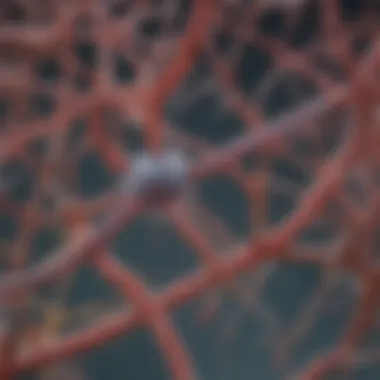
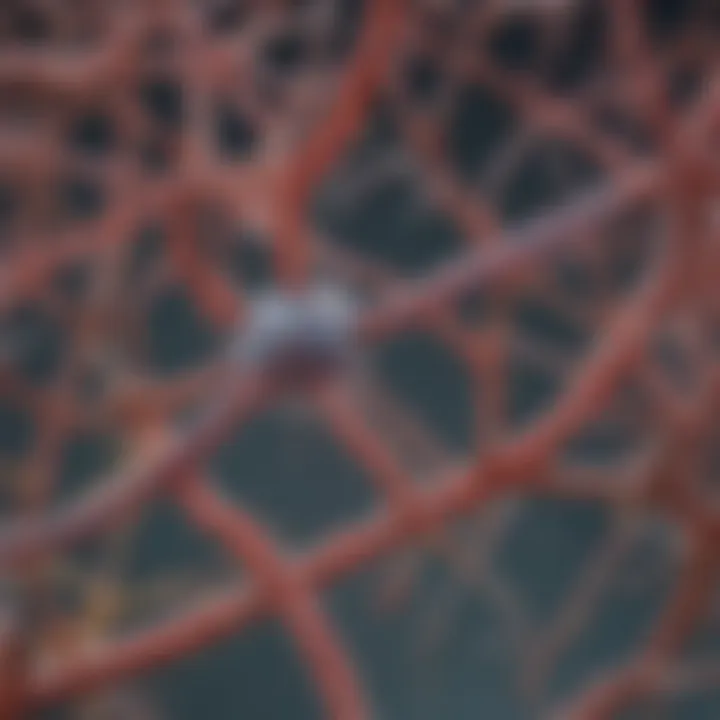
The classification is not merely a matter of taxonomical convenience but rather highlights the diverse functionalities that different cofactors bring to the biochemical landscape. As we can see, each type possesses unique characteristics that enable them to perform specific tasks—vital for maintaining the bustling life processes within cells.
Essential vs Non-Essential Cofactors
Cofactors can also be split into two categories based on their necessity for life: essential and non-essential cofactors.
- Essential Cofactors: These are substances that organisms cannot synthesize on their own and must obtain through their diet or other means. For instance, certain metal ions like magnesium and zinc are indispensable for various enzymatic reactions and must be periodically replenished.
- Non-Essential Cofactors: Unlike their essential counterparts, non-essential cofactors can be synthesized by the organism itself. However, they might still be advantageous to obtain from external sources. An example could be certain coenzymes that, while not strictly necessary, enhance metabolic efficacy under specific conditions.
In summary, understanding the classification and necessity of cofactors lifts the veil on how they influence ATP's functionality and, by extension, the intricate web of cellular processes. Their roles are multi-faceted and interlinked, underscoring that life is indeed a concert played by numerous musician-stylistic forces, each contributing to the harmony of function at a cellular level.
Types of ATP Cofactors
Understanding the types of ATP cofactors is essential as they play a pivotal role in facilitating biochemical reactions that are critical for maintaining life. These cofactors enhance the functionality of ATP by ensuring that energy transfer processes are efficient and effective. They can be classified broadly into metal ions and organic cofactors, each with unique characteristics that contribute to their functionality.
Metal Ions as Cofactors
Zinc
Zinc is often regarded as a trace element, but don’t let its small size fool you. It holds notable importance as a cofactor in numerous enzymatic reactions. One of the standout aspects of zinc is its ability to stabilize structures of proteins, which is key in enzymatic function. This metallic element is also critical in the catalytic activity of enzymes involved in DNA synthesis and repair.
Why Zinc? It's particularly attractive due to its relative abundance and versatility in various biological systems. Most importantly, zinc supports numerous cellular processes, from immune function to wound healing.
A unique characteristic of zinc is that it can exist in multiple oxidation states, which allows it flexibility in various biochemical reactions. However, it's worth noting that excessive zinc can lead to toxicity, making it a double-edged sword in biological systems.
