Agarose Gel Recipe: Essential Guide for Research
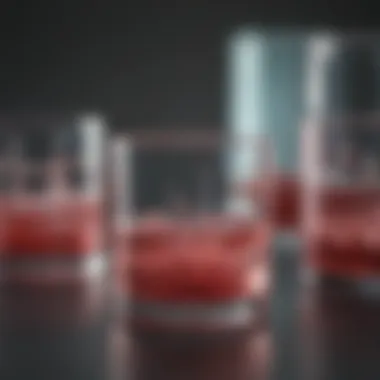
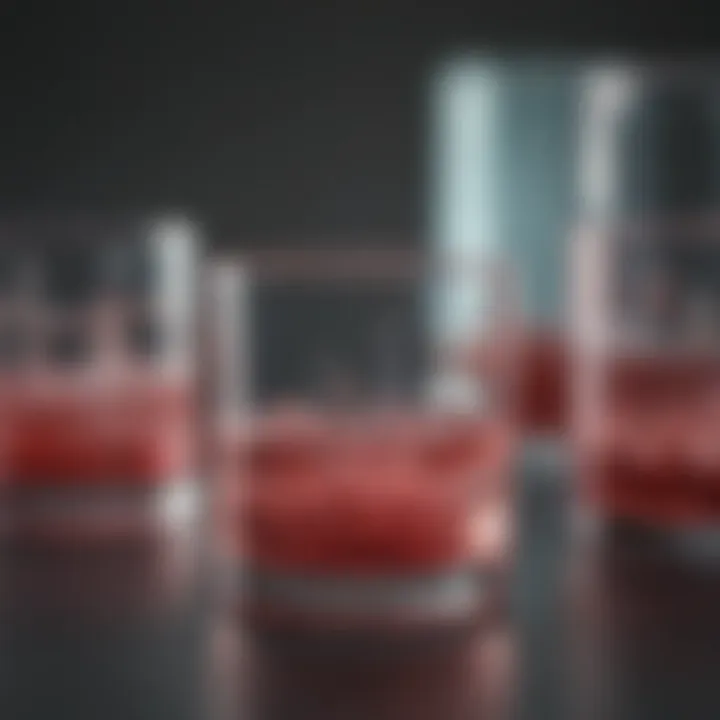
Intro
Agarose gel electrophoresis is a staple technique in molecular biology and biochemistry, allowing researchers to separate and analyze nucleic acids and proteins effectively. The process hinges on creating a gel matrix made from agarose, which plays a pivotal role in how well it performs during electrophoresis. Understanding this methodology not only enhances a researcher’s toolkit but also defines the accuracy and efficiency of related experiments.
By diving into the elements of agarose gel preparation, this guide serves as a resource for students, educators, and professionals alike. It emphasizes the importance of precise measurements, suitable additives, and optimal gel concentrations, which can make or break an experiment. Moreover, the exploration of variations in gel properties—due to differing concentrations or additives—unpacks a deeper knowledge of this essential technique.
Solid groundwork in agarose gel preparation opens doors to various applications, from genetic mapping to forensic analysis. As we work through this guide, you’ll find detailed insights into both standard practices and innovative approaches, ensuring that you are well-equipped to tackle any research challenges related to gel electrophoresis.
Prolusion to Agarose Gels
Agarose gels are a cornerstone in the toolkit of molecular biologists. They serve as a medium for separating biomolecules, primarily nucleic acids. Understanding the intricacies of agarose gels is vital for any researcher involved in genetic analysis, forensic science, or biochemical studies. Their ability to create a porous matrix allows different sizes of DNA or RNA to migrate at distinct rates under an electric field. This phenomenon is crucial when one aims to purify, quantify, or visualize nucleic acids in various applications.
The significance of agarose gels extends beyond mere separation. They provide clarity in the interpretation of results and enable researchers to make informed determinations about molecular weight and purity of samples. Additionally, agarose gels can be tailored by varying concentration levels, buffering systems, and even additives, thus expanding their versatility. Factors like these contribute to successful gel electrophoresis outcomes, yielding more reliable and reproducible results — a necessity in all scientific disciplines.
"Agarose gels are not merely a tool; they underpin many breakthroughs in genetic research and diagnostics."
What is Agarose?
Agarose is a polysaccharide derived from red algae, specifically from the gelidium spp. When isolated, it forms a gel when dissolved in hot water and subsequently cooled. Unlike some other gelling agents, agarose presents a low background fluorescence, making it highly advantageous for applications where visualization of nucleic acids is essential. The variable concentration of agarose allows researchers to fine-tune the gel's pore size, affecting the speed at which different-sized fragments navigate through the matrix.