Magnesium
Magnesium stands out as another key player among metal cofactors. This ion is vital for stabilizing ATP molecules, essentially acting as a gatekeeper for energy transfer. The primary feature of magnesium is its ability to form complexes with ATP, which is essential for enzymatic activities as it enhances the affinity of substrates to the enzymes involved in phosphorylation reactions.
Why is Magnesium Important? Magnesium has profound implications for overall health, including muscle functioning and nerve transmission, making it indispensable for many cellular activities. It’s often touted for its role in ATP hydrolysis, where it facilitates the release of energy, a crucial process in metabolism.
A special feature of magnesium is its energetic stabilization. While it is broadly beneficial, deficiencies can result in muscle cramps and neurological issues, which can be detrimental to cellular health.
Iron
Iron, often associated with blood health, finds its role as a cofactor in ATP-dependent processes as well. This metal is integral in various respiratory enzymes, which are critical in cellular energy metabolism. One of iron's defining characteristics is its ability to undergo oxidation and reduction, which is essential in energy transfer mechanisms.
Why Iron Matters? It is particularly viewed as a beneficial cofactor due to its involvement in the electron transport chain, which is fundamental for ATP synthesis in aerobic respiration.
An exceptional aspect of iron is its role in hemoglobin and myoglobin, facilitating oxygen transport in the bloodstream. However, too much iron can lead to oxidative stress and damage to cellular structures.
Organic Cofactors and Coenzymes
NADH
NADH operates as an essential electron carrier in metabolic pathways, especially glycolysis and the citric acid cycle. This cofactor is pivotal in the process of converting food into energy. One of its key features is that it can easily undergo reversible oxidation and reduction processes, allowing it to shuttle electrons effectively within the cell.
Importance of NADH: Its presence enables the continuous cycling of biochemical reactions that require electron donation, making it extremely beneficial in energy metabolism.
A unique aspect of NADH is its role in regenerating NAD+, which is crucial for sustaining metabolic pathways. Nonetheless, levels of NADH must be carefully regulated, as imbalances can lead to metabolic disorders.
FAD
FAD is another noteworthy organic cofactor, often considered as a sibling to NADH. It plays a critical role in the electron transport chain, where it contributes directly to ATP production. FAD has a strong affinity for electrons, a feature that enables it to be a powerful contributor to energy generation.
Why FAD is Chosen: This coenzyme is lauded for its efficiency in generating ATP, particularly in aerobic respiration systems.
A notable characteristic of FAD is its ability to enter the mitochondrial electron transport chain at a different point than NADH, thus yielding different amounts of ATP. As with many cofactors, maintaining a balance is vital; too little can stall energy production, while too much can lead to oxidative stress.
Coenzyme A
Coenzyme A, or CoA, is a key player in fatty acid metabolism and the synthesis of acetyl-CoA, a critical metabolic intermediate. One of its significant features is its ability to carry acyl groups, which are necessary for various enzymatic reactions.
Importance of Coenzyme A: It is a ubiquitous cofactor and is particularly beneficial for its versatility, participating in crucial metabolic pathways that generate energy from fats and sugars.
What sets Coenzyme A apart is its role in linking different biochemical pathways, making it an essential hub in metabolism. However, disruptions in CoA levels can have negative ramifications for energy production and metabolic health.
Ultimately, understanding the distinct roles and characteristics of these ATP cofactors provides crucial insight into how energy processes are managed within living organisms. Together, they ensure that ATP is not just a molecule of energy but a facilitator of life's complex biochemical dance.
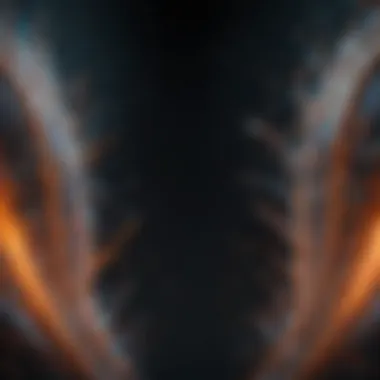
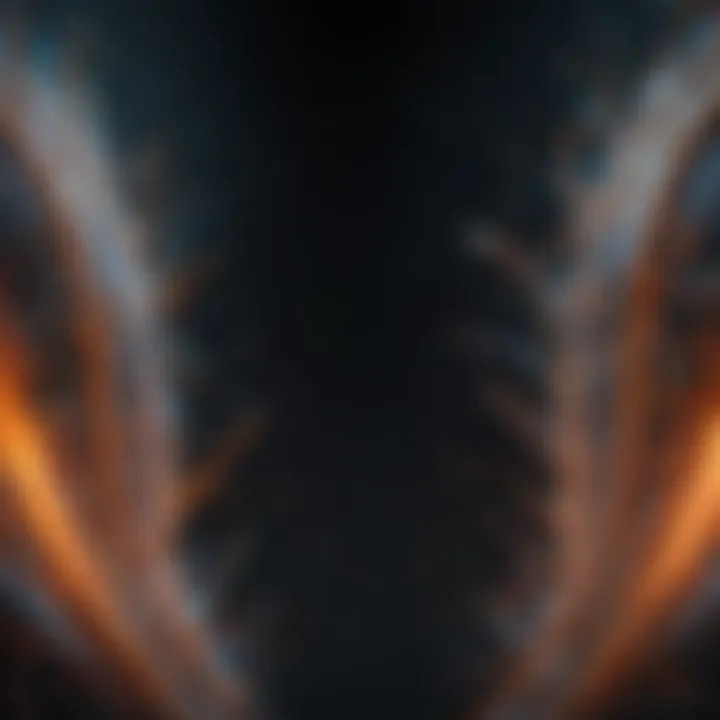
Mechanisms of Action of ATP Cofactors
Understanding how ATP cofactors function is crucial as they play a key role in cellular dynamics. Their mechanisms of action not only facilitate energy transfer but also enhance enzyme activity, thereby impacting myriad biochemical processes within living organisms. Recognizing these mechanisms is essential in dissecting metabolic pathways and improving our grasp of cellular health.
Cofactors in Energy Transfer
Cofactors are integral players when it comes to energy transfer, especially in scenarios where ATP undergoes turnover in metabolic cycles. Notably, magnesium ions, a prevalent cofactor in these processes, often sit at the heart of ATP’s structure, stabilizing the molecule and ensuring its readiness for hydrolysis. This reaction releases energy, which cells harness for various functions.
In conjunction with ATP, cofactors also participate in transferring energy to other molecules, acting somewhat like intermediaries. Think of them as helpful aides in a relay race: they pass energy efficiently from one runner—molecule—to the next, ensuring that no energy is wasted. This always keeps cellular processes running smoothly, enhancing productivity at every turn.
- Functionality in Transport: ATP cofactors are paramount in the transport of various molecules across membranes. They generate the necessary gradient that allows nutrients and ions to flow in and out, echoing their versatility and importance in cellular function.
- Participation in Metabolic Pathways: In metabolic processes such as glycolysis and the citric acid cycle, cofactors aid in the transfer of phosphoryl groups. This capability to attach and detach groups contributes to the continuous flow of energy through biosynthetic pathways.
Thus, the intricate dance of ATP and its cofactors in energy transfer reflects their supportive yet essential role, ensuring life sustains itself at the most fundamental level.
Influence on Enzyme Activity
ATP cofactors make life easier for our enzymes, allowing them to do their thing more efficiently. Enzymes, those unsung heroes of biochemistry, drastically speed up reactions, but they can't always do it alone. This is where cofactors step in, providing that extra push (or sometimes, a little nudge) to aid enzyme performance.
Many enzymes require cofactors to be fully functional. For example, zinc is a cofactor for numerous enzymes, playing a significant role in tasks ranging from DNA synthesis to protein folding. Without such cofactors, enzymes may struggle with binding substrates effectively, leading to decreased reaction rates or complete standstills.
- Cooperative Binding: The relationship between ATP cofactors and enzymes can often be characterized as cooperative, where the presence of one enhances the function of another. Such binding not only optimizes the reaction environment but may help maintain structural integrity.
- Post-translational Modifications: Certain cofactors are involved in post-translational modifications of enzymes, which may affect their stability, activity, and localization. This adds another layer of complexity, as modifications can deliver crucial signals to the cells, triggering or inhibiting specific pathways.
Biological Importance of ATP Cofactors
ATP cofactors play an essential role in various biological processes. Their importance cannot be overstated. They assist in numerous biochemical reactions that provide energy for cellular activities. To grasp their significance, it's crucial to delve into two main areas: metabolism and signal transduction. When we comprehend the biological role of these cofactors, we see their impact on health, disease, and overall cellular vitality.