Notably, agarose is favored over acrylamide for many applications due to its non-toxic nature and ease of preparation. When selecting agarose, it's paramount to consider its melting temperature, gel strength, and clarity to ensure optimal results. The choice of agarose type can significantly influence the outcome of gel electrophoresis, thus impacting the integrity of the research.
Applications in Molecular Biology
The applications of agarose gels in molecular biology are vast and varied. They are primarily used for:
- DNA Fragment Separation: Whether analysing PCR products or restriction enzyme digests, agarose gels enable precise separation based on size.
- Gel Electrophoresis for RNA: Essential for studying gene expression and analyzing transcripts, agarose gels facilitate effective RNA quantification.
- Cloning Verification: After inserting genes into vectors, agarose gels are employed to confirm successful ligation and insertion.
- Genotyping: Agarose gels can help to analyzing DNA fingerprinting and SNP genotyping, playing a key role in forensics and genetic research.
- Quality Control: Researchers depend on agarose gels to assess the quality and integrity of nucleic acids prior to further analysis.
The wide array of applications showcases agarose gels as more than just a utility; they are strategic instruments that have transformed how researchers conduct genetic and biochemical investigations. Thus, grasping the foundational knowledge about agarose and its multiple functions is indispensable for scientists engaged in today’s biotech landscape.
Ingredients of Agarose Gel Recipe
Understanding the ingredients that go into agarose gel preparation is fundamental for any researcher. Each component plays a pivotal role in determining not just the quality of the gel but also its effectiveness in various applications. Having the right mix ensures that the end product will meet the specific needs of molecular biology experiments, especially when it comes to gel electrophoresis.
Agarose Powder
Agarose powder is the core ingredient in the making of agarose gels. It’s a polysaccharide extracted from seaweed, primarily composed of agar, and is soluble in boiling water. When cooled, it forms a gel matrix, allowing for the separation of particles like DNA or RNA based on size.
The choice of agarose concentration is also key; a low concentration (around 0.7% to 1.0%) yields a flexible gel suited for larger fragments, while higher concentrations (up to 3%) are better for small fragments. Essentially, agarose powder's versatility is what makes it indispensable in laboratories.
Buffer Solutions
Proper buffer solutions are crucial in maintaining the pH and ionic strength during electrophoresis. They help to conduct the electric current through the gel, ensuring that the nucleic acids migrate correctly.
TAE Buffer
TAE Buffer (Tris-Acetate-EDTA) is one of the most commonly used buffers in agarose gel electrophoresis. The unique trait of TAE is its ability to support the stability and integrity of DNA during separation. It's particularly advantageous for ligation and restriction enzyme digestion protocols. However, one must consider that prolonged electrophoresis in TAE can lead to the degradation of DNA because it lacks the buffering capacity of TBE.
Key Characteristics of TAE Buffer:
- Acts as an effective buffer for DNA
- Easy to prepare and use in various molecular biology applications
That said, its degradation potential requires careful time management during running conditions, or else you might end up with unwanted results.
TBE Buffer
TBE Buffer (Tris-Borate-EDTA) stands out due to its excellent buffering capacity and stability over extended electrophoresis runs. This makes it a favored choice for separating smaller fragments of DNA. Its ability to maintain pH during longer procedures not only ensures clarity but also improves the resolution of the bands.
Despite its advantages, TBE is often considered a bit more complex to prepare compared to TAE. Another important point is that TBE can interfere with downstream applications like restriction digests. Thus, it is crucial to select this buffer carefully based on the requirements of your particular experiment.
Key Characteristics of TBE Buffer:
- Superior buffering capacity for longer runs
- Better resolution for smaller fragments
Staining Dyes
To visualize the DNA after the gel electrophoresis process, staining dyes are employed. These allow researchers to see the DNA bands under UV light, a critical step in analyzing results.
Ethidium Bromide
Ethidium Bromide has been a staple dye for visualizing nucleic acids for many years. This intercalating agent binds to DNA and fluoresces under UV light, making it easy to see bands on the gel. It’s popular largely because of its efficiency and strong signal. However, caution is necessary, as it is a known mutagen, necessitating safety precautions when handling.