Cofactors in Metabolism
Adenosine triphosphate (ATP) is like the fuel that keeps a car running, but its efficiency is greatly boosted by cofactors. These small molecules can be thought of as helpful companions that assist enzymes, enhancing metabolic pathways. For instance, a cofactor like NADH plays a critical part in cellular respiration, where it aids in the conversion of nutrients into usable energy.
- Energy Production: Cofactors enable the biochemical reactions necessary for the breakdown of glucose, fats, or proteins into energy. Without them, energy production would be inefficient.
- Process Facilitation: In processes such as glycolysis and the citric acid cycle, cofactors ensure reactions proceed smoothly. They help transition reactants into products efficiently, minimizing energy loss.
- Fine-Tuning Reactions: Certain cofactors can influence the speed of reactions. By doing so, they help ensure that cells respond adequately to changing conditions for maintaining homeostasis.
In summary, cofactors are pivotal in metabolic processes. They ensure that energy production is both efficient and effective, allowing cells to perform their functions optimally.
Role in Signal Transduction
Signal transduction is akin to a chain reaction, where one event triggers another, ultimately leading to a specific cellular response. ATP cofactors significantly influence this intricate communication system.
- Molecular Signaling: ATP itself acts as a signaling molecule in many pathways. It is called upon when a cell needs to communicate with others or respond to external stimuli. Cofactors work along with ATP to modulate these signals, making responses more precise.
- Activation of Pathways: Many signaling pathways, such as those involving protein kinases, need cofactors to be activated. These cofactors can help facilitate the phosphorylation of proteins, turning on various cellular functions related to growth and repair.
- Regulation of Cellular Responses: The balance of cofactors can determine how effectively a cell responds to signals. For instance, if cofactors are deficient or in excess, it can lead to aberrant signaling, causing health issues.
Sources of ATP Cofactors
Understanding the sources of ATP cofactors is fundamental for appreciating how our cells sustain energy needs and perform myriad functions. These cofactors are not merely additives; they are the unsung heroes in the grand choreography of metabolism and energy transfer. The implications of their sources stretch from the dinner plate to complex biochemical pathways, making them vital for maintaining cellular health and overall physiological processes.
Dietary Sources
When it comes to fueling our bodies with the necessary cofactors, diet plays a starring role. Dietary sources can be broken down into two main categories: those rich in essential minerals and those providing organic cofactors. Here’s a closer look at what you might find on your plate:
- Fruits and Vegetables: Foods like spinach and bananas are packed with magnesium, an important ATP cofactor. Dark leafy greens, notably, are also excellent sources of various trace minerals.
- Nuts and Seeds: Almonds and pumpkin seeds offer hefty portions of zinc and magnesium, two minerals crucial for the function of ATP-related enzymes.
- Meat and Fish: Animal proteins are loaded with B-vitamins and Coenzyme A, indispensable for forming key metabolic pathways.
- Legumes: Beans not only deliver proteins but also supply folate and other B-vitamins that aid in energy metabolism.
The bioavailability of these vitamins and minerals varies, so it's essential to consume a varied diet to capture these crucial cofactors effectively. Individual deficiencies could be subtle at first, manifesting as fatigue or decreased performance, but over time, they can lead to more significant health issues.
Synthetic Pathways
In addition to dietary intake, our bodies also draw upon complex synthetic pathways to produce ATP cofactors. These processes underline the body’s remarkable ability to generate necessary compounds from simpler precursors. Here are some examples of notable synthetic pathways:
- De Novo Synthesis: The body can synthesize certain cofactors from basic building blocks like amino acids and nucleotides. For instance, NADH is derived from the amino acid tryptophan, underlining the importance of protein sources in the diet.
- Metabolic Interconversions: Through biochemical processes, cells can convert one cofactor into another based on metabolic needs. For example, the interconversion between NADH and NAD+ allows cells to regulate energy-efficiently depending on their state—whether they're in a resting state or undergoing intense activity.
- Endogenous Production: Certain groups of cofactors, like Coenzyme A, can be synthesized from precursors found in various metabolic pathways. The availability of the nutrients for these pathways often relies on prior dietary intake, intertwining the need for adequate nutrition with biochemical health.
The synthesis of ATP cofactors is critical, not just for energy transfer but also for regulating broader metabolic functions across diverse tissues.
Understanding these sources not only informs dietary choices but also highlights the biochemical finesse behind our cellular operations. This intricate system of sourcing, be it from food or through internal processes, underscores the complex yet beautiful interplay that sustains life at a molecular level.
ATP Cofactors in Health and Disease
The intricate relationship between ATP cofactors and health is like an essential thread woven into the fabric of biological life. Their presence—or absence—can make all the difference when it comes to bodily function. Enhancing our understanding of these cofactors unveils insights into disease mechanisms and opens avenues for targeted therapies. In this section, we explore deficiencies, their implications, and the overarching impact on cellular functions, which is vital for anyone keen on delving deeper into biological systems.
Deficiencies and Their Implications
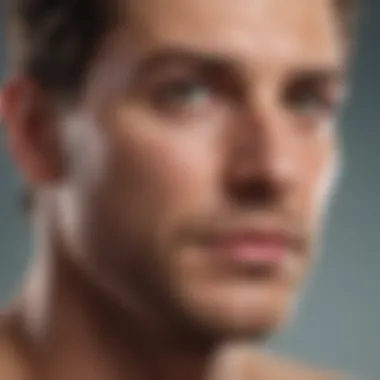
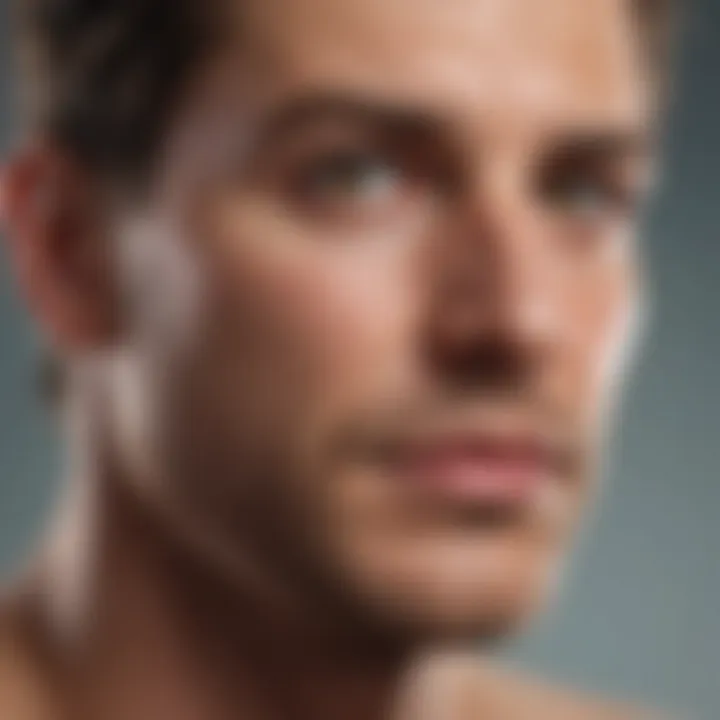
Deficiencies in ATP cofactors can lead to a cascade of biochemical mishaps in the body. Imagine a car running on inadequate fuel; it just won't run efficiently. The same goes for cells when cofactors are deficient. Here are a few key implications:
- Metabolic Disorders: Without sufficient cofactors, enzymatic reactions slow down. This slowdown can manifest as metabolic syndrome, diabetes, or even heart disease. The role of cofactors like NADH is crucial in energy metabolism; their deficiency is often tied to reduced ATP production.
- Neurological Issues: The brain is particularly sensitive to fluctuations in ATP levels. Cofactors like Coenzyme A and certain B vitamins are vital for brain function. A deficiency can cause cognitive decline or increase the risk of neurodegenerative diseases like Alzheimer's.
- Muscle Weakness: Inadequate magnesium levels impair muscle function, leading to weakness or cramping, particularly evident in athletes or physically active individuals. Without magnesium’s support in ATP synthesis, muscles can feel like they’ve hit a wall.
As you can see, these deficiencies are not just minor inconveniences; they pose significant health risks.
Impact on Cellular Functions
ATP cofactors play a dynamic role in maintaining cellular homeostasis. When functioning optimally, these cofactors keep the wheels of cellular processes turning smoothly. Here’s how deficiencies or imbalances can affect cellular operations:
- Energy Generation: ATP is the energy currency, but cofactors like magnesium facilitate its production within mitochondria. If magnesium levels drop, ATP generation falters, undermining energy-resilient functions.