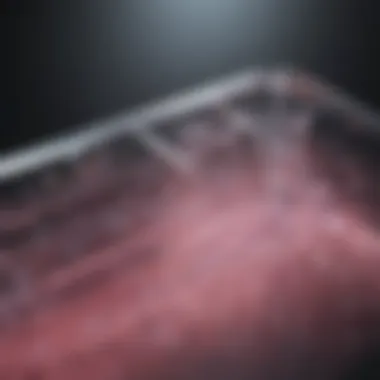
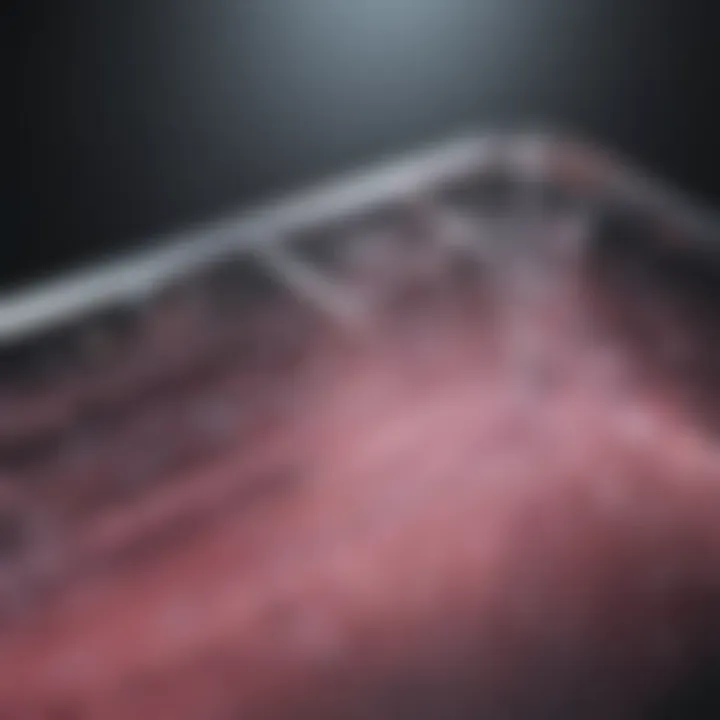
Advantages of Ethidium Bromide:
- High sensitivity in detecting DNA
- Established method with a vast supporting literature
Unfortunately, its mutagenic properties limit its use in some labs that prioritize safety and may prompt researchers to look for alternatives.
SYBR Safe
An alternative to Ethidium Bromide is SYBR Safe. This dye offers a safer approach to visualizing DNA. With similar effectiveness, SYBR Safe provides fluorescence under UV light, but with less mutagenic risk. It has rapidly gained traction due to its user-friendly nature and cost-effectiveness.
Advantages of SYBR Safe:
- Non-mutagenic, making it safer for the lab environment
- Strong signal for robust visualization
Despite its advantages, there are considerations regarding its potential for interference in some downstream applications, making it essential to evaluate your specific needs before selecting a dye.
In summary, understanding the function and importance of each ingredient in the agarose gel preparation process is vital for executing successful experiments. Making informed choices about agarose powder, buffers, and staining dyes can greatly influence the quality of results, thus, ensuring effective execution of molecular biology research.
Preparing the Agarose Gel
Preparing the agarose gel is a critical step in any gel electrophoresis experiment. This is where the magic starts, as the gel serves as the medium through which the biomolecules, like DNA or RNA, will migrate under an electric field. The gel’s composition and the method of preparation can significantly affect the results of your experiment. Therefore, understanding each element of this process is indispensable for achieving reliable, reproducible results.
Deciding the Gel Concentration
The concentration of agarose in your gel is largely determined by the size of the molecules you expect to separate.
Low Concentration Gels
Low concentration gels typically range from 0.5% to 1% agarose. These gels provide a more porous structure, facilitating the migration of larger DNA fragments.
- Key Characteristic: The larger pores allow for the easier movement of bulky DNA.
- Benefit: This concentration is particularly beneficial for separating whole plasmid DNA or large PCR products, where you want good resolution without the gel becoming too dense.
- Unique Feature: They provide a delicate balance; although the resolution for smaller fragments isn't as refined, it increases the feasibility of working with larger strands.
- Disadvantages: However, low concentration gels can sometimes lead to less precise band resolution if smaller fragments are present, as they may move too quickly through the gel.
High Concentration Gels
High concentration gels typically range from 1.5% to 2% agarose. These gels are more compact and create tighter pores that hinder the movement of large DNA fragments a bit more than their lower concentration counterparts.
- Key Characteristic: They shine when it comes to the separation of smaller DNA fragments, usually below 500 base pairs.