- Signal Transduction: Cofactors influence signal transduction pathways vital for cellular communication. For instance, when cofactors like NAD+ drop, it disrupts signaling and can lead to cellular miscommunication. This miscommunication can escalate into chronic inflammatory conditions or other diseases.
- Regeneration and Repair: Certain cofactors are critical in DNA and RNA synthesis, impacting cell proliferation and repair mechanisms. A decline in these cofactors can lead to impaired healing processes or increased mutation rates, contributing to cancer developments.
"Cofactors might be small players, but their impact on cellular functions is undeniably huge. Take care of your cofactors, and they will take care of you."
Understanding how ATP cofactors intersect with health and disease opens doors to nutritional strategies and potential therapeutic implications. Awareness of deficiencies and their cellular impact serves as a cornerstone for preventative health measures as well.
Research Advances in ATP Cofactor Studies
Research in ATP cofactors has made significant strides, reshaping our understanding of their roles in biological systems. These advances have broad implications, affecting various scientific fields such as biochemistry, pharmacology, and even nutrition. By investigating how cofactors interact with ATP, researchers have gained insight into both fundamental biological processes and potential therapeutic applications.
Innovations in Biochemical Research
The innovation landscape is continuously evolving, and ATP cofactors are at the forefront of this change. Breakthroughs in analytical techniques, such as mass spectrometry and nuclear magnetic resonance (NMR), have made it possible to study these cofactors in unprecedented detail. For instance, scientists now can monitor real-time interactions between ATP and its cofactors under physiological conditions, enhancing our understanding of enzymatic mechanisms.
These innovations allow researchers to uncover how various cofactors influence the rate of ATP hydrolysis and subsequent energy release. To put it simply, it’s like watching a well-choreographed dance where each step (or reaction) is critical to the final performance. This information can be beneficial in fields such as drug design, where understanding these interactions can lead to the development of more effective therapies.
"Understanding the intricacies of ATP cofactor interactions at a molecular level can illuminate pathways that were once considered inscrutable, paving the way for new advancements in medical science."
Emerging Therapeutic Applications
The therapeutic implications of research into ATP cofactors are immense. For example, metabolic disorders often stem from dysfunctions in ATP production or cofactor availability. By targeting these cofactors, scientists are developing strategies for corrective measures in conditions like diabetes or mitochondrial diseases. In recent studies, certain cofactors have shown promise as adjuncts in traditional therapies, enhancing overall treatment efficacy.
Moreover, there’s a burgeoning field focused on the development of novel cofactor analogs that could serve as potent drugs. These analogs can mimic natural cofactors, offering a way to modulate cellular processes without interfering with regular metabolism. As a result, this line of research is not only broadening the scope of therapeutic possibilities but also influencing preventive health strategies.
Future Directions in ATP Cofactor Research
The exploration and understanding of ATP cofactors has come a long way, but there is still vast territory to cover. Going forward, researchers aim to uncover not just new cofactors, but also more nuanced mechanisms of their action. This journey is not merely academic; it paves the path for potential breakthroughs in health, medicine, and biochemistry. Here are several specific elements and considerations crucial for future research in ATP cofactors:
- Innovative Cofactor Identification: Finding novel cofactors that can partner with ATP in various biological processes stands as a primary goal. These could enhance ATP's effectiveness or introduce completely new functionalities. For instance, the discovery of previously unknown small molecules could offer new avenues for energy synthesis and regulation in living cells.
- Understanding Interactions: Another vital area is depicting how cofactors interact with ATP and other cellular molecules. It’s not always as straightforward as just binding; the dynamics at play can be quite intricate. Enhanced techniques in spectroscopy and imaging might shed light on these interactions, facilitating a more complete picture of cellular energy transactions.
- Implications for Disease: The role of cofactors in diseases, particularly those linked with mitochondrial dysfunction or metabolic syndromes, needs more investigation. By understanding how cofactors influence ATP’s role in disease progression, researchers might identify targets for therapeutic interventions, or even dietary recommendations to counteract deficiencies.
"Understanding the role of ATP cofactors in human health can illuminate paths to innovative treatments for complex diseases."
- Synthetic Biology: With the advances in synthetic biology, designing engineered pathways that exploit ATP cofactors could revolutionize bioengineering processes. This might include the creation of more efficient biofuels or enhanced metabolic pathways for pharmaceutical production.