- Benefit: If high resolution for small fragments is your goal, this is the route to take. High concentration gels also help in achieving more distinct and better-separated bands, making them a popular choice for genetic fingerprinting or similar analyses.
- Unique Feature: However, while they excel at small fragment separation, they can make separating larger fragments difficult, essentially creating a bottleneck.
- Disadvantages: That said, the increased density can lead to a greater risk of gel rupturing or becoming overly difficult to load samples into wells.
Mixing Ingredients
Mixing the agarose powder with the buffer solutions is another key component of preparing the gel. Here, it’s essential to achieve the right consistency and homogeneity. Ensure that the powder is evenly distributed in the buffer to avoid any localized concentrations that could impact gel performance. This would be done by stirring gently as to not create bubbles, which could compromise the integrity of the gel.
Melting Agarose
To melt the agarose powder, it's usually dissolved by heating the mixture, typically in a microwave or a heat block. This step is crucial because the heat breaks down the agarose granules, allowing for a smooth, uniform gel mixture. Be careful to avoid overheating, as it can create unwanted bubbles or degrade the agarose itself.
Cooling and Setting the Gel
Once the agarose is melted and mixed well, it must cool down to the point where it can be safely cast without damaging the wells. Pouring the gel into trays before it fully cools is key, as it locks in the structure. Typically, it’s poured at about 50-60°C, allowing for solidification. As the gel sets, the pores form in the resultant matrix, which is crucial for the subsequent separation process.
The ability to prepare agarose gel effectively hinges on these foundational aspects. A well-prepared gel is instrumental for reliable electrophoresis outcomes.
Casting the Agarose Gel
Casting agarose gel serves as a pivotal step in the gel preparation process. It’s where the gel is set for subsequent electrophoresis, a technique that paves the way for analyzing nucleic acids, such as DNA and RNA. Done properly, casting ensures that the gel has a uniform structure, which plays an important role in the migration of samples during electrophoresis.
The importance of this phase cannot be overstated. A well-cast gel allows for reproducible results and accurate visualizations. Conversely, a poorly cast gel can lead to uneven migration patterns, resulting in smeared or distorted bands. This could mislead interpretations, making it critical for researchers and students alike to grasp the nuances of this task.
Using Gel Casting Trays
When it comes to choosing gel casting trays, several factors should be taken into account. Trays come in various sizes, and selecting the right one depends on the volume of the gel and the number of samples to be loaded. Plastic trays are common due to their affordability and availability. However, glass trays might offer advantages in clarity, making it easier to visualize the gel while working.
- Ensure the tray is clean and free from any residual agarose from previous uses. Cleaning with distilled water and drying can prevent contamination.
- Consider using trays with adjustable sidewalls that allow for varying gel thickness, thereby making them versatile for different applications.
Once the correct tray is selected, make sure it’s assembled properly with tools like combs set for well formations. Using clamps or tape along the edges can help prevent leakage of the agarose.
Formation of Wells
The formation of wells is crucial for loading samples into the gel. Proper well formation allows for an organized loading of reagents and minimizes contamination.
To form wells, a comb is inserted into the gel before it solidifies. Here's how to do it:
- Pour the molten agarose into the tray, ensuring a uniform distribution.
- Immediately place the comb into the gel with a firm, steady hand, pressing it down gently but not too hard to break the gel.
- Leave the comb in place for about 20-30 minutes or until the gel solidifies completely.
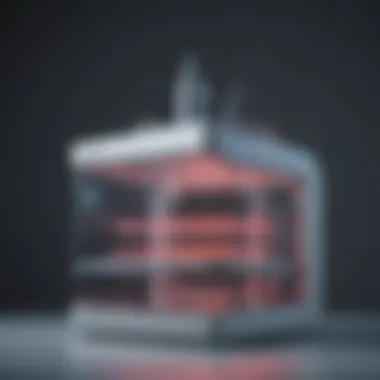
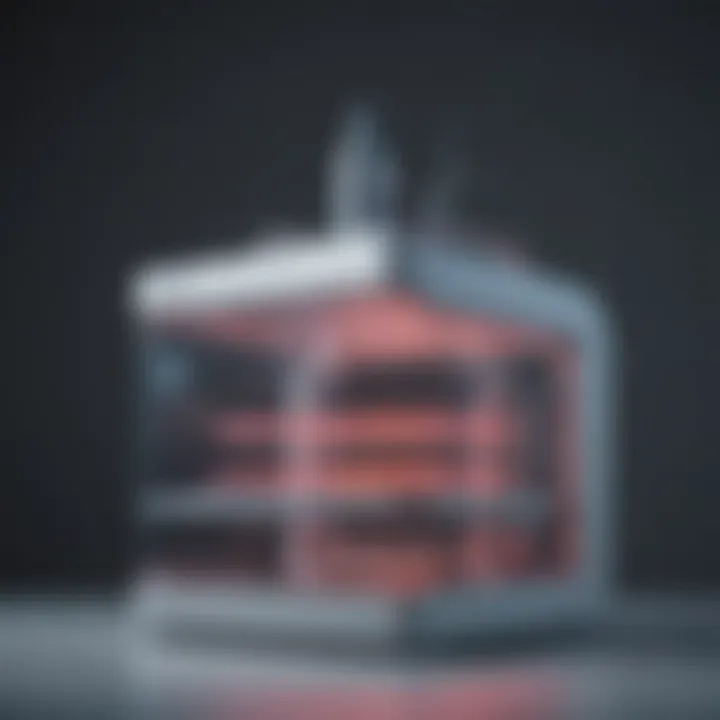
Once the gel has set, remove the comb carefully to avoid damaging the wells. It helps to wiggle the comb gently before lifting it out. A clean well formation is essential; jagged or uneven wells can lead to mixed or smeared results during analysis.
A properly cast agarose gel with clean wells is key for reliable experiments, offering clarity and precision in genetic analysis.
In summary, mastering the casting process—including selecting appropriate trays and establishing wells—is foundational to achieving accurate results in gel electrophoresis. Taking these steps seriously not only enhances the quality of the gel but also elevates the overall research outcomes for students and professionals alike.
Running Gel Electrophoresis
Running gel electrophoresis serves as the crux of studying molecular fragments, particularly DNA and RNA, allowing scientists to separate nucleic acids based on size, a pivotal process in fields spanning genetics to forensic science. It offers insights into genetic variation, aids in cloning procedures, and even helps in the analysis of expression patterns. This section unfolds essential components and methods, ensuring clarity as you embark on this intricate yet fascinating journey in agarose gel research.
Setting Up the Equipment
Preparation is everything when it comes to running gel electrophoresis. Setting up the equipment is like laying the foundation of a sturdy house; without it, your work could come crashing down. The core components include the electrophoresis apparatus, a power supply, and safety features such as gloves and eye protection.
- Electrophoresis Apparatus: Choose a gel box suitable for your gel dimensions. Ensure that electrodes are correctly positioned to avoid any mishaps. They should be clean, dry, and free from any contaminants.
- Power Supply: This equipment should be reliable and can provide a consistent voltage. It’s often helpful to use one that allows you to fine-tune the current according to the gel concentration and desired separation.
- Safety Gear: Always prioritize your safety—nobody wants a mishap while mixing chemicals or loading gels. Wear gloves, lab coats, and goggles at all times.
Understanding the setup procedures is vital because even minor mistakes can lead to poor results or hazardous situations.
Loading Samples
Once your equipment is set up, it's time to load samples into the wells you've formed in the agarose gel. This step requires precision and a delicate touch akin to threading a needle to avoid disasters. Here are key points to consider:
- Sample Preparation: Mix your DNA or RNA with a loading dye. The dye serves two purposes: it adds density, helping the sample sink into the well, and it allows you to track the progress of electrophoresis through color changes.
- Pipetting Technique: Use a micropipette to load samples slowly and steadily into the wells. Avoid puncturing the agarose or overloading wells, which could result in sample diffusion and compromised clarity.
- Stay Organized: Sequentially number your samples to prevent mix-ups. This organizational habit will save you headaches later, especially when interpreting your results.
Electrophoresis Protocol
Executing the electrophoresis procedure isn't just a walk in the park; it's methodical and necessitates attention to detail at every step. Follow these guidelines for successful electrophoresis:
- Configure the Power Supply: Set the voltage according to the manufacturer's guidelines, typically ranging from 80 to 150 volts, depending on gel size and thickness.
- Connect Electrodes: Double-check that your electrodes are correctly connected. The positive electrode should always lead to the opposite side of the gel, ensuring migration towards it.
- Monitor the Run: Keep an eye on the samples as they run. This involves observing the movement of the loading dye, which should give you a rough idea of when to stop the run. Aim for the dye to travel about two-thirds the length of the gel.
- Documenting Results: Once the run is complete, switch off the power supply. Start documenting where the bands are located against a ladder or marker you’ve included in the gel. This will guide you later during analysis.