Exploration of Novel Cofactors
The bold endeavor of searching for new cofactors means not just identifying them, but also exploring their functional capabilities within biological systems. This could encompass:
- Natural Sources: Scanning natural products for potential cofactors unlocks a treasure trove of options. Organisms that thrive in extreme environments often possess unique adaptations, and their biochemistry might hold rare cofactors that can teach us about ATP utilization.
- Synthetic Options: Beyond naturally occurring molecules, the synthetic scaffolding of new cofactors presents distinctive opportunities. By crafting molecules in the lab, researchers can tailor their properties and possibly enhance their compatibility with ATP in novel ways.
- Variability in Functions: Understanding that cofactors can have varied roles depending on the context is crucial. Some might serve as simple enzymes, while others can act as signaling molecules or structural components in cellular machinery.
Integrating Systems Biology Approaches
An evolving approach in researching ATP cofactors involves integrating systems biology. This multidisciplinary method considers the complexity of biological systems rather than isolated functions. Here are some implications:
- Holistic Understanding: Systems biology enables scientists to visualize the interplay among various molecules, including ATP and its cofactors. By utilizing computational models, one can simulate cellular environments, paving the way for predictions about how cofactors interact under different conditions.
- Network Analysis: Future studies could employ network analysis to delineate pathways in which ATP cofactors participate. This can reveal a broader picture of metabolic and signaling networks, leading to discoveries about how these cofactors can influence whole-system behavior.
- Multi-Omics Technologies: The convergence of genomics, proteomics, and metabolomics will enhance the understanding of how ATP cofactors operate within the broader cellular context. Advanced techniques could allow researchers to measure how changes in one area affect others, leading to insight that might not arise from traditional investigations.
In essence, the future of ATP cofactor research is ripe with possibility. Bridging gaps between basic science and real-world applications holds the key to unlocking their full potential, benefiting human health and advancing our understanding of life at the molecular level.
The End: The Intricacies of ATP Cofactors
In summarizing the multifaceted realm of ATP cofactors, it becomes clear that these biological entities are not merely supporting players in cellular processes. Instead, they are fundamental to the intricate dance of life at the molecular level. Their impact extends across various dimensions of biological functions, threadimg through metabolic pathways, energy transfer mechanisms, and even cellular signaling. Understanding these cofactors has far-reaching implications, not only for comprehension of fundamental biochemistry but also for practical applications in health sciences and disease management.
Summary of Key Points
- ATP's Central Role: Adenosine triphosphate is widely recognized as the primary energy currency of cells. It's a critical molecule in bioenergetics that fuels various cellular functions, including muscle contraction, biosynthesis, and cell division.
- Diversity of Cofactors: ATP cofactors are diverse, including metal ions like magnesium and organic coenzymes like NADH and Coenzyme A. Each type plays a distinct but overlapping role in facilitating biochemical reactions.
- Mechanisms of Action: These cofactors participate actively in reactions by stabilizing enzyme structures or contributing directly to the energy transfer process. They can determine the efficiency and specificity of enzyme activity, influencing overall metabolic efficiency.
- Health Implications: Consideration of ATP cofactors is crucial as deficiencies can lead to significant health issues, from metabolic disorders to impaired cellular signaling. This highlights the necessity of ensuring appropriate dietary intake of essential cofactors.
- Research Advances: Recent studies in biochemistry have unveiled new insights into how cofactors function, paving the way for therapeutic applications. Understanding ATP cofactor dynamics could inform the development of drugs targeting metabolic diseases or cellular dysfunctions.
Significance for Future Research
Moving forward, research on ATP cofactors continues to hold promise.
- Novel Cofactors: Investigating lesser-known cofactors and their roles can illuminate previously obscure pathways in metabolism. Such exploration could uncover new strategies for enhancing cellular function or treating diseases.
- Systems Biology Approaches: Integrating systems biology could provide a holistic view of how ATP cofactors interact within the cellular environment. Synergistic relationships among molecules, enzymes, and signal transduction pathways present intriguing possibilities for comprehensive understanding.
- Translational Applications: The transition from bench to bedside in medical research often necessitates a deeper understanding of basic principles. As we learn more about ATP cofactors’ roles in cellular energetics, novel therapeutic pathways might emerge, especially in areas like cancer, where energy metabolism is often disrupted.
In reflection, acknowledging the intricacies of ATP cofactors allows for a fuller understanding of biochemistry and fuels the engine of scientific inquiry. As the research progresses, it’s essential to highlight their significance not only in fundamental science but also in understanding health and disease, shaping the future landscape of biological and medical research.