By understanding the significance of each step in running gel electrophoresis, you are well prepared to embrace the world of molecular analysis with confidence.
"Good preparation is half the battle won."
Post-Electrophoresis Analysis
Understanding the analysis following electrophoresis is vital in molecular biology research. Proper interpretation of results can provide insights into the effectiveness of the experimental design and the quality of the samples used. This phase allows researchers to assess whether the desired DNA fragments were successfully separated and visualized, which is crucial for the reproducibility and validity of experiments.
The post-electrophoresis analysis serves multiple purposes. First, it enables the visualization of DNA bands, indicating the presence and concentration of the DNA fragments separated during the gel running process. This is crucial in determining if the concentration of agarose was appropriate and whether the samples were loaded correctly. Secondly, it aids in understanding potential issues that might have arisen during the electrophoresis process, helping researchers diagnose problems and refine their techniques for future experiments.
Visualizing DNA Bands
Visualizing DNA bands is a fundamental step in analyzing the outcomes of gel electrophoresis. This process often employs fluorescent stains like Ethidium Bromide or SYBR Safe to enhance the visibility of DNA under ultraviolet light. When UV light is applied, the bands can be seen, allowing researchers to gauge their size and integrity. However, care must be taken due to the carcinogenic nature of some filtration agents; always practice appropriate lab safety measures when handling these substances.
The visibility of bands can be influenced by several factors:
- Gel Concentration: Higher concentrations lead to better separation of smaller fragments, while lower concentrations are more accommodating for larger DNA fragments.
- Electrophoresis Time: Running the gel for too long can cause bands to run too far, potentially merging with others or diffusing.
- Staining Time: Over-staining or under-staining can also affect visualization. It’s crucial to optimize this based on the experiment’s requirements.
The clarity of bands is essential when assessing the quality of the samples analyzed and whether they meet the expected distribution of fragment sizes. A successful visualization allows for the effective documentation and troubleshooting of the overall electrophoretic process.
Interpreting Results
Once the DNA bands are visualized, the next step is interpreting the results. This means analyzing the size of the bands in comparison to a molecular weight standard or ladder, which acts as a point of reference. Identifying the sizes of DNA fragments enables researchers to verify if the expected bands corresponding to specific regions of interest are present in the samples.
Several critical considerations come into play during this interpretation:
- Comparison with Standards: Aligning results with a ladder helps determine fragment sizes accurately.
- Band Intensity: The intensity of the bands provides insight into the relative concentration of DNA, which can be pivotal in quantifying nucleic acids present in the samples.
- Presence of Unexpected Bands: Observing unexpected bands or smearing can indicate issues such as degradation of DNA or non-specific binding. Recognizing these anomalies is essential for understanding the quality of reagents and the overall experimental design.
"Analyzing agarose gel results is akin to piecing together a puzzle, where each band and its size tell a story about the molecular interactions at play."
The analysis does not end at merely observing the bands. It necessitates a comprehensive understanding of how experimental conditions affect the outcome. Keeping detailed notes throughout the process can help in refining techniques and identifying areas for improvement in future experiments.
Troubleshooting Common Issues
When you’re involved in agarose gel electrophoresis, troubleshooting common issues becomes a critical skill. It’s essential because errors can lead to invalid results, wasted time, and resources. By understanding potential problems and their solutions, you not only save yourself some headaches but also deepen your comprehension of the electrophoresis process itself.
Issues with Gel Quality
The quality of your agarose gel can significantly impact the outcome of your experiments. Factors like the agarose concentration, the mixing of the gel solution, and even the cooling phase play into this. For instance, if your gel has air bubbles or a heterogeneous texture, it might not allow the DNA migration to happen efficiently. Here are a few pointers to ensure your gel quality:
- Make sure to dissolve the agarose completely before pouring it.
- Check for air bubbles during the cooling. Gently tapping the mold can help.
- Inspect for any debris or particulates that might create uneven migration paths.
The significance of these elements cannot be overstated. A gel that's well-prepared can lead to sharp, distinct bands while a poorly made gel might yield smear or no bands at all. So if you ever find yourself staring at a gel without visible results, it might just be a problem with quality at the preparation stage.
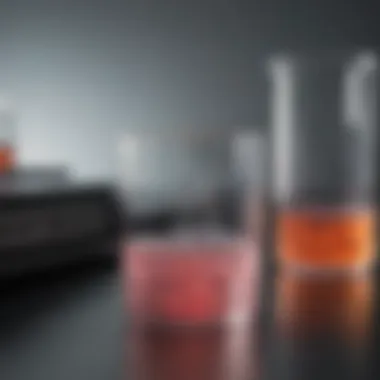
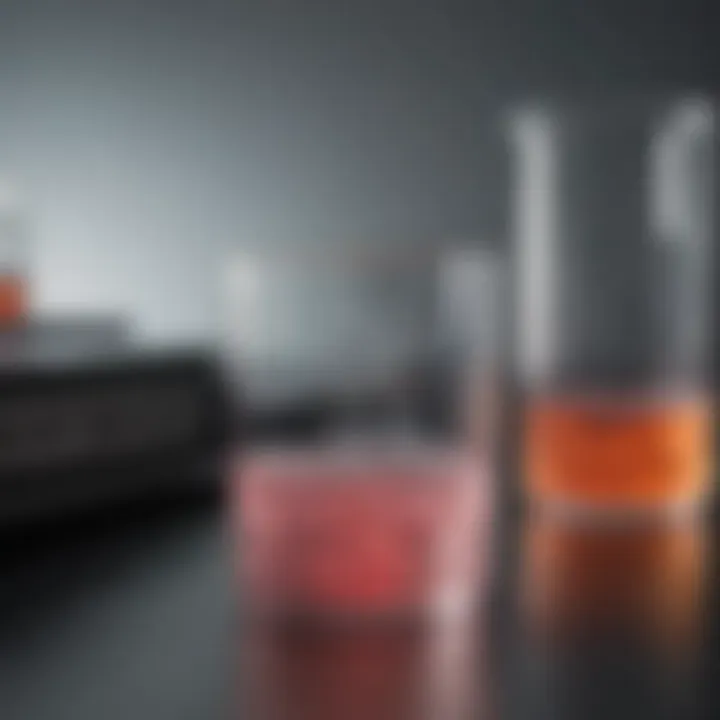
Migration Problems
Migration problems occur when DNA fragments do not move through the gel properly. This can stem from various factors, including voltage settings, gel thickness, or even the composition of your buffer. For example, running your gel at too high a voltage can cause overheating, leading to distorted bands or bands that run off the gel entirely. Conversely, if the voltage is too low, the migration speed might be so slow that the bands do not resolve adequately.
Here’s a checklist for troubleshooting migration issues:
- Always use the recommended voltage settings for your buffer system. For instance, TAE and TBE have different optimal voltages.
- Ensure uniformity in the gel thickness; a thicker gel means slower migration.
- Double-check buffer concentrations; dilution can affect the conductivity and thus the migration.
Understanding how to tackle these migration problems is key for successful gel electrophoresis. The nuances of how your samples behave can reveal a lot about their composition and integrity—so keeping a keen eye on these factors can turn a perplexing situation into a skillfully resolved experiment.
Variations in Agarose Gel Recipes
Agarose gel recipes are not one-size-fits-all; they vary widely based on specific research needs. This flexibility is crucial in molecular biology, where subtle differences can lead to vastly different results. Whether it's optimizing the resolution of DNA fragments or adapting to specific staining protocols, understanding the variations can largely improve the outcomes of your experiments.
By customizing gel formulations, researchers can fine-tune properties like pore size, clarity, and even band separation. Some might need a gel that accommodates larger DNA bands, while others aim for sharp resolution of smaller fragments. Knowing these variations equips scientists with tools to address differing experimental challenges effectively.
Incorporating Additives
Adding components to agarose gels can greatly influence their performance. Additives such as gelatin and carrageenan offer unique properties that can enhance your results. Each has features that cater to specific research requirements, providing alternatives to classic agarose setups.
Gelatin for Enhanced Resolution
Gelatin is a natural polymer with a unique ability to improve the clarity and resolution of gels. When incorporated, it can help in separating closely sized DNA fragments—crucial during DNA analysis. One positive trait of gelatin is its biodegradability, making it a favorable choice for environmentally conscious researchers. Additionally, this ingredient can soften the gel, allowing for easier handling in certain applications.
However, the inclusion of gelatin isn’t without its drawbacks. It may introduce variability; different batches can alter the gel's firmness and, thus, outcomes. This can add a layer of complexity when striving for repeatable experiments.
"When striving for precision, even the smallest changes can ripple into your results, spare no detail in gel prep."
Carrageenan for Different Matrix Properties
Carrageenan, mainly derived from red seaweed, is another additive gaining traction in the field. It serves to create gels with distinct rheological properties, enabling scientists to manipulate the texture and strength of the gel matrix. Its ability to form gels at room temperature can enhance workflow efficiency, especially in multi-step procedures.
Carrageenan's unique feature lies in its capacity to provide both firm and soft gels, depending on the concentration used. Researchers can target various analyte sizes more effectively with this flexibility. Nonetheless, using carrageenan can come with a cost; its processing might require careful calibration to avoid unwanted intermolecular interactions that could influence migration patterns.
Using Alternative Gelling Agents
Alternatives to agarose are available for specific situations. Some researchers explore different gelling agents to accommodate unique experimental setups or to tackle particular challenges in separation techniques.
Agar Instead of Agarose
Agar, a close relative to agarose, is often seen as a viable substitute for certain applications. It tends to form more rigid gels, which can be advantageous in experiments requiring minimal deformation upon loading samples. The simplicity of agar preparation can make it attractive, especially in resource-limited environments.
Yet, agar's rigidity might limit its use in scenarios requiring flexibility, particularly in delicate experiments that involve longer electrophoresis runs. Thus, while it posits certain benefits, it might not serve as an all-encompassing solution for everyone.
Polyacrylamide Gels
A further alternative is polyacrylamide gels, which are particularly suited for high-resolution applications, such as protein separation. The synthetic nature of polyacrylamide allows for control over pore size to a greater extent than agarose, catering to significantly different experimental needs.
One noteworthy aspect of polyacrylamide gels is their compatibility with various sample types, enhancing their versatility. Nevertheless, they can be somewhat more labor-intensive to prepare and come with potential hazards due to the acrylamide, which has neurotoxic properties. As such, careful handling is essential during preparation.
By understanding these variations and alternatives, researchers equip themselves with the ability to optimize their methodologies for specific outcomes, opening doors for advancements in scientific discovery.
Finale and Implications
Drawing the curtain on agarose gel preparation, it's essential to appreciate the undeniable impact this technique has on the field of molecular biology and biochemistry. Not just a method, but a reliable friend for researchers aiming to delve into the world of nucleic acids. Agarose gels provide a window into the structural and functional aspects of DNA and RNA. Whether you're purifying fragments, checking the quality of your samples, or even analyzing the genetic material, the importance of a well-prepared agarose gel cannot be overstated.
The benefits are numerous:
- Reliability of results, essential for drawing scientific conclusions.
- Flexibility in applications, accommodating various molecular weights and sample types.
- Accessibility, as agarose gel preparation is comparatively straightforward and cost-effective.
However, it's also vital to consider the limitations inherent in this method. For instance, while agarose gels are superb for nucleic acids, they may not be the best choice for protein analysis, where alternatives like polyacrylamide gels would serve better. Understanding these boundaries ensures proper application without redundancies.
"Agarose gels represent a blend of art and science – it's not just about technique, but also about understanding molecular interactions."
In summary, agarose gel techniques are indispensable to the toolkit of modern scientific researchers. As we move forward in molecular biology, the implications of comprehending and refining agarose gel preparation can lead to breakthroughs in genetic research, diagnostics, and therapeutics. The adaptability of these gels in various research directions remains a cornerstone of innovation in life sciences.
Summary of Key Points
- Agarose gels offer a fundamental platform for visualizing nucleic acids, making them essential for genetic analysis.
- Versatility allows for various applications, from electrophoresis to gel purification.
- Critical technique empowers researchers with reliable results, although its limitations must be acknowledged when applied in different contexts.
- Future innovations in gel electrophoresis hold the potential to expand and refine the applications of agarose gels even further.
Future Directions in Gel Electrophoresis Research
As we look toward the horizon of gel electrophoresis research, there’s a palpable excitement regarding the future capabilities for agarose gels. Scientists are continuously exploring novel additives and hybrid gel formulations that could enhance resolution and specificity. Innovations such as fully automated electrophoresis systems could revolutionize workflow efficiency.
Moreover, advancements in gel composition can enable better separation of complex mixtures. Customizing gels tailored for specific applications is a tantalizing prospect, opening doors for investigative techniques previously constrained by traditional gel recipes. With increasing emphasis on reproducibility and accuracy in research, the journey of refining agarose gel methodologies appears full of promise.
In this evolving landscape, collaboration between researchers and technologists is paramount. The merging of computational approaches with experimental techniques could yield new insights into agarose gel behavior under various conditions, enhancing our understanding of electrophoretic dynamics.
Overall, the road ahead for agarose gel research is rife with opportunities, with ongoing innovations likely to shape the future of how we analyze and interpret nucleic acid behavior in countless applications.